1.
Introduction
The heterogeneous photocatalysis process has been found to be very promising, for instance the generation of green fuel (H2) by splitting of water, sterilization of medical instruments by the bactericidal effect, purification of water by decomposition of dyes and other waste products of industries etc. In this photocatalysis process, photoexcited carriers in semiconductor nanostructures facilitate the breaking of dye molecules into more diminutive innocuous molecules through the redox reaction. The nanostructures of semiconductors like ZnO and TiO2 have already been popular for this photocatalysis process[1–5]. As the band gaps of ZnO and TiO2 are more than 3 eV, so these materials are independently found to be very useful for UV photocatalysis application. In order to harvest the synergetic, structural, and electronic properties of both ZnO and TiO2, many efforts have been made to prepare zinc orthotitanate (Zn2TiO4), the compound semiconductor of these two materials. Due to band gap engineering, this material and its composite are found to demonstrate great photocatalytic action with visible light[6–19]. However, no methodical contemplation has been done on the artificial visible light photocatalytic dye decomposition with Zn2TiO4 nanoparticles. Particularly, no effort has been made on finding the reaction kinetics and reaction rate constant of the artificial visible photocatalytic dye decomposition activity of Zn2TiO4 nanoparticles grown by the solid reaction method. This is one of the simplest methods to grow Zn2TiO4 nanoparticles, thus in this work, we have investigated their artificial visible light photocatalytic dye decomposition behavior in detail.
2.
Experimental details
2.1
Material preparation and characterization
The nanoparticles of Zn2TiO4 were grown by a solid state reaction route with powder precursors of ZnO (Product No. 28975, Fisher Scientific, purity: 98.99%) and TiO2 (product No. 28375, Fisher Scientific, purity: 98.99%) taken in a molar ratio of 2 : 1[20]. Both measured powder precursors were ground for 3 h in an acetone medium followed by calcination at 950 °C for 2 h. The X-ray diffraction (XRD) analysis, field emission scanning electron microscopy (FESEM), transmission electron microscopy (TEM), diffuse reflectance spectroscopy (DRS), photoluminescence (PL) study, and Brunauer–Emmett–Teller (BET) study were carried out to characterize the Zn2TiO4 nanoparticles.
From the XRD analysis, the peaks were identified at angle 2θ = 31.97°, 34.62°, 36.46°, 53.98°, and 56.81° correspond to (201), (211), (202), (204), and (105) crystal planes of Zn2TiO4 respectively (Fig. 1(a)). This result is found to be in good agreement with the result published by Nikam et al.[12] indicating that the dominant phase of the grown material is Zn2TiO4. Some minor peaks were also found at angle 2θ = 38°, 56°, and 48° originating from residual ZnO and TiO2. The angles at 2θ = 38°, 56° correspond to the (200), (211) planes of ZnO whereas the angle at 2θ = 48° corresponds to the (102) crystal plane of TiO2 respectively. From the analysis of FESEM, most of the nanoparticles were found within the range of 20–180 nm having a maximum number of particles of size 58 ± 18 nm (Fig. 1(b)). From the BET isotherm (Fig. 1(c)), the specific surface area of nanoparticles was estimated as 8.78 m2/g.

class="figure_img" id="Figure1"/>
Download
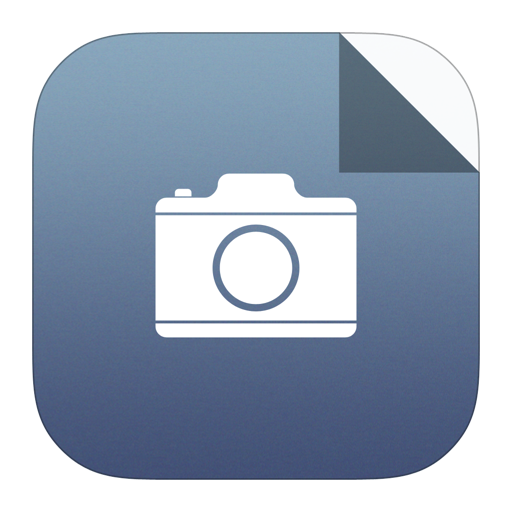
Larger image
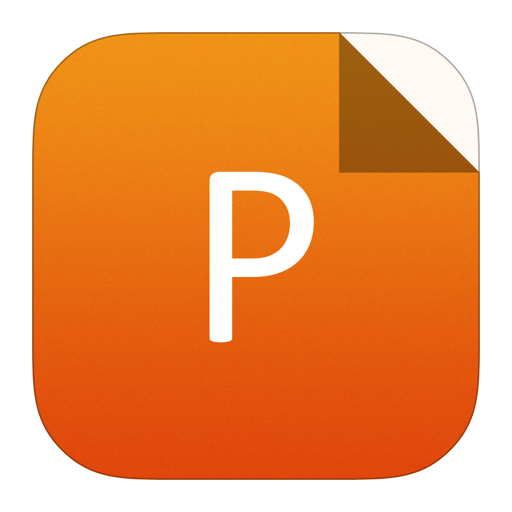
PowerPoint slide
Figure1.
(Color?online) (a) XRD pattern, (b) FESEM image, and (c) BET isotherm of the Zn2TiO4 nanoparticles.
The morphologies of Zn2TiO4 nanoparticles were also analysed using a JEOL-2010 transmission electron microscope (TEM) operated at an accelerating voltage of 200 kV. Fig. 2(a) shows the TEM image of Zn2TiO4 nanoparticles. The size of the particles has been observed to be in the range of 41–166 nm with their average size of 70 nm (Gaussian fit). This value is inconsistent with the value estimated from FESEM analysis and Scherrer’s equation[20]. Fig. 2(b) represents the high resolution TEM image of Zn2TiO4 nanoparticles. From this, the interplanar distance has been calculated as d = 0.255 nm corresponding to the (211) plane, which is in good agreement with the result published by Nikam et al.[12].

class="figure_img" id="Figure2"/>
Download
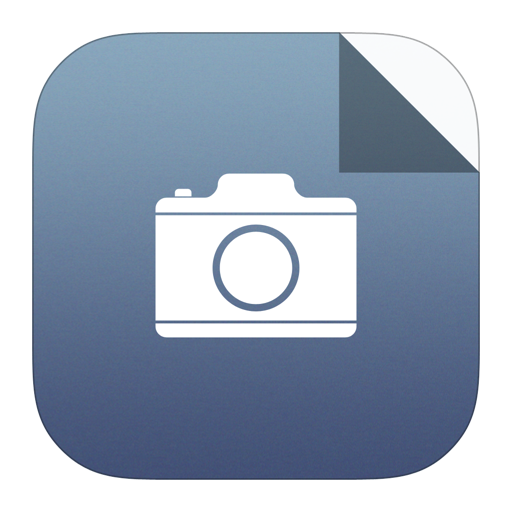
Larger image
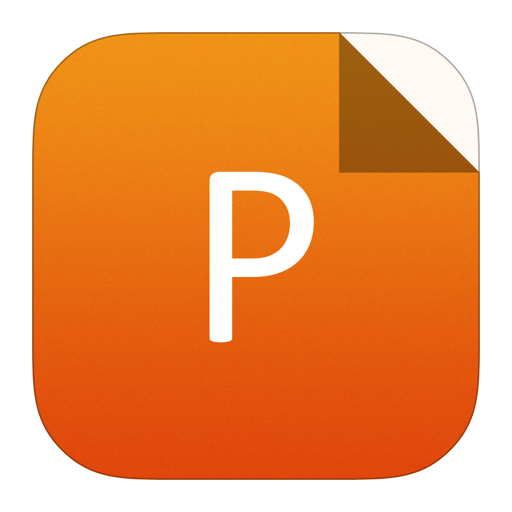
PowerPoint slide
Figure2.
(a) TEM image, and (b) HRTEM image showing lattice fringes with d211 = 0.255 nm of Zn2TiO4 nanoparticles.
The UV–visible DRS of the Zn2TiO4 nanoparticles were recorded by a UV–vis spectrophotometer (UV-2450, Shimadzu) with an attached integrating sphere. The absorbance data as obtained from this study is shown in Fig. 3.

class="figure_img" id="Figure3"/>
Download
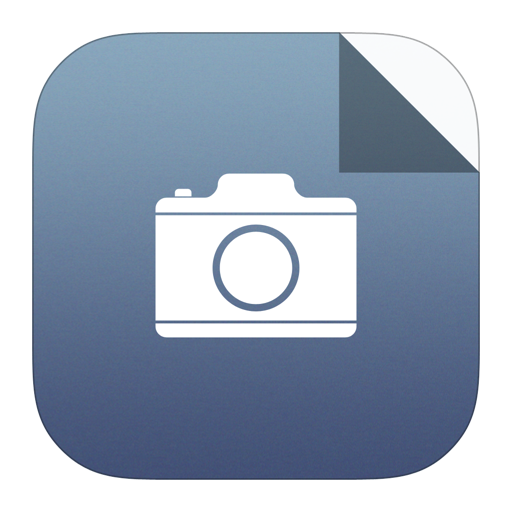
Larger image
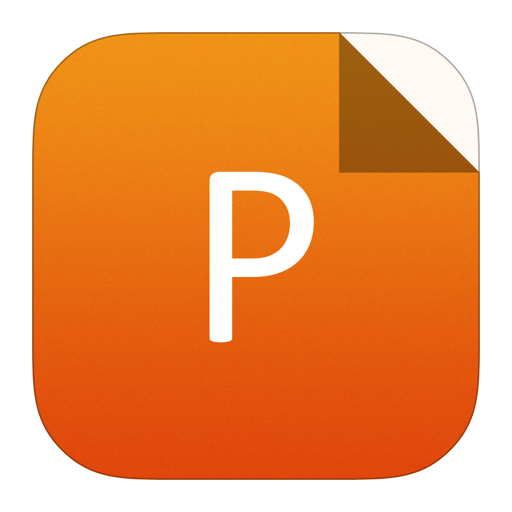
PowerPoint slide
Figure3.
(Color?online) UV–vis absorbance curve of Zn2TiO4 nanoparticles.
The band gap as obtained from this graph is 3 eV. Such large band gap material can show visible light photocatalytic activity only in the presence of defect levels inside the band gap[21]. From this DRS study we could not obtain any information on the defect level corresponding to the visible range of wavelength. Therefore, we did the PL study with the excitation wavelength of 325 nm. The result of this study is shown in Fig. 4 (solid line).

class="figure_img" id="Figure4"/>
Download
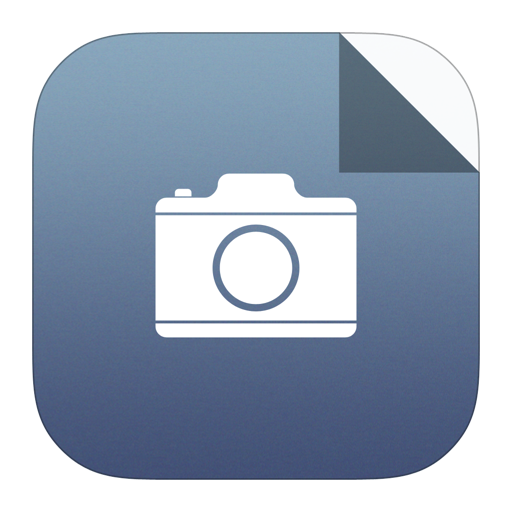
Larger image
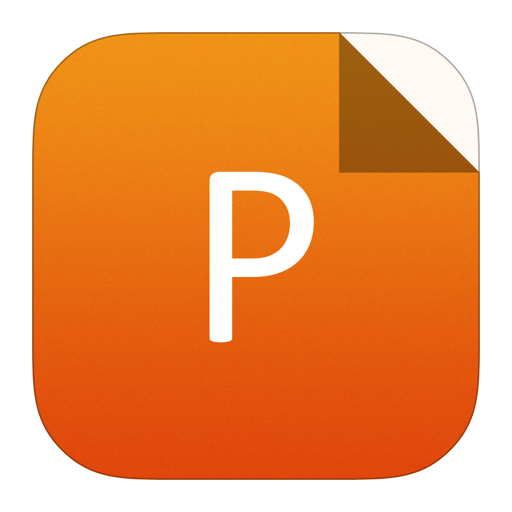
PowerPoint slide
Figure4.
(Color?online) PL spectra of Zn2TiO4 nanoparticles.
By multiple Gaussian fits (dashed lines) we found that this luminescence has 3 peaks. The UV peak at 385 nm (peak a) corresponds to the band-edge emission. This result is found to be well consistent with the result obtained from our DRS study. However, the other 2 peaks at 420 nm (peak b) and 470 nm (peak c) are in the visible range. These correspond to the various defect levels in the material[22]. The presence of such defect levels can promote the visible photocatalytic activity[21]. This may be the probable cause for the visible photocatalytic behaviour of our material.
2.2
Photocatalytic studies
The photocatalytic dye decomposition study with Zn2TiO4 nanoparticles was carried out in a photocatalytic reactor comprising of a visible lamp of 500 W and a magnetic stirrer. The spectrum of this lamp is shown in Fig. 5. This spectrum (450 to 860 nm) is found to cover almost the whole visible range.

class="figure_img" id="Figure5"/>
Download
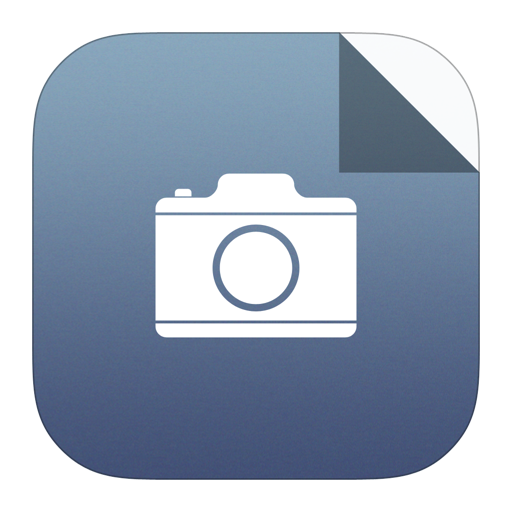
Larger image
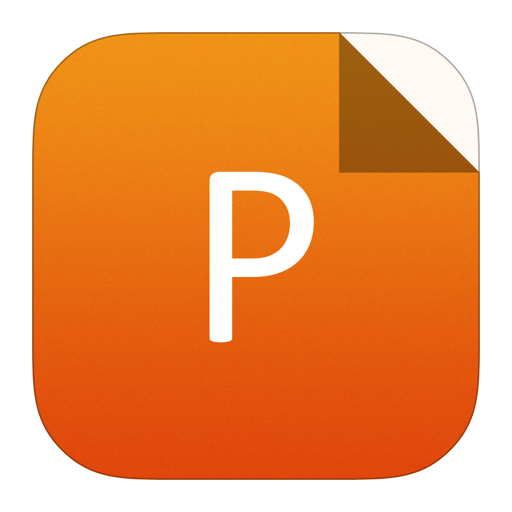
PowerPoint slide
Figure5.
(Color?online) Spectrum of the visible lamp used for the photocatalysis experiment.
Right off the bat, 50 mL of aqueous solution of methylene blue (MB) (2.5 × 10?5 molar conc.) and 0.02 g of nanoparticles were taken in a container of 50 mL capacity. The distance between the container and the light source was kept to be 25 cm. Before light illumination, the solution was kept for 30 min in dark conditions for complete adsorption–desorption of dyes on the catalyst surface. The entire photoreaction (both dark and light condition) was completed under persistent moderate mixing with a magnetic stirrer. The dye decomposition was measured through the absorption spectroscopy of the solution. For this in the wake of doing the photocatalytic movement for a specific interim of time, 3 mL of sample was taken to do the absorption spectroscopy to find the concentration of the dye. In order to find the reaction kinetics, a graph on ln (C0/C) versus visible irradiation time was plotted (where C0 is the initial concentration of MB and C is its concentration after a certain time of photocatalytic reaction). From the slope of the linear fit line of the graph, the reaction rate constant was estimated. The degradation efficiency was estimated by the formula [(C0 ? C)/C0 × 100].
2.3
In situ active species determination
To understand the mechanism of the photocatalysis reaction it is required to know the types of active species which are responsible for the degradation of organic dyes[23, 24]. So free radicals trapping experiments were carried out in the presence of scavengers like Isopropyl alcohol (IPA) and Ethylenediaminetetraacetate (EDTA). By following the procedure given by Liu et al.[25], the quantity of EDTA was taken to be 0.015 g and 0.013 mL of IPA per 50 mL of MB dye solution along with the Zn2TiO4 nanoparticles.
3.
Results and discussion
Fig. 6(a) displays the colour of dye solution after 0, 40 and 90 min time of artificial visible light exposure. Fig. 6(b) represents the UV–visible absorption spectra indicating the degradation of MB in the presence of visible-light irradiation for different durations.

class="figure_img" id="Figure6"/>
Download
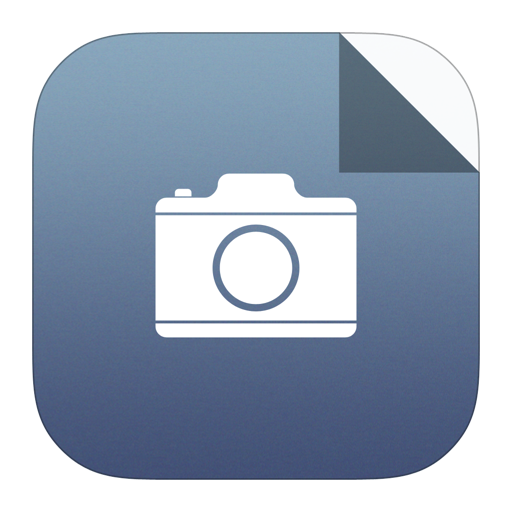
Larger image
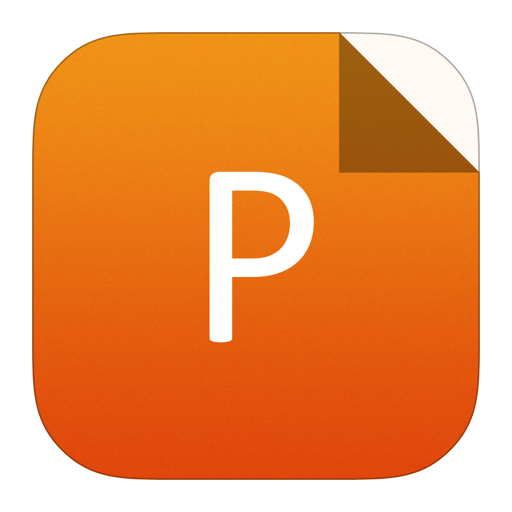
PowerPoint slide
Figure6.
(Color?online) Image of degradation of MB irradiated under visible-light for different durations in (a) the presence of Zn2TiO4 nanoparticles, and (b) UV–visible absorption spectra of MB dye solution.
Fig. 7(a) shows the photocatalytic degradation of MB (plot of C/C0 versus time) in the presence of visible-light with Zn2TiO4. In the absence of nanoparticles, no degradation of MB dye occurred under visible-light irradiation even after 30 min. The plot of ln(C0/C) versus irradiation time (Fig. 7(b)) shows a linear behaviour indicating the pseudo first order degradation kinetics. The rate constant of MB degradation was calculated from the slope of linear fit line of the plot of ln(C0/C) versus irradiation time as shown in Fig. 7(b). From this, the rate constant is found to be 0.069 min?1.

class="figure_img" id="Figure7"/>
Download
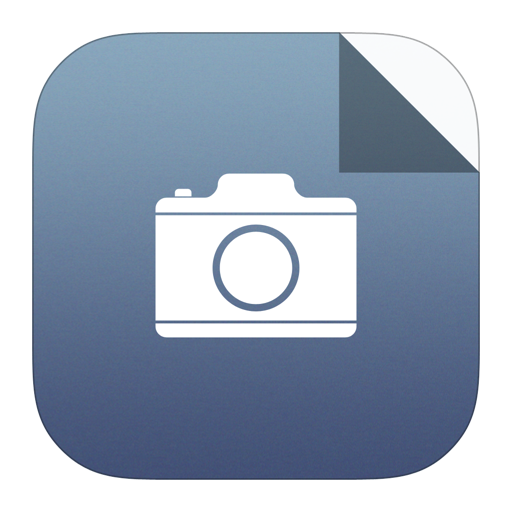
Larger image
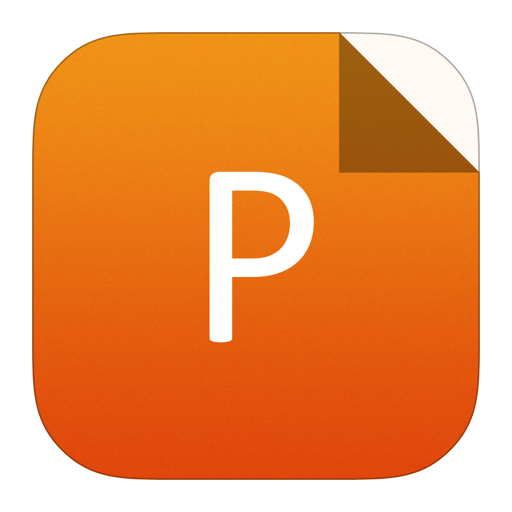
PowerPoint slide
Figure7.
(Color?online) (a) Photocatalytic decomposition of MB in the presence of visible-light with different catalysts, and (b) ln(C0/C) versus time graph. (scattered points: estimation from experimental data, solid line: linear fit)
The photocatalytic degradation of MB dye solution under visible light irradiation in the presence and absence of scavengers is shown in Fig. 8. For all the conditions the experiment was done for 40 min.

class="figure_img" id="Figure8"/>
Download
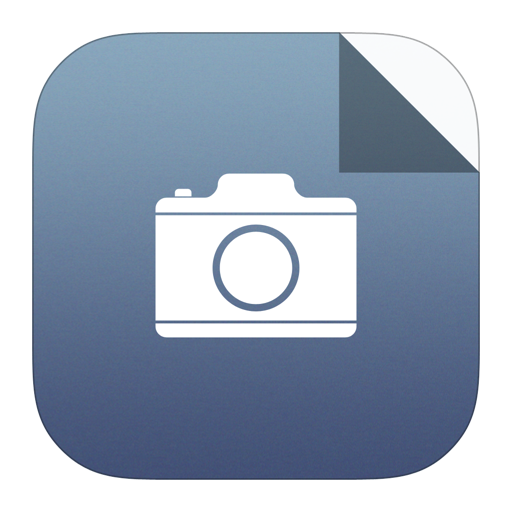
Larger image
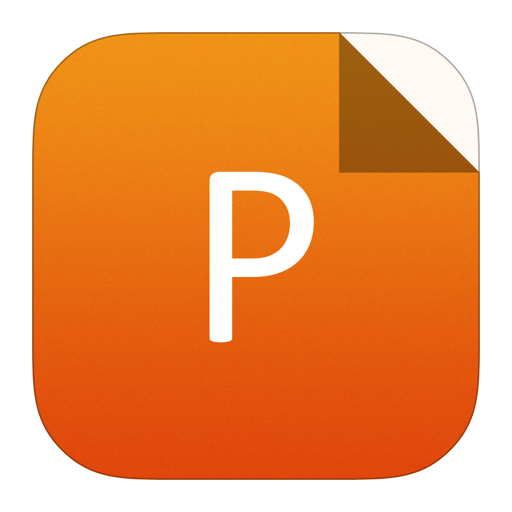
PowerPoint slide
Figure8.
(Color?online) MB dye degradation using Zn2TiO4 nanoparticles without scavenger and with scavengers in the presence and absence of visible light irradiation.
When EDTA was added into the solution, the photocatalytic degradation of MB dye was 40.4% whereas with the addition of IPA, the degradation was 10.2%. The degradation percentage of MB was 78.7% in the no scavenger condition under visible irradiation but 4.19% in dark conditions. Without Zn2TiO4 nanoparticles the MB dye degradation percentage under visible irradiation was only 5.24%. Generally IPA and EDTA can act as scavengers for hydroxyl radical species (·OH) and holes (h+) respectively[25]. As in the presence of these scavengers the photocatalytic dye degradation decreased drastically, it is clear that h+ and ·OH play a crucial role in the visible light photocatalytic degradation of MB dye.
Based on the aforementioned results, we confirm the proposed photocatalytic mechanism for the degradation of MB dye using Zn2TiO4 nanoparticles as the photocatalyst in our experiment as follows (Eq. (1) to Eq. (6))[9].
${ m Z}{{ m n}_2}{ m T_{ m i}}{ m O_4} + hgamma to { m e}_{ m CB}^ - + { m h}_{ m CB}^ + ,$ ![]() | (1) |
${ m Ti}{ m O_2}left( {{{ m e}^ - }} ight) + { m O_{ m 2ads}} to bullet { m O}_{ m 2}^ - + { m Ti}{ m O_{ m 2}},$ ![]() | (2) |
$ bullet { m O}_2^ - + { m H_2}{ m O} to { m H}{ m O_2} bullet +; { m O}{ m H^ - },$ ![]() | (3) |
${ m H}{ m O_2} bullet + { m H_2}{ m O} to { m H_2}{ m O_2} + { m OH} bullet ,$ ![]() | (4) |
${ m ZnO}({ m h^ + }) + { m H_2}{ m O_{ads}} to bullet { m OH} + { m H^ + } + { m Z_n}{ m O},$ ![]() | (5) |
${ m{OH}} bullet { m or};{ m H}{ m O_2} bullet + ;{ m MB} to { m C}{ m O_2} + { m H_2}{ m O}left( {{ m{Mineralized}};{ m{products}}} ight).$ ![]() | (6) |
The activation of Zn2TiO4 nanoparticles by visible light in our case produces electron-hole pairs which act as oxidizing and reducing agents, respectively (Eq. (1)). The defect levels that we mentioned in our PL study are mainly responsible for this visible light activation of Zn2TiO4 nanoparticles. Such an activation effect has already been reported in ZnO nanostructures containing oxygen vacancies[21]. The oxidative and reductive reactions that take place due to the generated electron–hole thereafter are expressed from Eq. (2) to Eq. (5) respectively. In the degradation of organic compounds (MB), the hydroxyl radical, which comes from the oxidation of adsorbed water or adsorbed OH?, is the primary oxidant; and the presence of oxygen can prevent the recombination of hole–electron pairs. For a complete reaction, the final products of the reaction among others are CO2 and H2O (Eq. (6)).
In order to compare the visible photocatalytic activity of the solid state grown Zn2TiO4 nanoparticles with respect to its individual constituents ZnO and TiO2 nanoparticles (all ground for the same amount of time) we did the experiment for the light exposure time of 40 minutes. The result of this study is shown in Fig. 9. From this figure it is clear that Zn2TiO4 nanoparticles can show a much more superior photocatalytic activity than its individual constituents. More efficient charge separation and increased lifetime of the charge carriers of Zn2TiO4 nanoparticles could be responsible factors for this[26–29].

class="figure_img" id="Figure9"/>
Download
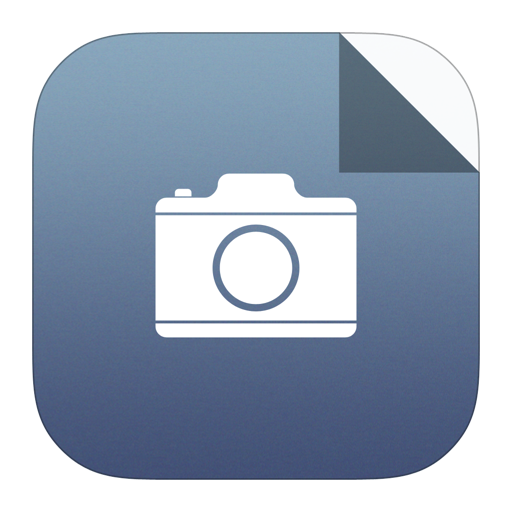
Larger image
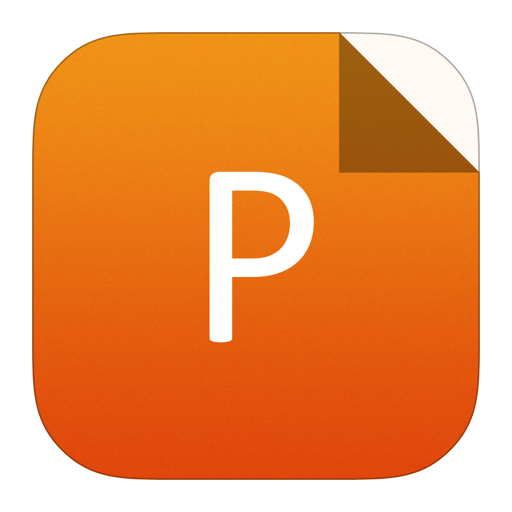
PowerPoint slide
Figure9.
(Color?online) MB dye degradation of Zn2TiO4 nanoparticles and its constituents (ZnO and TiO2) after 40 min of visible light irradiation.
Finally, it is to be noted that in our work, Zn2TiO4 nanoparticles were prepared from the laboratory grade ZnO and TiO2 powders of extremely low cost ($10 for 500 g). Because of this, these nanoparticles can be a better choice for applications of visible photocatalytic dye decomposition.
4.
Conclusion
In the present work, Zn2TiO4 nanoparticles of an average diameter of 58 nm and BET surface area of 8.78 m2/g were grown by the solid state reaction method and used for visible photocatalytic dye decomposition. The reaction kinetics was found to be the pseudo first order and the reaction rate constant (k) of Zn2TiO4 nanoparticles was found to be (k = 0.069 min?1). The possible mechanism of the observed visible photocatalytic dye decomposition activity is discussed by taking the result of PL and in situ active species determination studies. The cost of the material used is very low, so it can be found to be very useful for photocatalytic dye decomposition.
Acknowledgements
Institute of Physics (IOP), Bhubaneswar is acknowledged for providing the TEM facility for characterization of the nanoparticles.