1.
Introduction
Recently, III–V semiconductor nanowires (NW) have attracted great research interest due to their superior electrical and optoelectronic properties for future high performance nanoscaled devices, such as photodetector, solar cell, nanolaser, and light-emitting diode[1–7]. The future of all semiconductor NW technology will inherently rely on their doping capability. Both n- and p-type doping of NWs play important roles in most optoelectronic applications, since these applications are fabricated by the barriers such as pn junction[8]. Different from the growth of film structures, it is recognized that NW growth is initiated by a supersaturated liquid alloy through the vapor–liquid–solid (VLS) mechanism[9]. However, the question of doping mechanism still remains unsolved. It is not clear whether the dopants accumulate within the catalyst or they undergo diffusion mechanism from the sidewalls[10]. In addition, the typical NW growth conditions often do not favor dopant incorporation. There are only a very few publications describing the growth of p-type GaAs NWs[11, 12]. The widely used method of p-type doping in metal-organic chemical vapor deposition (MOCVD) is adding diethyl zinc (DEZn) to the gas phase during growth. It is reported that the doping concentrations are controlled from 4.6 × 1018 up to 2.3 × 1019 cm?3 by adjusting the II/III ratio[22]. In this paper, we report on p-type doping of GaAs nanowires realized by an additional DEZn flow during the growth. The I–V curves of NWs were measured and the doping concentration approximated to 1019–1020 cm?3.
2.
Experiment
The growth was carried out in a Thomas Swan close-coupled showerhead-metal-organic chemical vapor deposition (CCS-MOCVD) reactor at the pressure of 100 Torr. Before the growth, an Au film with a thickness of 4 nm was deposited on GaAs (111) B substrate by magnetron sputtering; the Au-coated GaAs substrate was placed in the reactor and annealed in situ at 645 °C in ambient arsine (AsH3) for 900 s to form the Au–Ga alloyed particles as catalyst. Trimethylgallium (TMGa) and AsH3 were used as precursors. The doping source was DEZn and hydrogen (H2) served as the carrier gas. GaAs NWs growth began after it was ramped down to the desired temperature. GaAs NWs were grown at 490 °C for 2000 s. The input II/III ratio was adjusted by varying the DEZn flow while keeping the TMGa flow constant at 14.4 sccm and the AsH3 at 100 sccm. After growth, the samples were cooled down in ambient H2. Five samples were grown in the experiment. The dopant to TMGa (II/III ratio) in the samples was varied in the range of 0.013 ≤ II/III ≤ 0.05.
As-grown nanowires were then transferred onto an intrinsic Si substrate that was covered with a 500 nm SiO2 layer by sonication with ethanol as carrier. Then electrodes were defined on both ends of a chosen NW by UV lithography and electromagnetic sputtering followed by a “lift-off” process. Before sputtering, the unmasked contact areas were etched for 5 s, and then passivated with (NH4)2S solution[13–15]. The morphological and structural characteristics of the samples were characterized by scanning electron microscopy (SEM) and the current–voltage (I–V) measurements were performed with an Agilent 2901A source meter. A 532 nm continuous-wave diode-pumped solid-state (DPSS) laser was used to excite the device.
3.
Results and discussion
Figs. 1(a)–1(e) show the cross-sectional SEM images of samples A–E, respectively. The DEZn flow during the growth of these samples increased from 5 sccm (A) to 20 sccm (E) as shown in Table 1. That is, the II/III ratio changed from 0.013 to 0.05. The average length of NWs for sample A, B, and C is 15.5, 16.1 and 20.0 μm, corresponding to the growth rate of 7.8, 8.1, and 10 nm/s, respectively. The increased growth rate with increasing the II/III ratio indicates that the enhanced flow of DEZn has a slight promoting effect on the growth rate of GaAs NWs. The reason is that DEZn, which predominantly decomposes heterogeneously, passivates the bare GaAs surface by a partial coverage of non-decomposed DEZn molecules and thereby increases the kinetic energy barrier for the growth of NW[16]. Besides, when DEZn flow is high (i.e. 15 sccm), a kink is observed on the top of NW which is marked by the red dotted circle in sample D. Kinking enhanced with the increased II/III ratio since the higher Zn concentration in the droplet destroyed the stabilization of the surface energy of the droplet. Moreover, the droplet split up under the II/III ratio of 0.05 (sample E), thus brand is observed on the top of NWs as shown in Fig. 1(e).

class="figure_img" id="Figure1"/>
Download
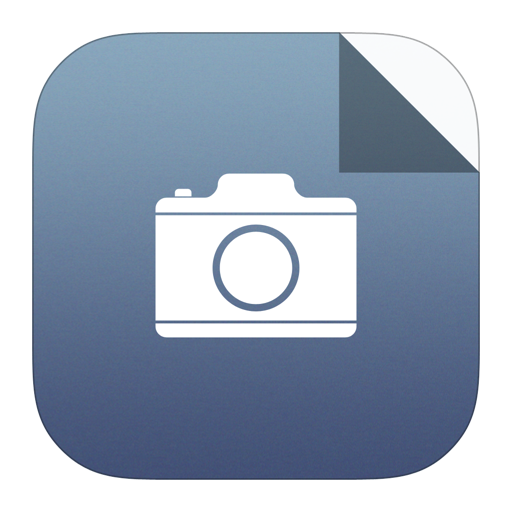
Larger image
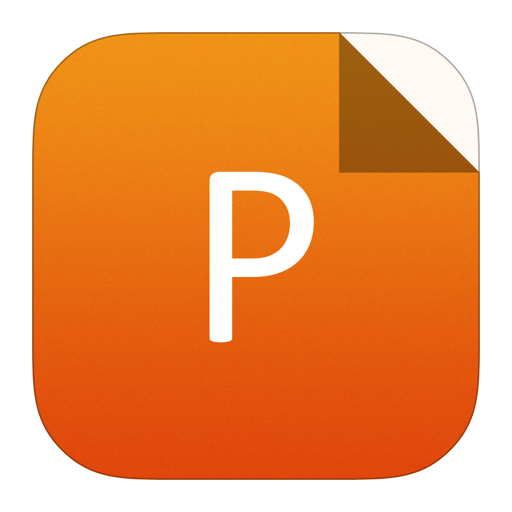
PowerPoint slide
Figure1.
The SEM image of all the samples. The growth parameters of each sample are shown in Table 1.
Sample | DEZn flow (sccm) | AsH3 flow (sccm) | TMGa flow (sccm) | II/III ratio | Growth temperature (°C) | Growth time (s) | Height (μm) |
A | 5 | 100 | 14.4 | 0.013 | 490 | 2000 | 15.5 |
B | 10 | 100 | 14.4 | 0.025 | 490 | 2000 | 16.1 |
C | 12.5 | 100 | 14.4 | 0.031 | 490 | 2000 | 20.0 |
D | 15 | 100 | 14.4 | 0.038 | 490 | 2000 | 17–18 |
E | 20 | 100 | 14.4 | 0.05 | 490 | 2000 | – |
Table1.
The growth parameters of all samples.
Table options
-->

Download as CSV
Sample | DEZn flow (sccm) | AsH3 flow (sccm) | TMGa flow (sccm) | II/III ratio | Growth temperature (°C) | Growth time (s) | Height (μm) |
A | 5 | 100 | 14.4 | 0.013 | 490 | 2000 | 15.5 |
B | 10 | 100 | 14.4 | 0.025 | 490 | 2000 | 16.1 |
C | 12.5 | 100 | 14.4 | 0.031 | 490 | 2000 | 20.0 |
D | 15 | 100 | 14.4 | 0.038 | 490 | 2000 | 17–18 |
E | 20 | 100 | 14.4 | 0.05 | 490 | 2000 | – |
The widely accepted growth model is the vapor–liquid–solid (VLS) mechanism. In the VLS mechanism, it is assumed that the NW growth can be controlled by the direct impingement of growth material from the gas phase onto the catalyst droplet. Furthermore, the changed nanowire growth direction during growth could be understood from the kinetic coefficients of crystallization based on the theory of nucleation at constant supersaturation in eutectic droplets. The influence of the composition of the catalyst droplet may change the liquid-vapor interfacial energy. In our experiment, the interfacial energy is changed by the doping of Zn. The changed interfacial energy makes it possible for the droplet to unpin from the nanowire tip during the growth. As the atoms grow layer-by-layer on the top of NW, the displacement of the droplets may occur in the growth of every layer of atoms. When the liquid droplet unpins, it moves onto a sidewall (111) facet, and continues to grow, thus forming a kink. It is observed that the probability of the displacement of the droplet increases with the concentration of Zn in the droplet, thus kinking enhanced with the increased II/III ratio.
To estimate the doping concentration of Zn in the GaAs NWs, the I–V curves of NWs were measured by the device of NWs between two Pt/Ti/Pt/Au (200 nm) ohmic contacted electrodes. Fig. 2 shows the SEM image of the device. The measurement was divided into two groups in which the NWs came from sample A and sample D, respectively. Fig. 3 shows the I–V curves of the two groups. All the devices show a linear hole transfer characteristic due to the ohmic contact. Although the resistances of NWs should change with the doping concentration, it is hard to show the doping concentration from the I–V curves. This is because the NWs in each group have different diameters and lengths, the resistances of each NW are different even in the same group. To calculate the doping concentration, the conductivity needs to be calculated by the measured parameters of each samples such as the length of conductive channel and the diameter of each NW. The diameters and lengths are measured in the SEM image of each device and the resistance is calculated from Fig. 3. The morphology parameters and estimation resistance of these measured GaAs NWs are shown in Table 2. Taking these parameters into consideration, the conductivity can be calculated by Eq. (1):

class="figure_img" id="Figure2"/>
Download
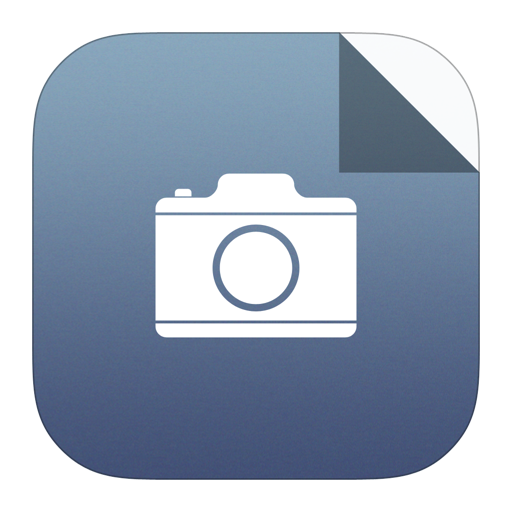
Larger image
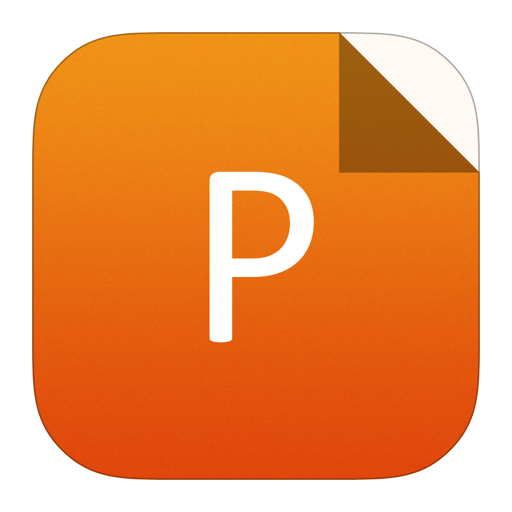
PowerPoint slide
Figure2.
SEM image of the measured device.

class="figure_img" id="Figure3"/>
Download
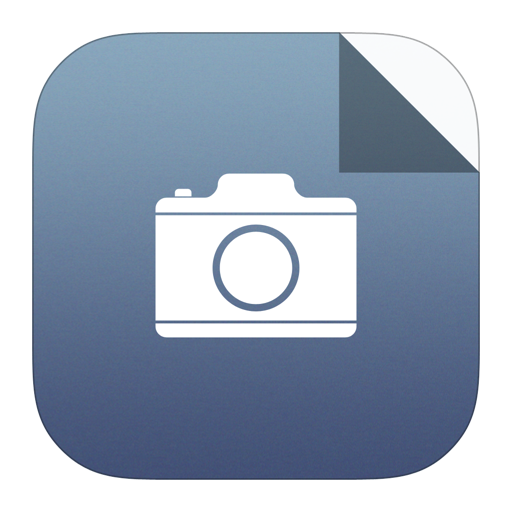
Larger image
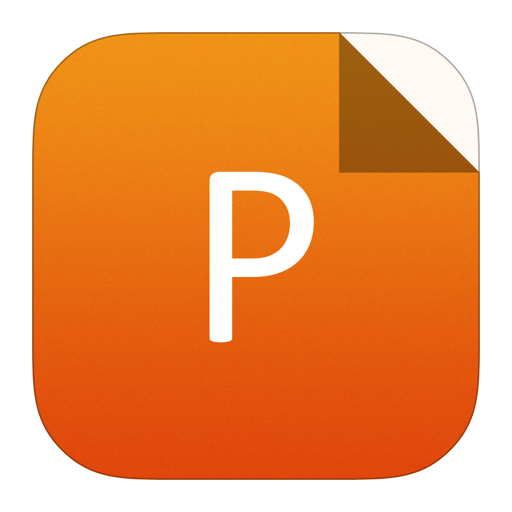
PowerPoint slide
Figure3.
(Color online) (a) I–V curves of NWs from sample A. (b) I–V curves of NWs from sample D.
Sample | Diameter (nm) | Length (μm) | Resistance (Ω) | ||
A1 | 340 | 6.59 | 1700 | ||
A2 | 890 | 5.9 | 120 | ||
A3 | 200 | 6.47 | 6500 | ||
D1 | 650 | 7.27 | 440 | ||
D2 | 345 | 6.68 | 2020 | ||
D3 | 300 | 5.88 | 2290 | ||
D4 | 150 | 6.5 | 430 000 |
Table2.
Morphology parameters and estimation resistance of these measured GaAs NWs.
Table options
-->

Download as CSV
Sample | Diameter (nm) | Length (μm) | Resistance (Ω) | ||
A1 | 340 | 6.59 | 1700 | ||
A2 | 890 | 5.9 | 120 | ||
A3 | 200 | 6.47 | 6500 | ||
D1 | 650 | 7.27 | 440 | ||
D2 | 345 | 6.68 | 2020 | ||
D3 | 300 | 5.88 | 2290 | ||
D4 | 150 | 6.5 | 430 000 |
$$sigma = frac{l}{{R{ m s}}}.$$ ![]() | (1) |
Thus, the doping concentration NA[17–20] can be estimated by Eq. (2) and Eq. (3)[21–23]:
$$sigma = pq{mu _{ m{_{p,GaAs}}}},$$ ![]() | (2) |
$${mu _{ m {_{p,GaAs}}}} = frac{{{mu _{ m {p0}}}}}{{1 + sqrt {frac{{{N_{ m A}}}}{{{{10}^{18}} { m cm}^{ - 3}}}} }},$$ ![]() | (3) |
where p is the hole concentration in the NW which approximates the doping concentration NA, μp, GaAs is the GaAs mobility with doping, μp0 is the GaAs mobility without doping which is estimated as 450 cm2/(V·s)[23, 24]. The estimation results are shown in Fig. 4. It is observed that the p-type dope concentrations under the II/III ratio of 0.013 (A) and 0.038 (B) approximate to 1019–1020 cm?3. The estimated value will be higher if the GaAs mobility without doping is used as the hole mobility of GaAs NW which is several tens of cm2/(V·s)[23, 24]. It is reported that the doping concentration increased with the enhanced diameter, which is consistent with that in our experiment[23]. Besides, it is also reported that the doping concentration increased with the enhanced II/III ratio during the unkinked growth[23]. However, when the NWs exhibit kink under high II/III ratio (sample D) due to the increased Zn in the droplet, the doping concentration seems almost unchanged compared with the low II/III ratio (sample A). That is, although the NW kinked by increasing the flow of DEZn, the high concentration of Zn in the droplet has little influence on the doping concentration. Nevertheless, the fabrication of the NW with high doping concentration that has a more stable droplet under high II/III ratio during growth still needs future investigations.

class="figure_img" id="Figure4"/>
Download
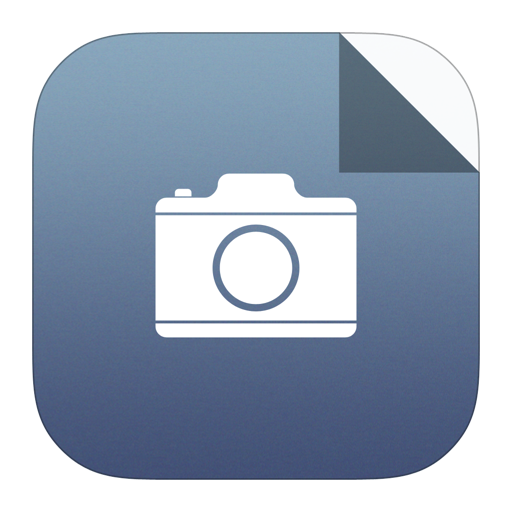
Larger image
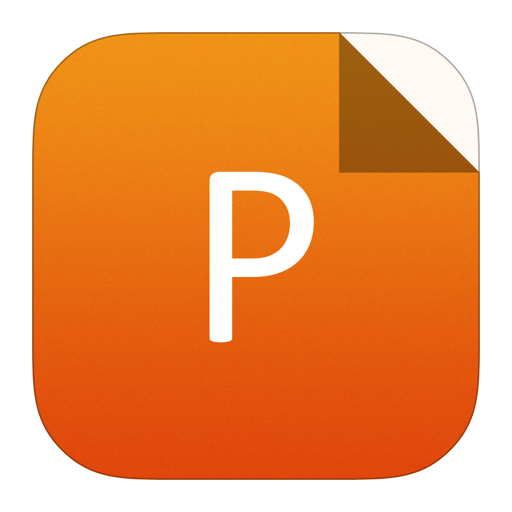
PowerPoint slide
Figure4.
(Color online) The calculated doping concentrations of measured NWs.
4.
Conclusion
In summary, p-type doping using DEZn as doping precursor during the VLS growth of GaAs nanowires was demonstrated. The II/III ratio in the samples varied in the range of 0.013–0.05. Stronger kink of the NWs with increasing DEZn flow gives evidence that Zn is incorporated via VLS mechanism, and it also indicates an upper limit of p-type doping. I–V characteristic of NWs that shows a linear dependence and the morphology parameters of measured NWs is obtained from the SEM image. Calculating by these parameters, the p-type dope concentration under the II/III ratio of 0.013 and 0.038 approximated to 1019–1020 cm?3. The p-type doping of nanowires is of general interest for all compound semiconductor nanowires. What is more, the high doping concentration of NW will play an essential role in future applications of optoelectronics and sensor technologies.