全文HTML
--> --> -->增强混合的装置一般是基于加快失稳、加快诱导涡结构原理设计的. 混合增强的方式可以分为主动增强和被动增强[6]. 被动增强是改变尾缘结构, 常见的被动混合增强构型有波瓣、锯齿等[7-9]. 主动增强是向流场周期性的注入能量, 常见的方式有机械振动、射流扰动和放电激励[10-12]. 被动增强混合虽然具有简单易行的特点, 但是不能根据情况改变. 较被动增强混合方式而言, 主动增强混合方式可以弥补这一缺陷, 并且能在增强混合的同时降低总压损失. 虽然主动增强混合是较为有效的增强混合方式, 但传统的主动增强混合手段仍存在能量较低、响应慢、需要附加气源等方面的不足.
等离子体合成射流是一种新的控制手段, 具有能量密度高、响应速度快、无需额外的气源和没有机械活动部件的优势, 弥补传统主动增强混合的不足[13-15]. 此外等离子合成射流的扰动还具有一定的方向性. 等离子体合成射流工作原理可以简单表述为: 在激励器腔内放电将气体加热, 腔体内气体受热后压力上升, 气体从小孔喷出对外膨胀做功. 等离子体合成射流不同于直接暴露在流场中的放电激励. 等离子体合成射流是通过产生的射流和压缩波对流场进行扰动. 直接暴露在流场中的放电激励是通过快速局部焦耳热效应产生的压缩波和电流体动力学效应对流场实现扰动[16].
以等离子体合成射流作为控制手段, 在超声速流动中主要应用于边界层和激波的控制[17-19], 对于混合层的控制主要还停留在亚声速. Hardy等[20]实验研究了等离子合成射流对亚声速射流的控制, 实验结果表明等离子体合成射流诱导出涡结构使射流剪切层变厚. Huet[21]采用数值仿真方法研究了等离子体合成射流对亚声速射流的流动控制, 实现了降低噪音. Chedevergne等[22]采用实验加数值仿真的方法研究了等离子体合成射流对马赫数为0.6的高雷诺数射流的流动控制机理. 作为一种对混合层的控制手段, 等离子体合成射流在亚声速中表现出较强的控制能力.
等离子体合成射流用于超声速混合层的控制目前研究的较少. 本文将采用实验加仿真的方法, 验证等离子合成射流对超声速混合层控制的有效性, 分析对比激励器布置位置不同对激励器性能以及混合增强效果的影响, 为在超声速流场中设计高效的增强混合方案提供参考.
2.1.实验风洞
实验在低噪声超声速混合层风洞中进行. 混合层风洞如图1所示, 实验段长度为350 mm, 高度为60 mm, 宽度为200 mm. 为消除流向压力梯度, 风洞的上下壁面有1°的张角. 厚度为10 mm的隔板从风洞入口到喷管出口将风洞从中间分为两部分. 风洞实验段实物图如图2所示. 风洞上侧喷管马赫数为1.37, 风洞下侧喷管马赫数为2.39, 根据对流马赫数(Mc)计算公式[23,24]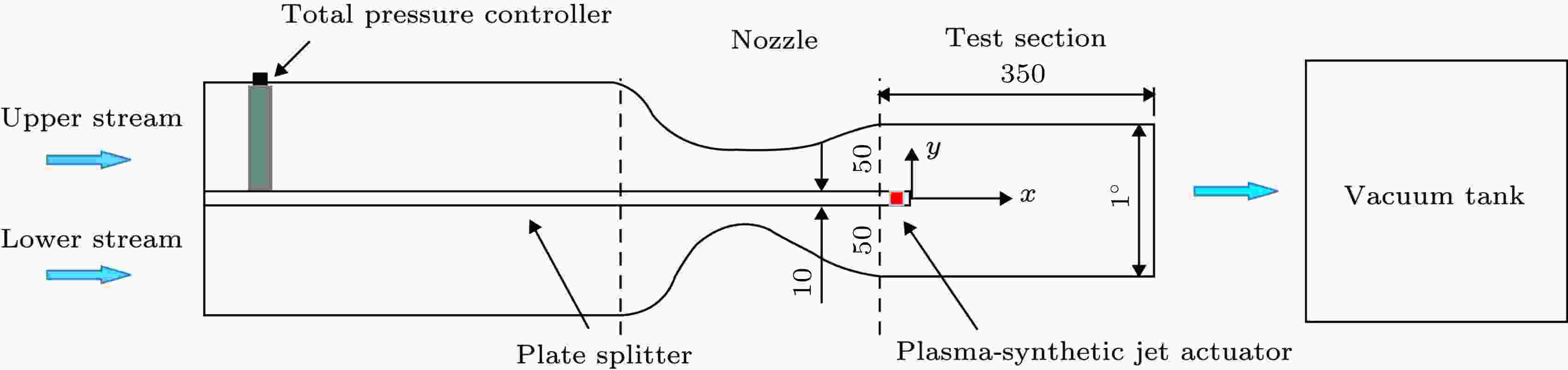
Figure1. Schematic of the supersonic mixing layer wind tunnel.
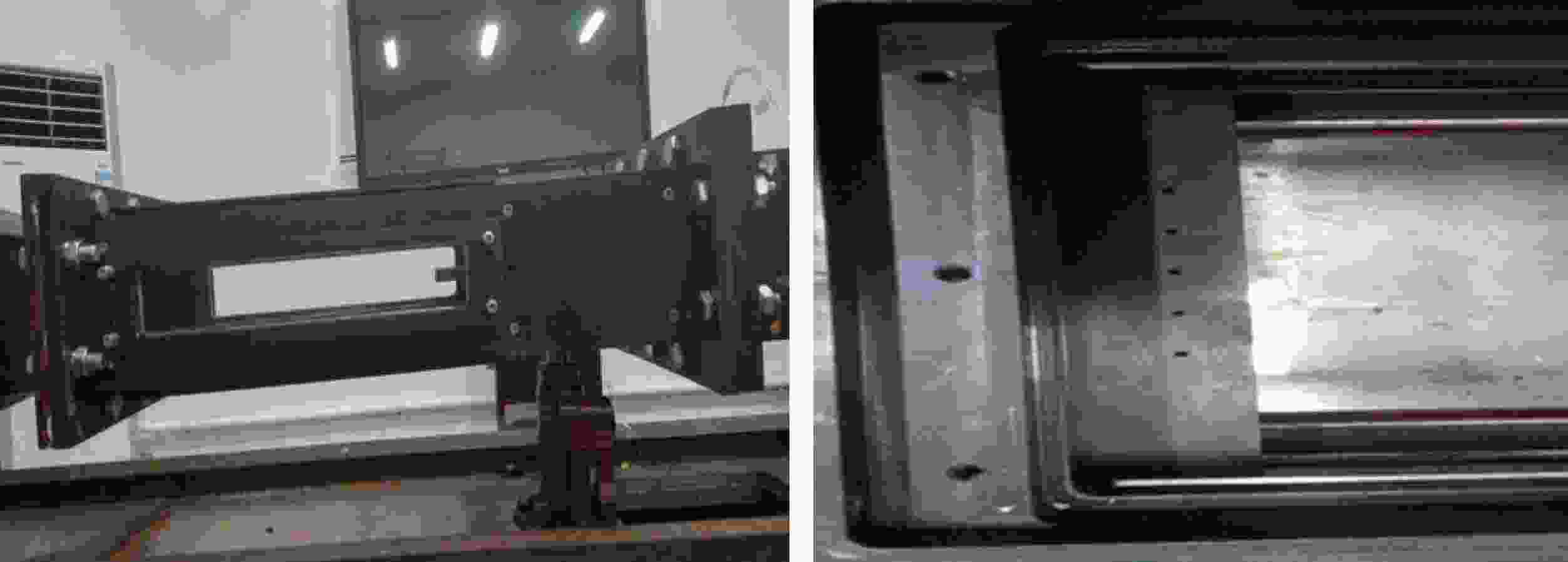
Figure2. The test section of supersonic mixing layer wind tunnel.
马赫数Ma | 速度U/m·s–1 | 静温T/K | 总温T0/K | 运动黏度μ/ 10–5 m2·s–1 |
1.37 | 405.16 | 218.39 | 300 | 1.4312 |
2.39 | 567.18 | 139.87 | 300 | 0.9635 |
表1压力匹配情况下校测流场参数
Table1.Flow parameters of supersonic mixing layer.
2
2.2.等离子体合成射流激励器
等离子体合成射流的详细原理在文献[25-27]中有较多的介绍. 图3是安装有等离子体激励器阵列隔板的示意图. X, Y, Z分别代表流向、横向和展向的方向. 激励器安装在距离隔板尾端约15 mm处, 实现对上侧气流的扰动. 5个激励器采用串联放电方式工作. 每个激励器由圆柱形放电腔体和一对电极组成. 采用抗放电烧蚀能力强的钨针作为电极, 电极直径为1 mm, 腔体采用的是树脂材料. 放电电极之间的间距为1 mm. 圆柱形放电腔体的直径为12 mm、高度为6 mm、体积为678.24 mm3. 有一个直径为2.5 mm的射流孔, 如图4所示. 电源采用KD-1高压脉冲电源[16], 最大输出电压为10 kV, 脉冲频率为1—50 Hz, 单次脉冲最大输出能量为20 J. 本次实验使用的放电电容为640 nF.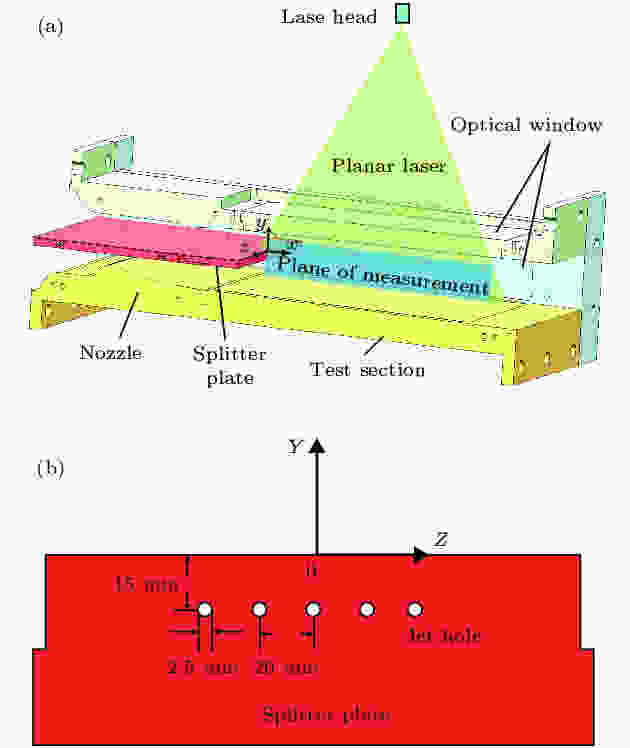
Figure3. Schematic of the wind tunnel and the actuator mounted inside a plate: (a) Splitter plate in the wind tunnel; (b) actuator in the splitter plate.
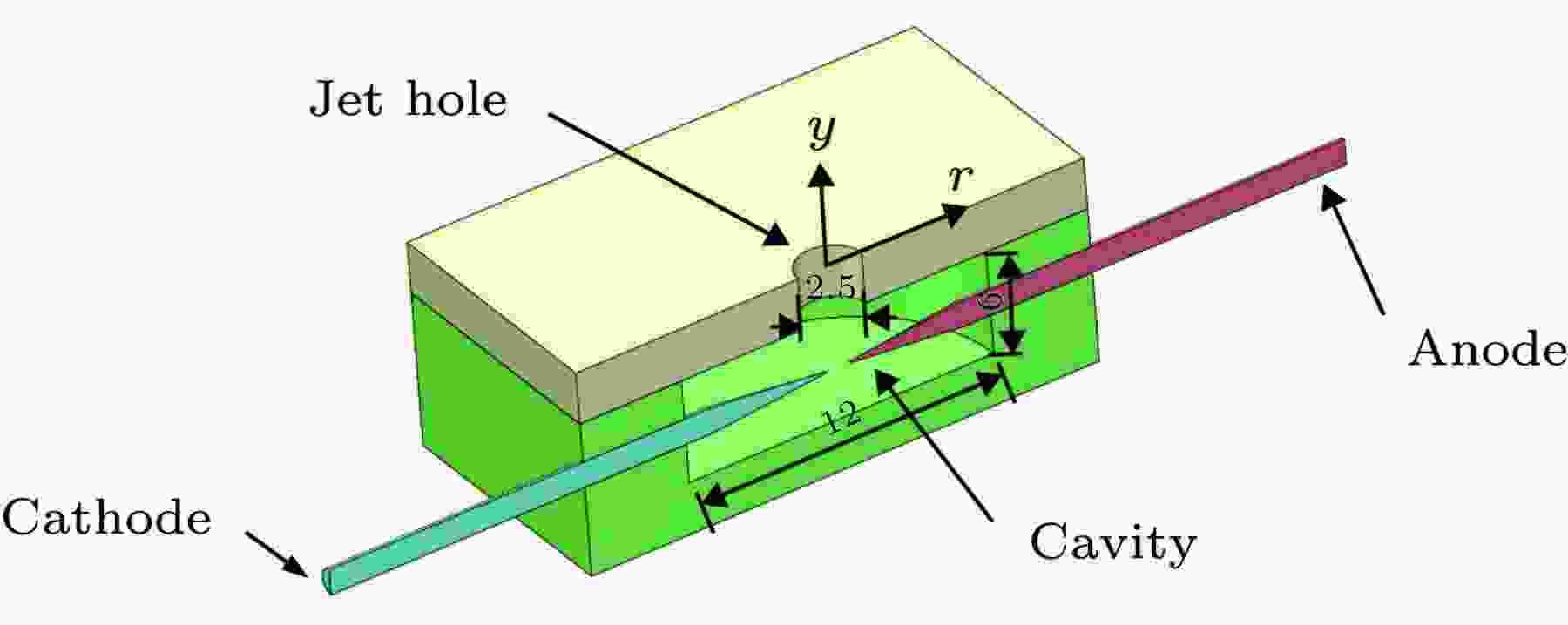
Figure4. Two-electrode plasma synthetic actuator
2
2.3.测量设备
使用纹影系统观测等离子体合成射流对混合层的扰动过程. 纹影系统主要包括光源、高速相机、凹面镜、刀口. 纹影系统与试验段的位置如图5所示. 凹面镜直径为200 mm、焦距为2 m. 光源采用的是连续的碘钨光源. 相机的曝光时间为1 μs, 拍摄频率为30000 Hz, 拍摄的像素为1024 pixel × 688 pixel.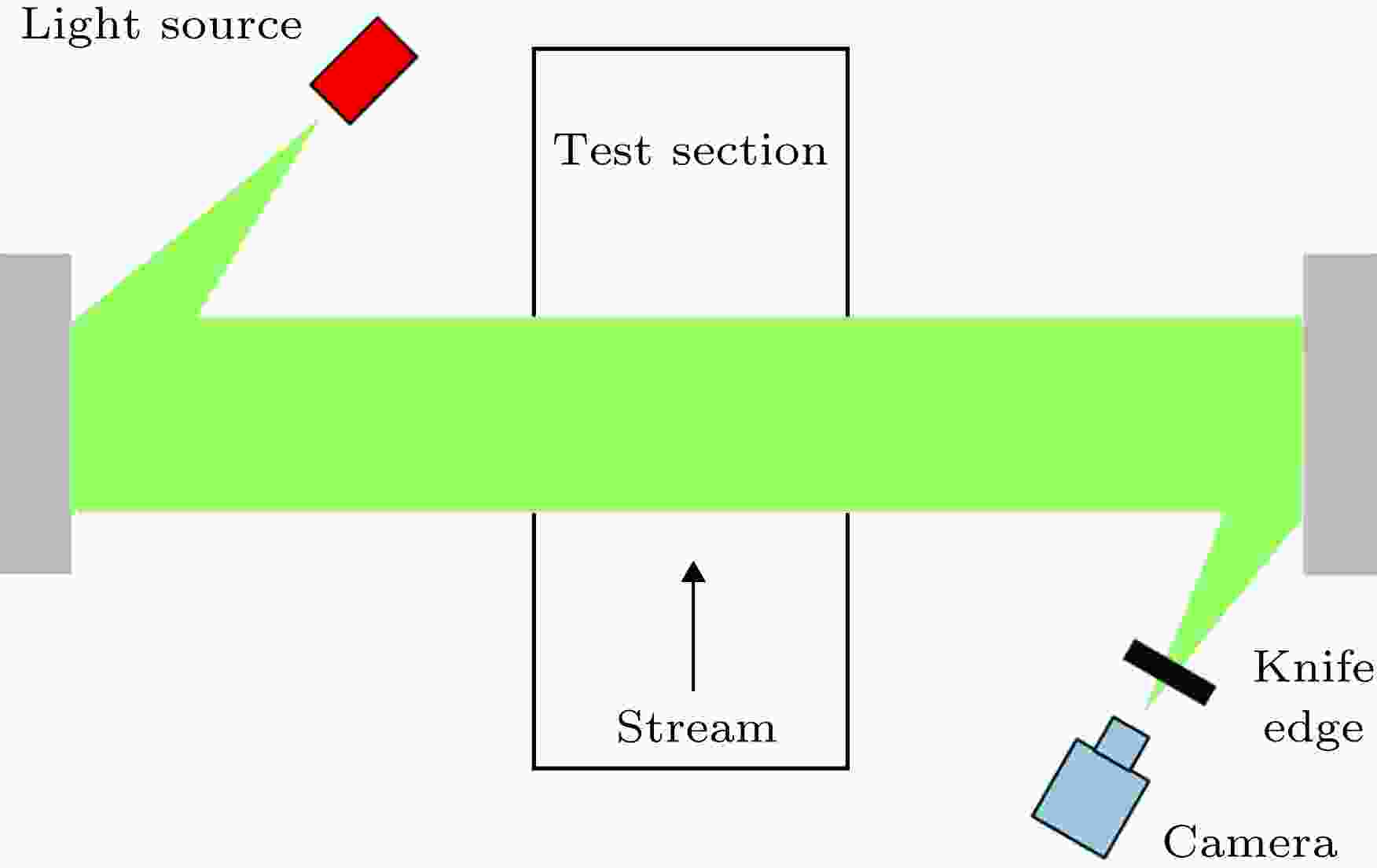
Figure5. The schematic diagram of schlieren system
实验使用基于纳米粒子的平面激光散射技术(nanoparticle-based planar laser scattering, NPLS)的系统来获取流场的精细结构. NPLS系统是由赵玉新等[28]基于瑞利散射原理开发出来的. NPLS系统包含有: 双腔Nd:YAG激光器, 波长为532 nm, 单次脉冲的能量为350 mJ, 脉冲宽度为6 ns; 一台跨帧像素为4008 pixel × 2672 pixel跨帧CCD相机; 一台控制激光器和相机的同步控制器; 一台纳米粒子发生器; 一台计算机. TiO2被选为示踪粒子, TiO2有效直径为42.5 nm, 松弛时间为66.3 ns. TiO2优势在于对超声速气流中小尺度的脉动有较好的跟随性. 流场图片的灰度图与纳米粒子的浓度成比例, 纳米粒子的浓度又与密度成比例, 所以流场图片的灰度值可以反映密度场.
粒子图像测速仪(particle image velocimetry, PIV)与NPLS系统共用一套设备. 利用纳米粒子良好的跟随性可以获得较为准确的超声速流场速度分布. CCD相机的最短曝光时间间隔为0.5 μs, 根据两幅跨帧图像以及时间间隔可以得出速度场.
NPLS/PIV的最大工作频率为2 Hz, 但是相机的曝光时间仅为6 ns, 等离子体合成射流激励器作用在流场的时间远小于1 ms. 等离子体合成射流的扰动需要一段时间之后才能传递到观测区域中间方便观测. 为满足上述的要求, NPLS/PIV需要在等离子体合成射流激励器工作一段时间之后再开启工作. 用一台信号源发生器先触发等离子体合成射流激励器, 延时一段时间触发NPLS/PIV系统. NPLS/PIV拍摄区域见图3. 实验系统的具体时序见图6.
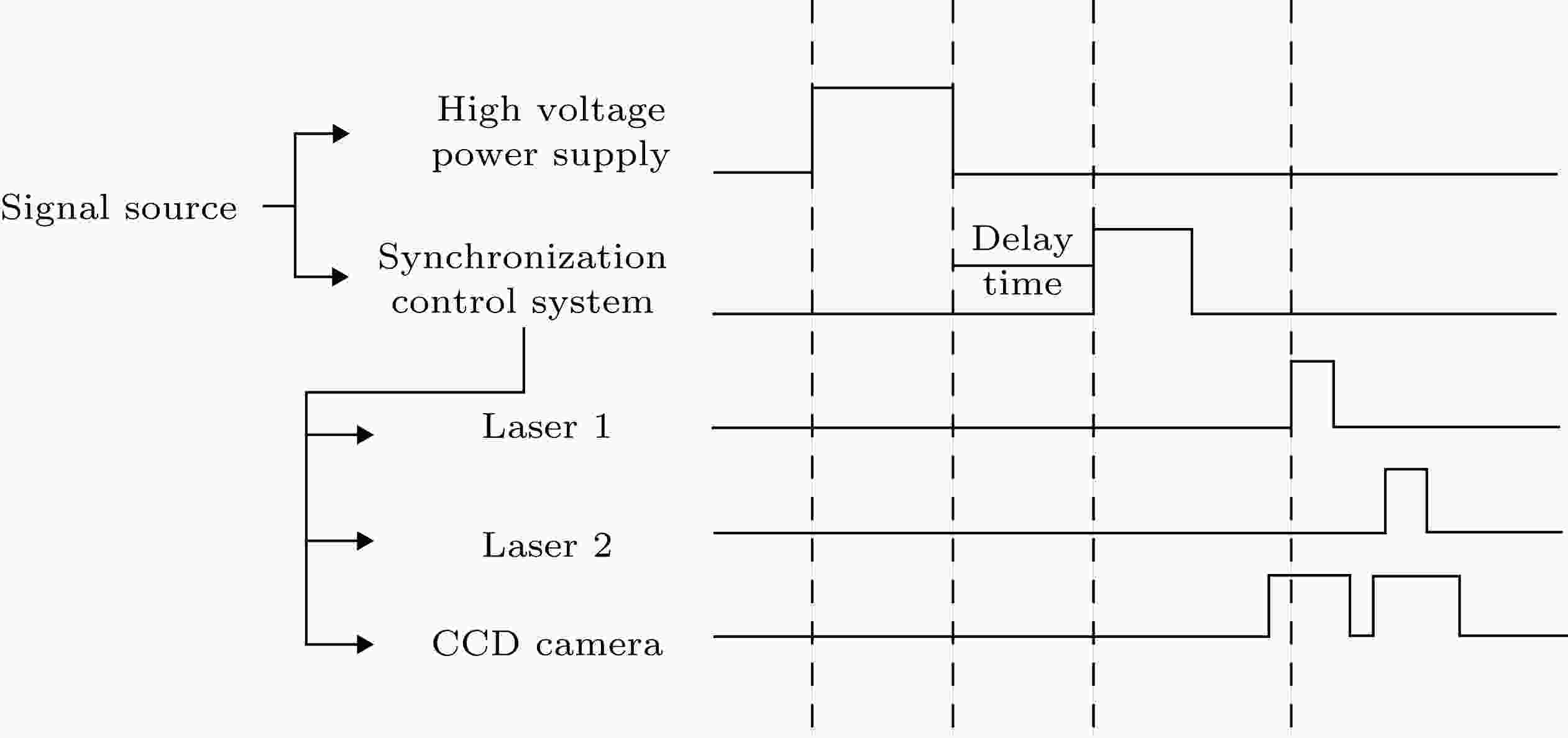
Figure6. Schematics of the experimental system sequence chart.
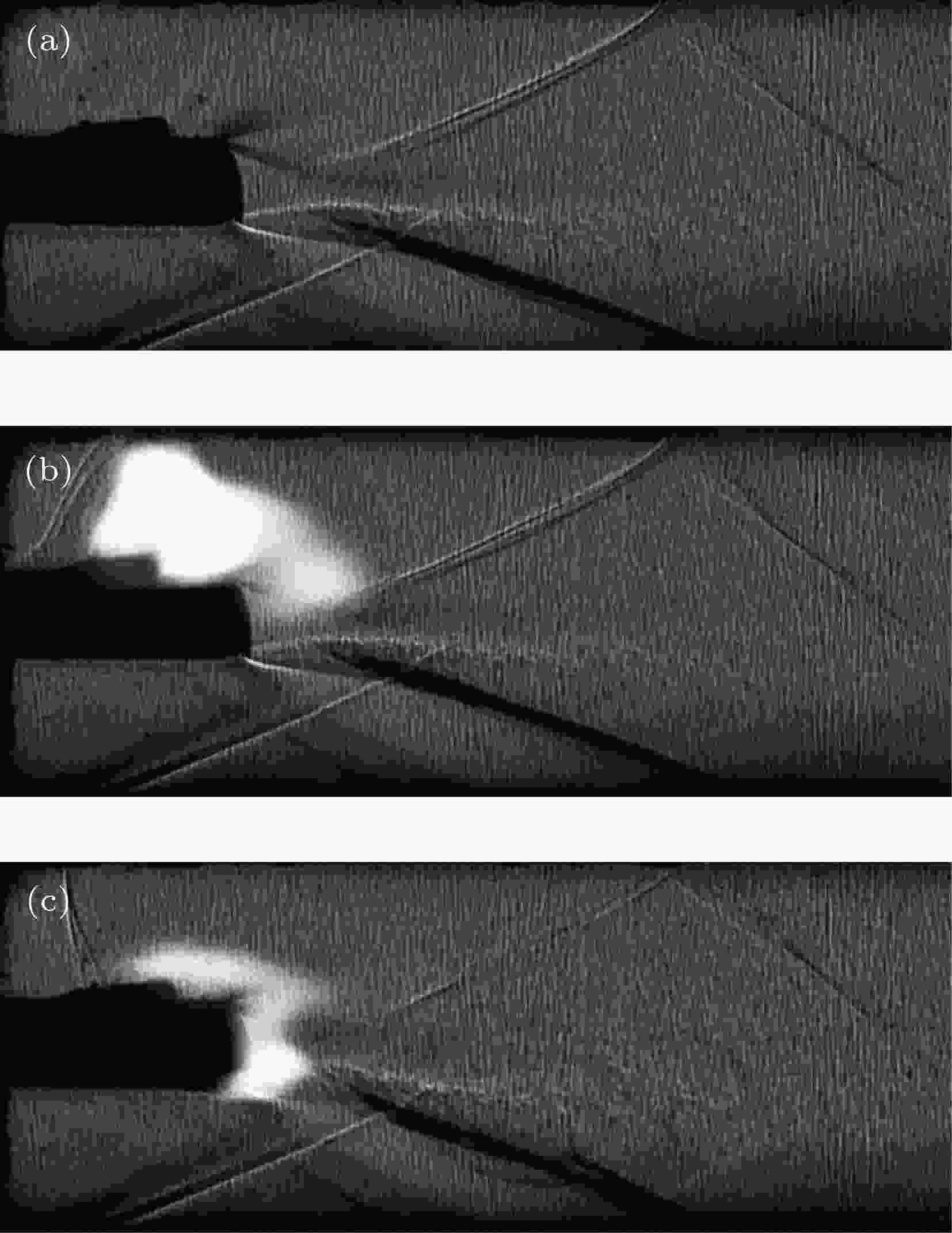
Figure7. Schlieren images: (a) T0 + 0 μs; (b) T0 + 67 μs; (c) T0 + 233 μs.
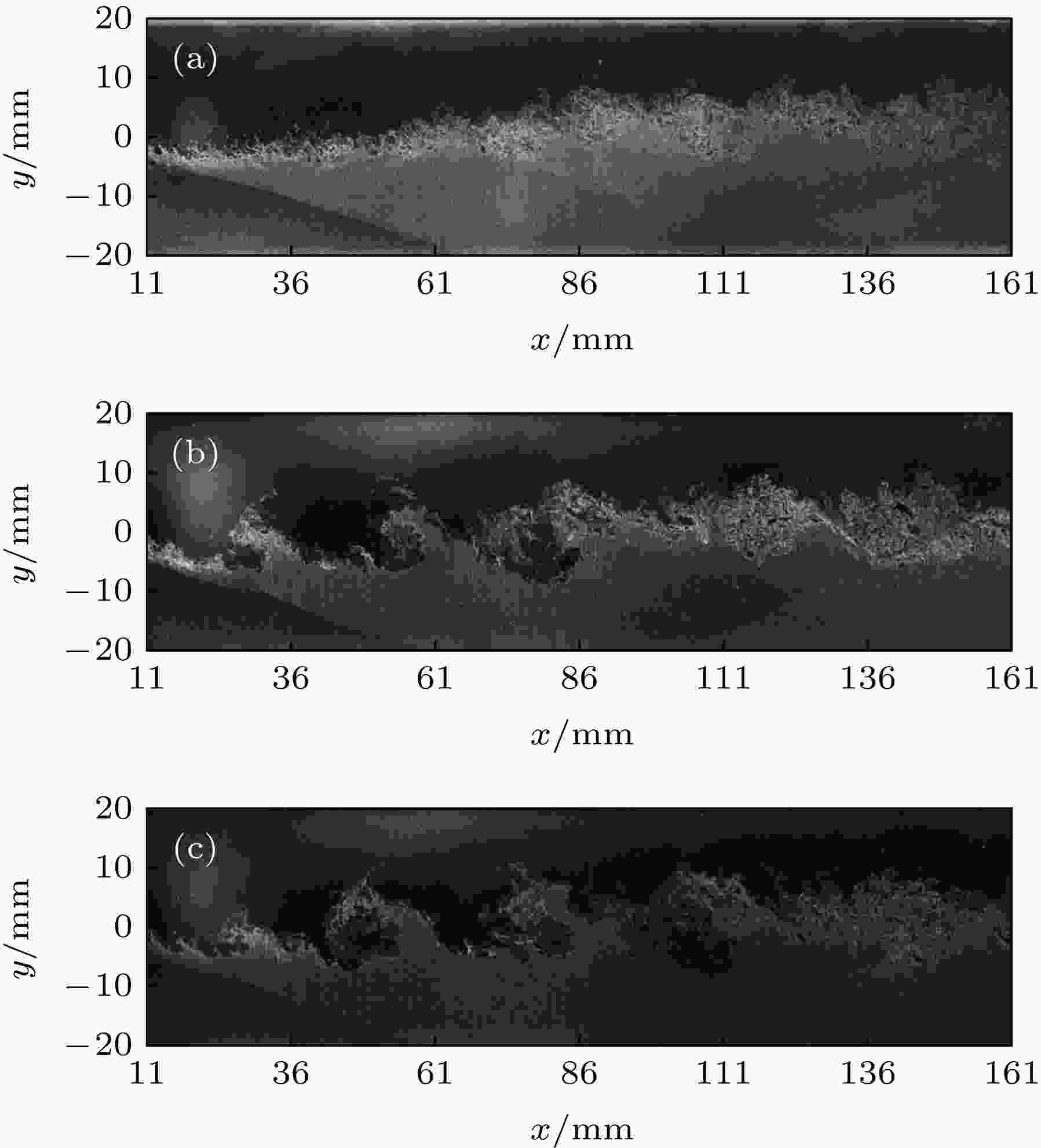
Figure8. NPLS images of supersonic mixing layer under perturbation and unperturbation: (a) Unperturbation; (b) T0 + 180 μs; (c) T0 + 230 μs.
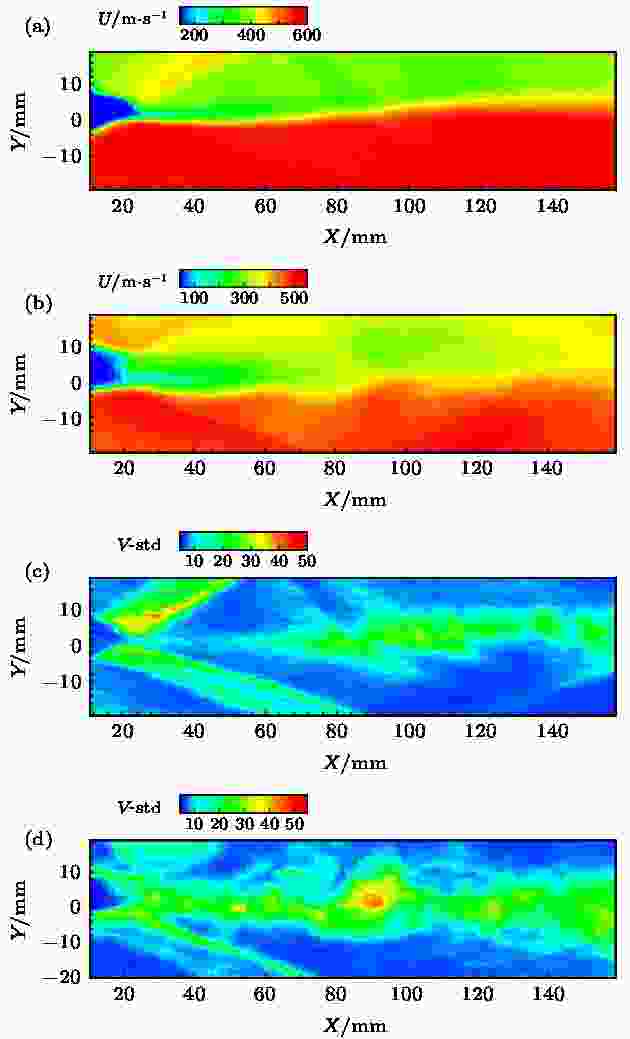
Figure9. PIV experimental results: (a) Averaged X-velocity; (b) averaged X-velocity at T0 + 230 μs; (c) Y-velocity standard deviation; (d) Y-velocity standard deviation at T0 + 230 μs.
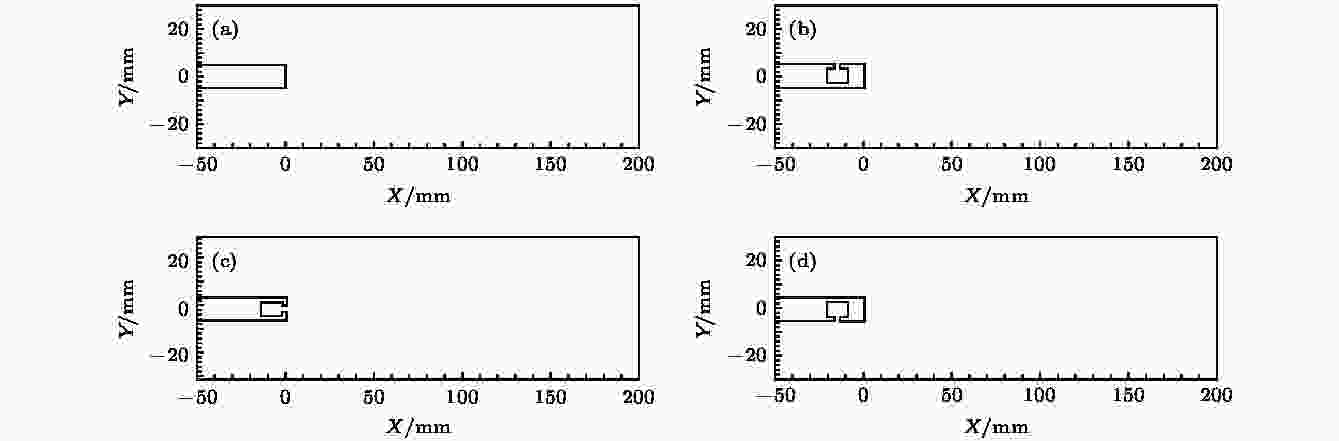
Figure10. Physical model: (a) Unperturbation; (b) the actuator at the upper surface of the splitter plate; (c) the actuator at the end surface of splitter plate; (d) the actuator at bottom surface of splitter plate.
等离子体对气体加热的过程十分复杂, 本文将其简化为一个热源. 将放热过程持续时间设置为10 μs, 假设热量为恒定输出. 根据文献[30], 其释放热效率取为10%, 热源密度为



2
4.1.算例及网格无关性验证
仿真模型以及网格加密示意图如图11所示, 使用结构化网格对流场进行网格划分, 对隔板上下表面进行网格加密, 隔板上下表面第一层网格为2 × 10–6 m, 以确保y+ ≤ 1, 满足大涡模拟对第一层网格的要求. 对混合层所在区域进行y方向加密. 在流场的入口以及隔板尾端进行x方向加密.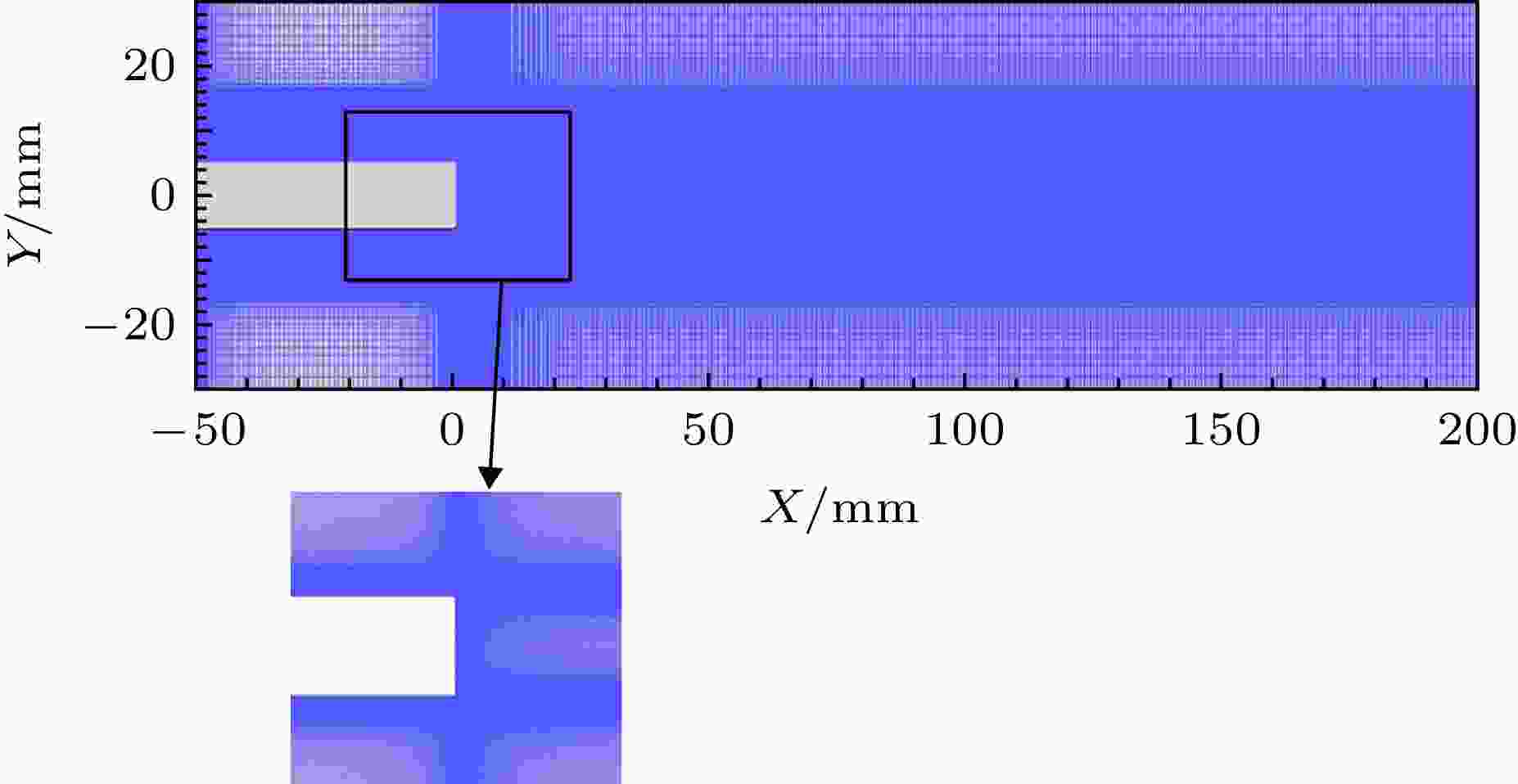
Figure11. Calculation model and grid for code validation.
使用三套网格进行网格无关性验证, grid-1网格量为179640, grid-2网格量为334804, grid-3网格量为742480. 图12为未受扰动工况下三套网格的数值仿真结果和实验结果在流向x = 150 mm处的流向速度剖面曲线. 从图12中可以看出三套网格结果相差不大, 说明满足网格无关性的要求. 本文选取grid-2网格做计算.
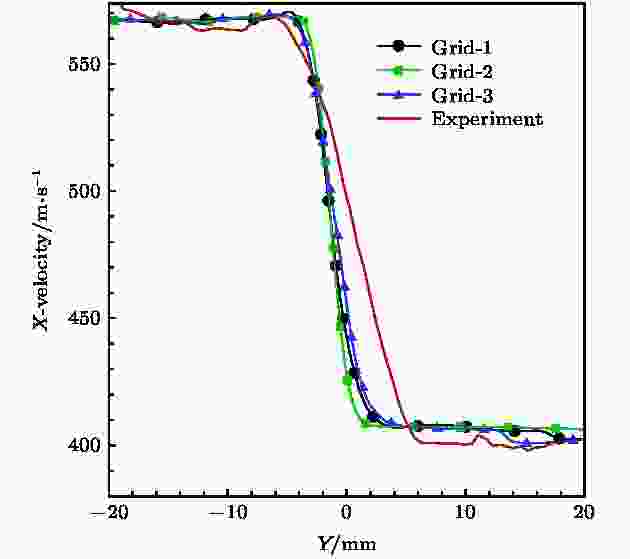
Figure12. Certification of grid independence and code validation
算例验证主要包括两个方面, 一是计算方法是否可以准确的仿真超声速混合层; 二是计算方法是否可以仿真等离子体合成射流对超声速流场的扰动. 对于对超声速混合层的数值仿真, 从图12中可以看出数值仿真结果与实验结果在上侧和下侧气流处略有偏差. 对于流场上侧和下侧出现偏差的原因主要是: 1)PIV系统本身存在1%左右的误差; 2)作为数值仿真边界条件的流场测量参数存在测量误差. 在流场的混合区域, 数值仿真结果与实验结果存在一定的误差. 从图7和图8可以看出, 流场中存在着安装激波、隔板尾端的膨胀波以及混合层相遇产生的再附激波, 经过波系后混合层向上侧倾斜, 并且涡量增大混合更加均匀. 这样就出现了图12中的结果, 实验结果的混合区域比数值仿真结果位置偏上, 并且实验结果比数值仿结果速度曲线过渡更加平滑. 总体来说, 采用的计算方法合理且可行.
等离子体合成射流对超声速流场扰动的算例验证, 仿真采用图10(b)所示的物理模型. 使用纹影结果和NPLS结果与数值仿真结果进行对比. 如图13所示, 在(T0 + 67 μs)时刻的数值纹影和纹影实验结果进行对比, 可以看出在射流孔的上游都产生了斜激波. 如图14所示, 将(T0 + 180 μs)时刻数值仿真密度场和NPLS结果进行对比, 可以看出扰动传递到的地方会产生较大的涡结构, 在扰动未传播到的地方, 涡结构较小. 但是数值仿真的扰动传播距离要小于实验中扰动传播的距离. 由于采用二数值仿真, 其腔体大小和出口直径不能完全反映实验工况. 激励器腔体内放电过程较为复杂, 仿真时只是将放电能量沉积过程简化为一个热源对腔体气体加热过程, 其能量传递给腔体气体的值也难以准确估算. 因此仿真与实验结果存在一定的差距. 但是数值仿真可以反映超声速混合层受等离子体合成射流扰动的基本形态和发展趋势, 可以进行定性的对比分析.
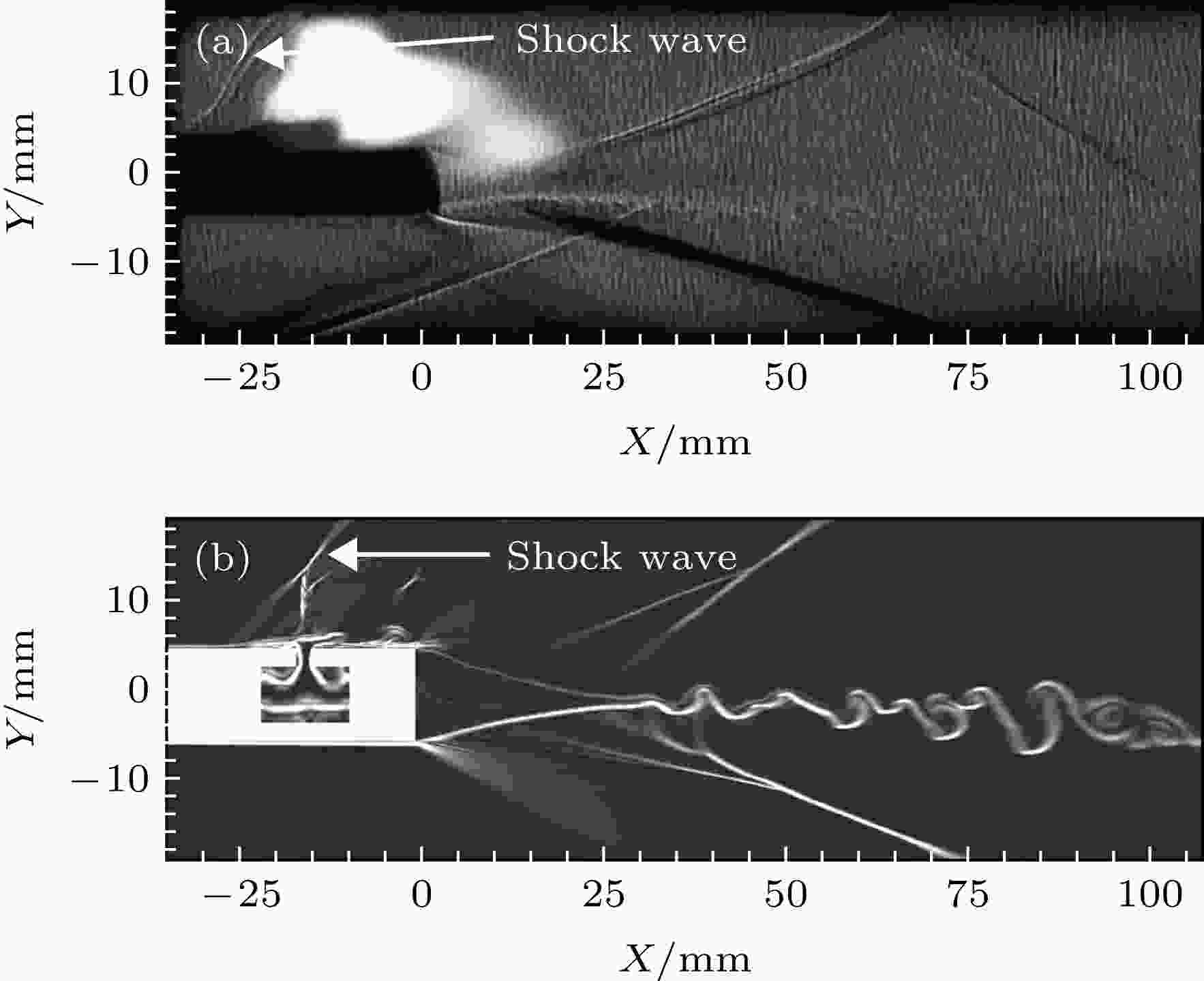
Figure13. T0 + 67 μs, numerical and experimental schlierens: (a) Experimental schlieren; (b) numerical schlieren.
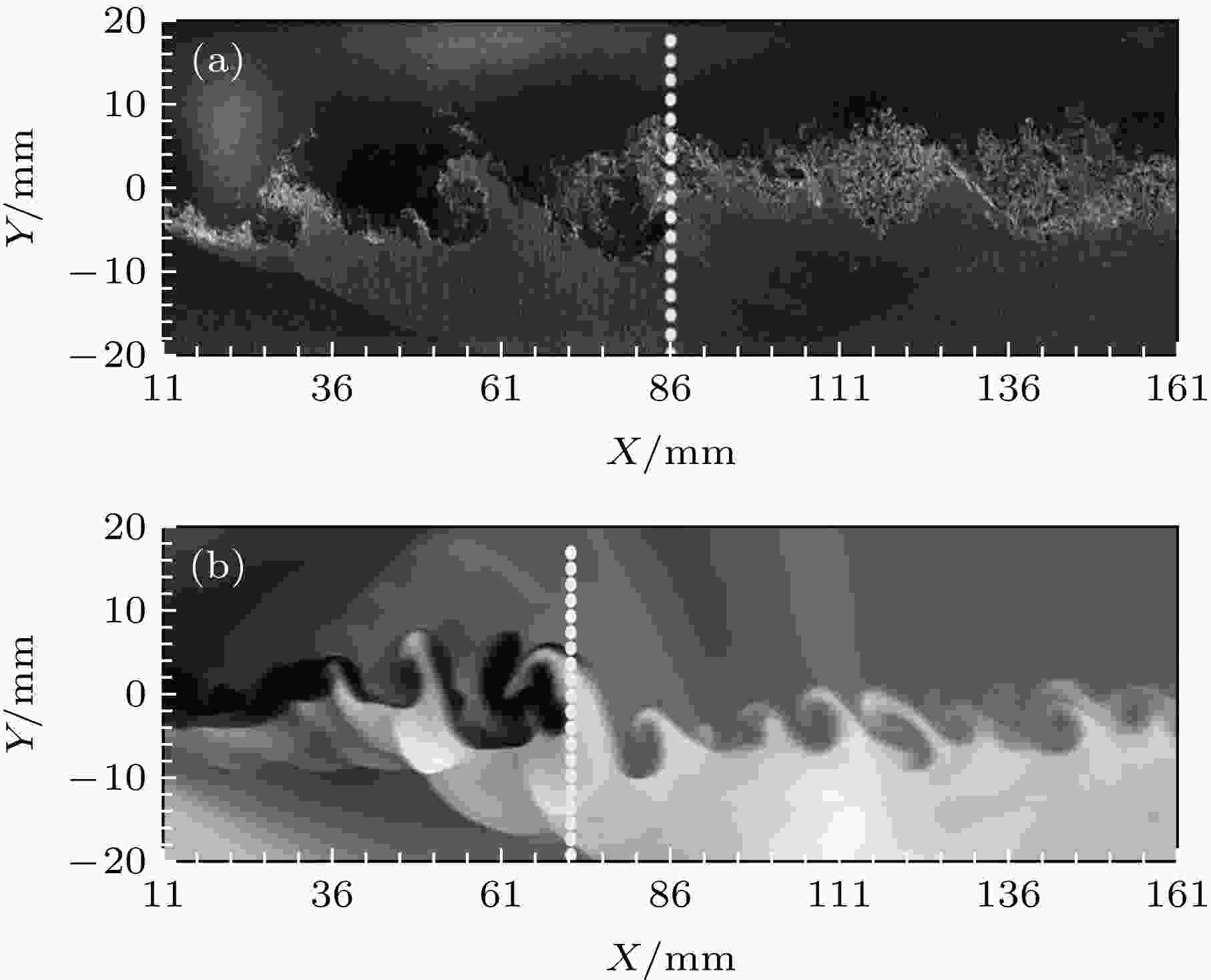
Figure14. T0 + 180 μs, contour of density and NPLS result: (a) NPLS result; (b) contour of density.
2
4.2.激励器位置不同对混合层的影响
图15所示为(T0 + 555 μs)时刻瞬时数值仿真密度场, 与未受扰动的工况对比, 可以看出这三个工况涡结构都有明显的增大, 扰动已经影响到了整个流场, 诱导出连续大尺度涡结构.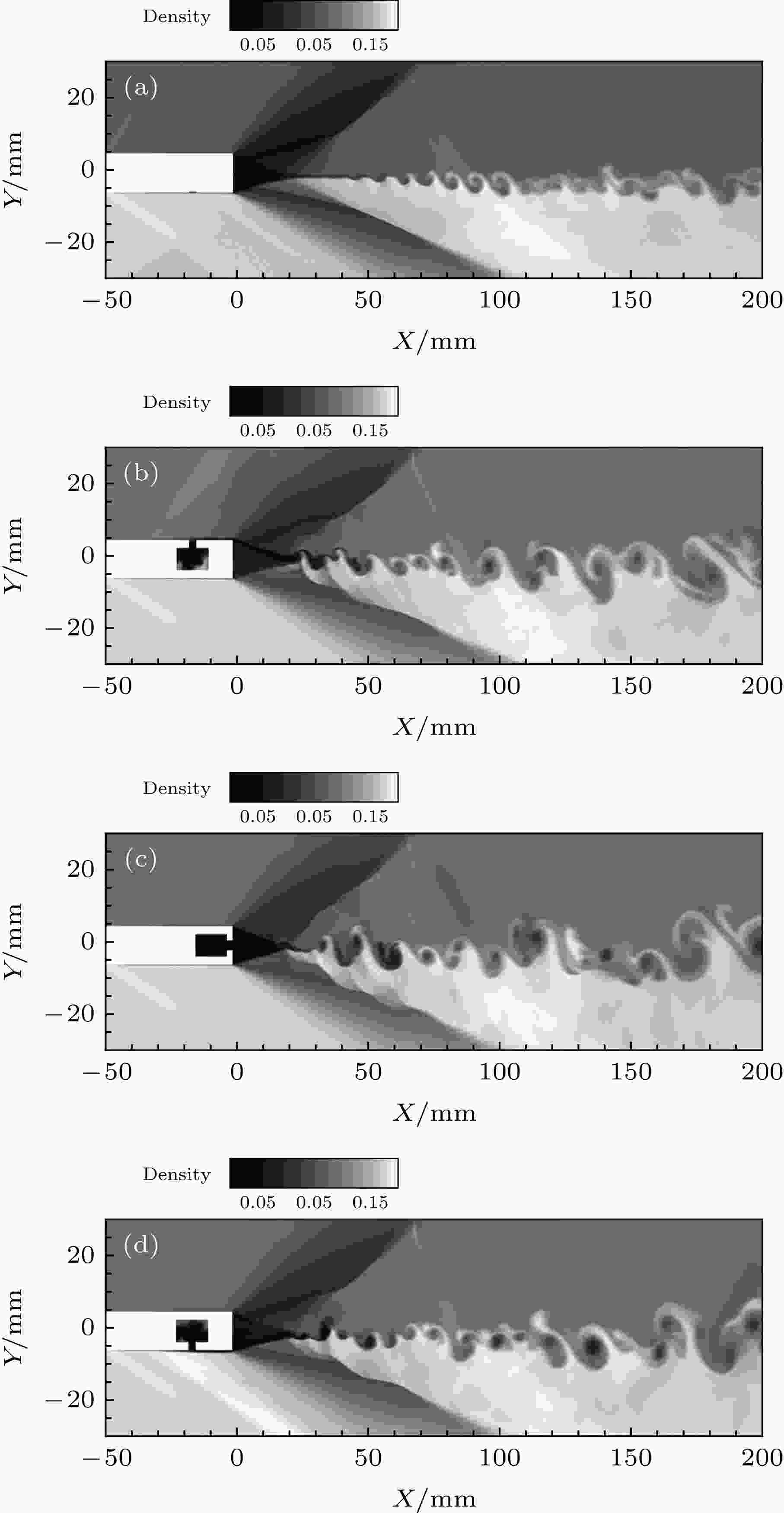
Figure15. Contours of density at T0 + 555 μs: (a) Unperturbation; (b) the actuator at the upper surface of the splitter plate; (c) the actuator at the end surface of the splitter plate; (d) the actuator at the bottom surface of the splitter plate.
图16为(T0 + 75 μs)瞬时温度云图和流线仿真结果. 图16(a)中激励器在隔板上表面布置, 可以看出热气流喷出后, 形成一个虚拟型面将来流抬高, 周期性的射流喷出可以实现气流的上下摆动, 使得y方向速度脉动量增加, 有助于气流掺混均匀. 图16(b)中激励器在隔板的尾端布置, 可以看出等离子体合成射流喷出后直接作用在混合层的再附点上, 从而加快混合层失稳, 达到增强混合的效果. 并且由图16(a)和图16(b)这两个图可以推知, 由于在隔板尾端布置的激励器可以直接作用在混合层上, 因而混合层对在隔板尾端布置的激励器扰动响应最快.
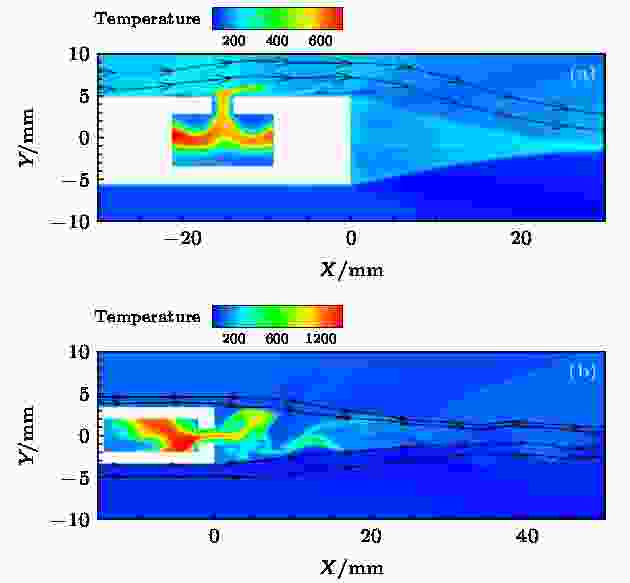
Figure16. Simulation of the temperature and flow: (a) The actuator at the upper surface of the splitter plate; (b) the actuator at the end surface of the splitter plate
图17为555 μs内的时均速度厚度曲线. 混合层速度厚度δ定义为归一化速度


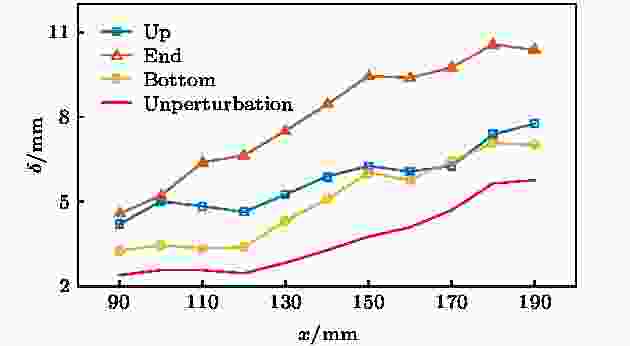
Figure17. Time-averaged velocity thickness of mixing layer
2
4.3.布置位置不同对激励器性能的影响
图18为激励器出口参数. 由于在隔板尾端外部压力较小, 喷出射流获得了较大的速度, 因此气体膨胀做功转化的动能较多, 因而出口的动量是这三个工况中最大的. 而在隔板上下表面布置的激励器, 射流与来流相互作用, 气体膨胀做功转化为动能较少. 但是由于上下两股气流的引射造成隔板尾端布置的激励器腔体内气体密度较小, 因而喷出的射流质量流量最小. 同时可以看出在隔板尾端布置激励器出口压力也小于激励器布置在隔板上下表面的工况.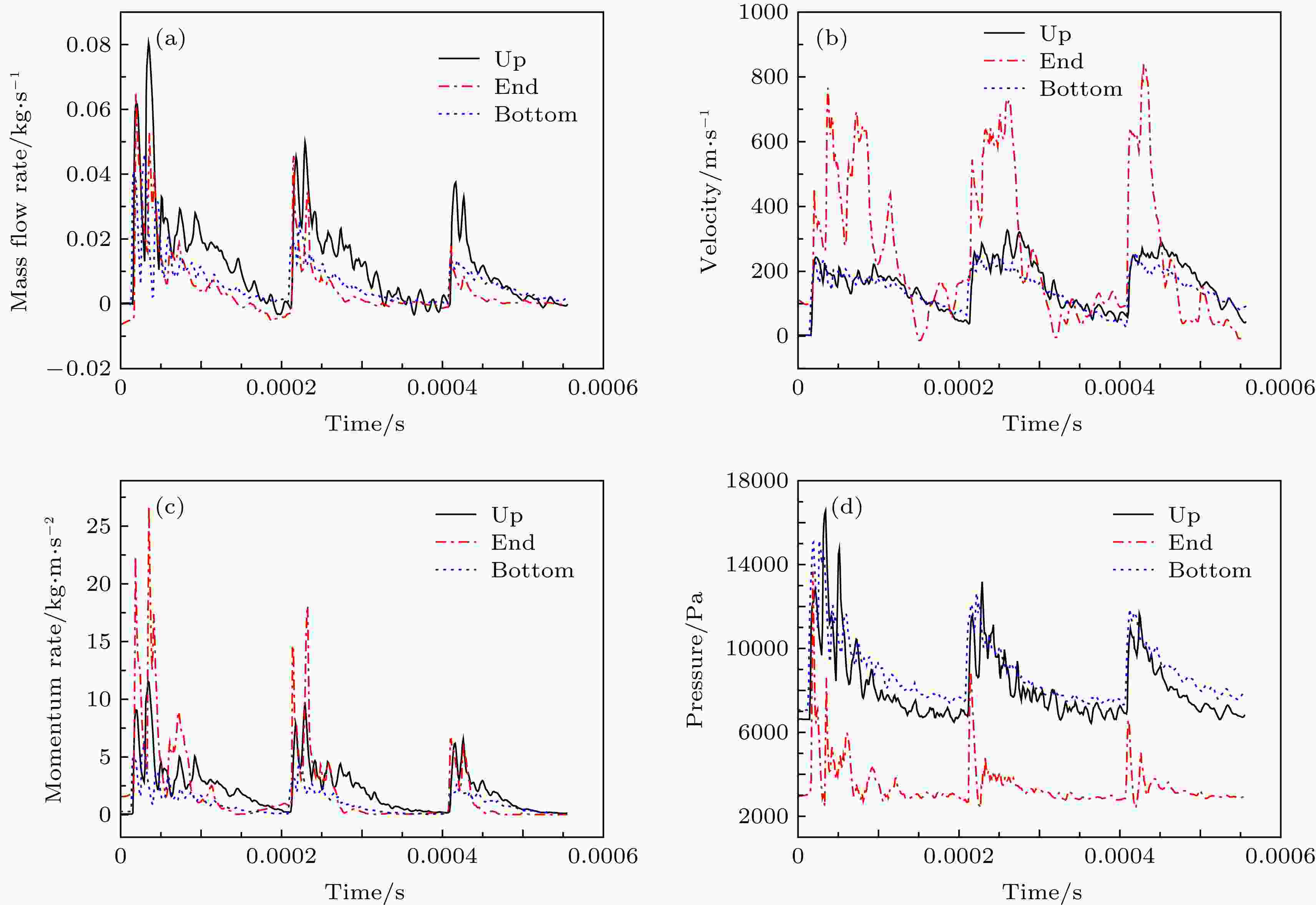
Figure18. The parameters of actuator outlet: (a) The mass flow rate of actuator outlet; (b) the velocity of actuator outlet; (c) the momentum rate of actuator outlet; (d) the pressure of actuator outlet.
图19为激励器腔体内参数. 图19(a)为激励器腔体内气体密度曲线图, 从图中可以发现, 腔体内密度随着放电次数的增加而逐渐降低. 这是由于算例设置的激励器腔体是绝热壁面, 做功过后腔体内温度难以降低, 腔体内维持一定压力造成外部气体内难以回吸. 这样就导致随放电次数增多, 激励器做功能力下降. 对于高频的激励器来说, 应该采用六方氮化硼陶瓷等导热能力强的材料作为激励器腔体, 或者采用冲压式激励器[32]. 图19(b)为激励器腔体温度曲线图, 在热源释放热量相同, 腔体体积相同的情况下, 腔体内气体温度的变化与密度成反比. 由于激励器布置在不同位置造成腔体内密度不相同, 因此温度变化也不相同. 在隔板尾端布置的激励器由于气体密度最小, 所以温度升高也最高. 图19(c)为激励器腔体内压力曲线图, 可见激励器布置在隔板上下表面的工况腔体内的最大压力是相同, 而在隔板尾端布置的激励器腔体内的最大压力小于其他两个工况. 这可能是由于上下两股气流的引射, 造成腔体内密度较小, 因而最大压力要小于其他两个工况的最大压力. 同时可以看出这三个工况达到峰值压力的时间差别不大.
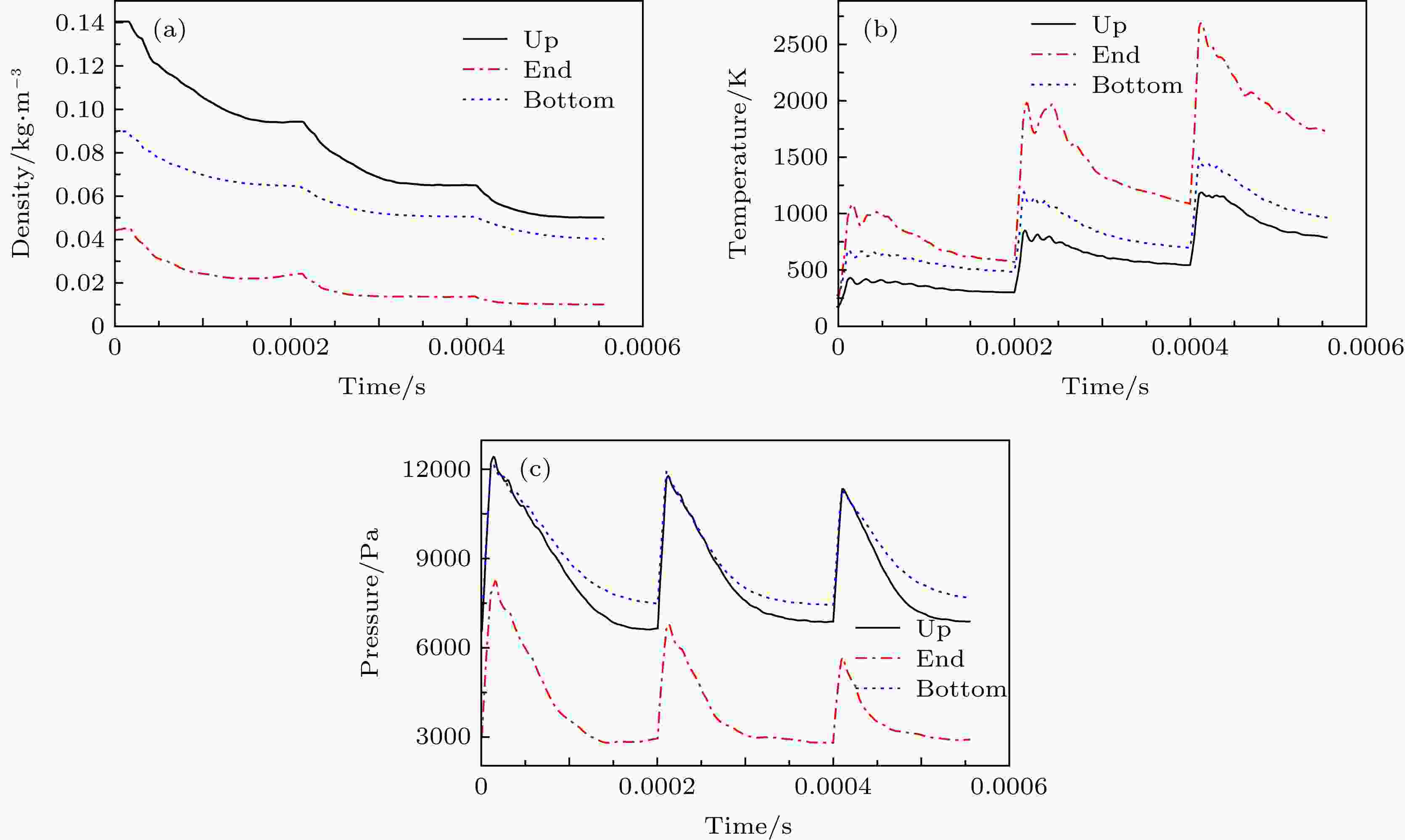
Figure19. Parameters of actuator cavity: (a) Density of the gas in the actuator chamber; (b) temperature of the gas in the actuator chamber; (c) pressure of the gas in the actuator chamber.
1)激励器出口布置隔板上下表面作用机理与激励器出口布置在隔板尾端的作用机理不相同. 布置在隔板上下表面激励器先作用在来流上, 然后再影响混合层发展, 激励器出口在隔板尾端的射流作用在混合层再附点上, 加速混合层的失稳. 并且可以推知混合层对在隔板尾端布置的激励器响应最快.
2)在激励器腔体内气体吸收的热量是在相同的前提下, 位置不同导致激励器出口的外部环境差别较大, 因而对等离子体合成射流做功能力的影响很大.
3)高频等离子体合成射流激励器对气体回吸要求较高, 只有气体及时回吸才能将保证激励器做功能力不衰减. 因此在设计等离子体合成射流激励器时应该采用导热性能好的材料或者采用冲压式设计, 保证每次做功激励器腔体内气体密度符合要求.