全文HTML
--> --> -->2004年, Sundar等[1]成功制备了有机小分子材料红荧烯单晶场效应晶体管, 载流子迁移率高达15 cm2/(V·s); 2007年, Luo等[2]在超高真空的条件下, 采用热蒸发的方法生长了红荧烯薄膜. 同年5月, Park等[3]采用热蒸发的方法, 通过控制衬底温度以及退火温度, 制备了非晶及多晶的红荧烯薄膜. 2012年, Deng等[4]采用真空蒸镀的方法, 通过控制蒸镀时间制备了具有多晶结构的红荧烯薄膜. 同年, Ng等[5]成功制备了基于rubrene∶MoO3混合薄膜的有机光电器件, 实现了宽带隙材料在近红外区的应用, 证明了rubrene在MoO3诱导下产生中间能级, 形成电荷转移络合物. 2017年, Liu等[6]成功制备了基于电荷产生层为C60/rubrene∶MoO3、电荷注入层为LiF/Al的串联式有机发光二极管, 其电流效率和能量效率分别为单一发射单元器件的2.8倍和1.9倍. 研究表明, 价带较低的宽带隙过渡金属氧化物具有很高的电子亲和能, 对P型材料分子显示出强烈的相互作用[7—10], 氧化钼(MoO3)是其中的典型代表. 2011年, 杨海刚等[11]利用磁控溅射的方法, 通过金属钼靶材在Ar+O2气氛中反应溅射制备了氧化钼薄膜, 并通过X射线衍射(X-ray diffraction, XRD)对薄膜样品结构进行了表征, 发现MoO3薄膜样品均为非晶态. 同年, 王娜娜等[12]采用MoO3作为阳极缓冲层, 制备了结构为ITO/MoO3/P3HT/C60/Bphen/Ag的有机太阳能电池器件, 研究了MoO3薄膜厚度对器件性能的影响. 到目前为止, 未见有关不同比例rubrene∶MoO3混合薄膜的光学性质研究的文献报道. 本文制备了五种不同比例的混合薄膜, 并对其光学性质进行研究.
欧姆接触广泛应用于各种器件的金属电极制备上, 在实际运用上, 主要采用隧道效应的原理在半导体上制造欧姆接触[13]. 显然, 无论是高温退火还是重掺杂都不适用于有机半导体器件的电极制备. 电荷转移络合物明显增大有机材料的载流子浓度和迁移率, 且可根据半导体器件的需要选择合适的材料配比. 关于不同比例的rubrene∶MoO3混合薄膜的电学性质的研究尚未有报道. 本文通过一系列实验, 寻找一种合适的比例, 使rubrene∶MoO3的混合薄膜与金属电极的接触形成欧姆接触, 为各种器件的制备提供了帮助. 同时, rubrene在MoO3的诱导下产生中间能级, 形成电荷转移络合物, 使得混合薄膜在近红外区域表现出强烈的敏感性, 因此rubrene∶MoO3混合薄膜在近红外区域显示出潜在的应用前景.
经过一系列实验, 在真空度为7.0 × 10–4 Pa的高真空条件下, 经过对rubrene和MoO3薄膜分别进行计时计厚的蒸镀实验, 计算出每种薄膜在不同温度下的蒸发速率, 以保证后续不同比例混合薄膜的蒸镀实验. 通过控制rubrene和MoO3的蒸发温度, 在衬底温度为室温的条件下分别制备了rubrene∶MoO3为4∶1, 2∶1, 1∶1, 1∶2, 1∶4等几种比例的混合薄膜, 利用Veeco Dektak 150型台阶仪对厚度进行测量, 薄膜厚度均为200 nm.
利用Veeco-Multimode型原子力显微镜对样品的表面形貌进行表征; 利用XD-3型X射线衍射仪对样品的晶体结构进行测量; 利用SHIMADZU UV-3600型紫外光谱仪对样品的吸收和透射进行测量; 制备了ITO/rubrene∶MoO3/Al这种“三明治”式的器件, 利用4200-SCS型半导体测试仪对混合薄膜的电学性质进行了测量.
3.1.混合薄膜结构表征
图1是通过原子力显微镜 (atomic force microscope, AFM)得到的rubrene和MoO3在不同混合比例条件下的表面形貌图, 薄膜衬底为硅衬底, 扫描范围为2.0 μm × 2.0 μm. 从图1可以看出, 不同浓度的MoO3确实对薄膜表面的平整度产生了影响. 在本实验所测量的几个比例中, 当rubrene与MoO3的掺杂比例为2∶1时, 薄膜表面的平整度最佳. 图2给出了不同混合比例条件下的薄膜表面粗糙度RMS (rough mean square), 和薄膜的AFM图对比具有很好的一致性. 随着混合比例的不同, 薄膜表面粗糙度RMS从0.907 nm逐渐下降到0.602 nm. 从图2可知: 五种混合比的薄膜表面粗糙度RMS均在1 nm以下, 说明rubrene和MoO3两种材料的分布较均匀; 但由于两种材料的混合比例不同, 薄膜表面粗糙度RMS又出现差别, 混合比为2∶1时最小, 1∶2时最大, 当混合薄膜中MoO3浓度超过50%时, 薄膜表面粗糙度迅速增加, 这是由于当MoO3浓度过高时, 混合膜不能较好地进行分子排列的优化, 导致薄膜局域化优先成核生长[14]. 另外, 在电场作用下, 薄膜表面粗糙度也会对载流子迁移率造成影响, 而且较大的粗糙度起伏会对界面态密度产生影响, 进而对其电学性质造成不良影响[15], 关于表面粗糙度对电学性质的影响后面将有讨论.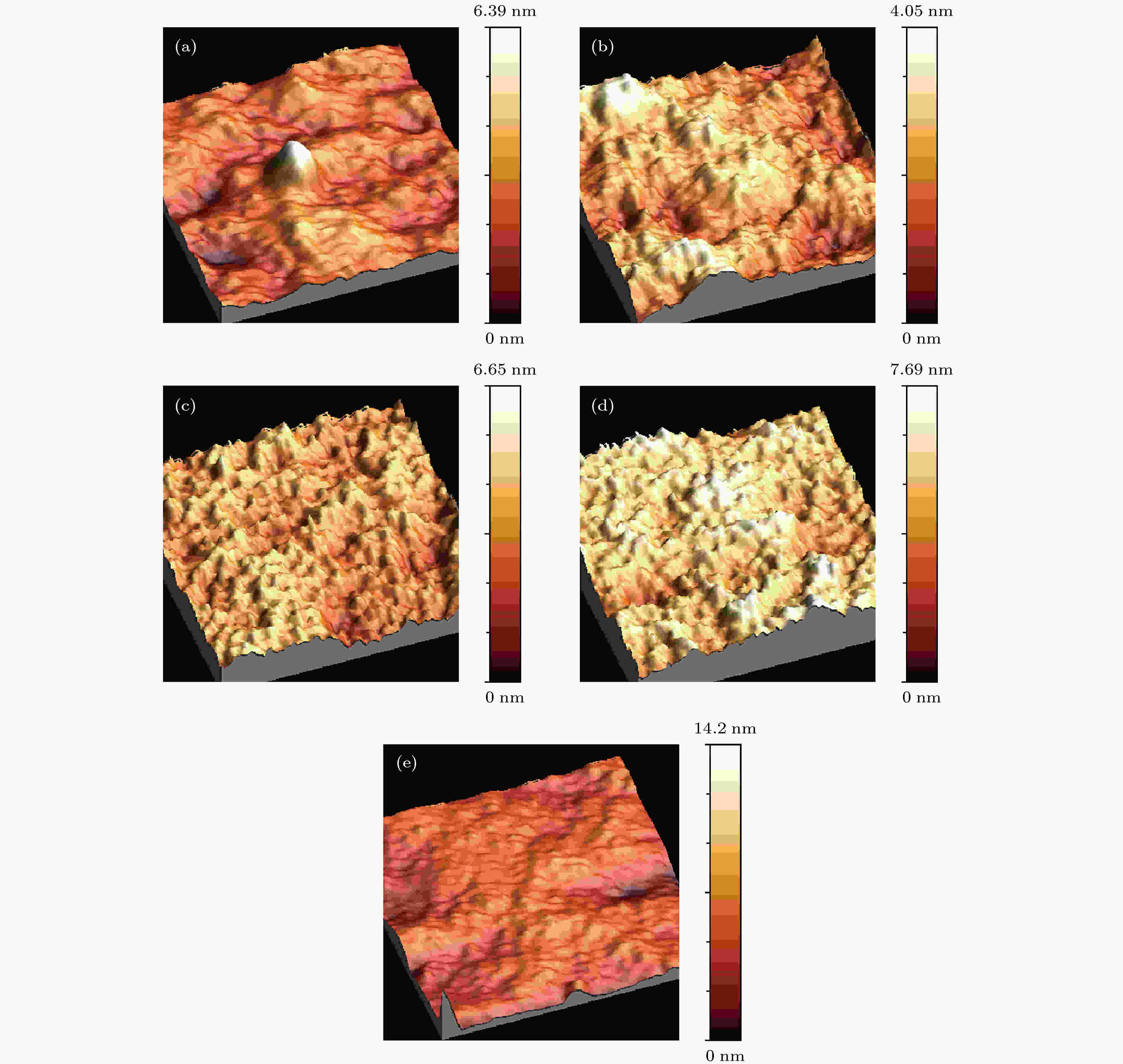
Figure1. The AFM images of rubrene∶MoO3 films under different proportion (scan areas are 2.0 μm × 2.0 μm): (a) 4∶1; (b) 2∶1; (c) 1∶1; (d) 1∶2; (e) 1∶4.
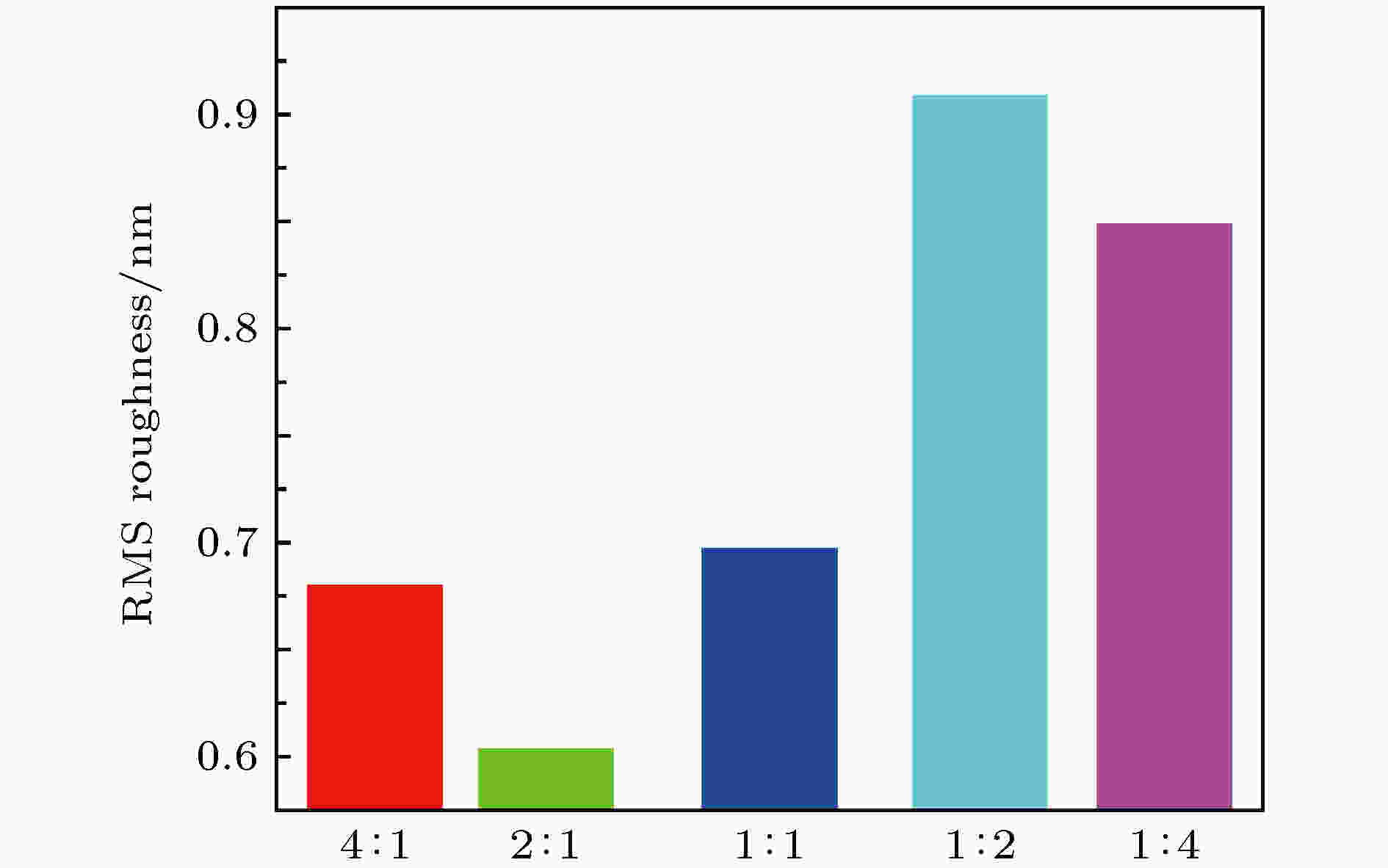
Figure2. The RMS roughness of rubrene∶MoO3 films under different proportion.
本文利用单晶Si(400)作为衬底, 对不同掺杂比例的混合薄膜做了XRD分析, 如图3所示. 从图3可以看出, 在69.8°方向上出现一个特征峰, 据文献[16]报道, 这个特征峰为Si衬底的特征峰. 44°附近出现的是Si表面SiO2对应的衍射峰. 当混合比例为4:1和2:1 (MoO3浓度较小)时, 33°非晶峰中有明显的衍射峰, 而当混合比例为1∶1, 1∶2和1∶4 (MoO3浓度较大)时, 33°非晶峰中的衍射峰消失, 说明当混合薄膜中MoO3含量较少时, 薄膜表面有Rubrene晶粒出现, 图1(a)和图1(b)中的凸起正说明此问题, 33°非晶峰中的衍射峰即为Rubrene衍射峰. 从图3可以看出, 由于薄膜比较薄, 并且衬底温度是室温, MoO3并未晶化, 因此MoO3的掺杂浓度对混合薄膜的晶体结构不产生影响, 任何比例的混合薄膜都表现为非晶态特征.
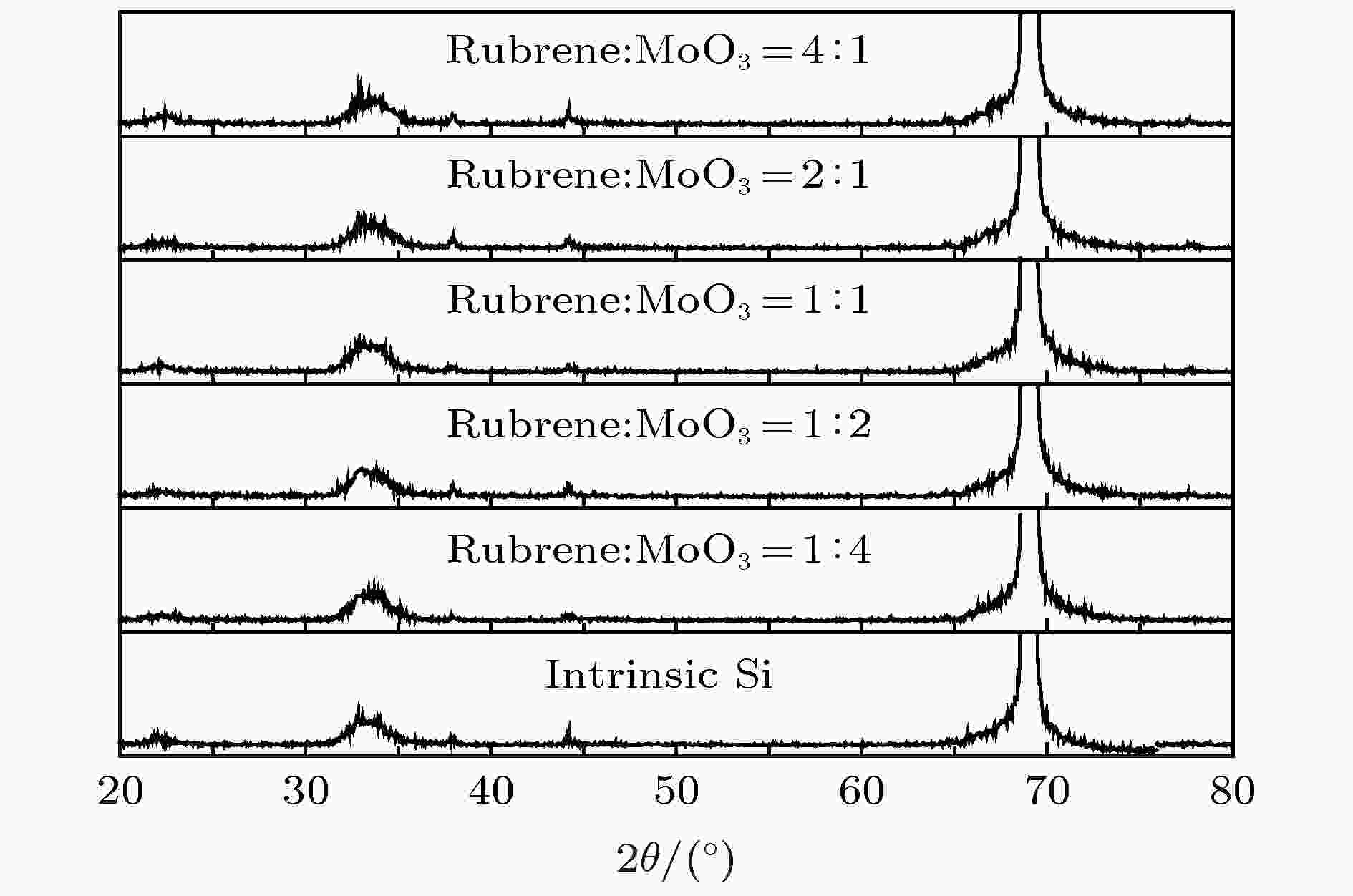
Figure3. The XRD image of rubrene∶MoO3 films under different proportion
2
3.2.混合薄膜的光学和电学性质
不同掺杂比例的混合薄膜的光致发光(PL)特征如图4所示. 通过对比不同掺杂浓度的混合薄膜发现, 在所研究的五种比例中, 当rubrene∶MoO3等于4∶1和2∶1时, 混合薄膜的PL谱峰波长均为567 nm附近, 两种比例的能隙值基本相同, 后面的讨论进一步说明这个问题. 随着混合薄膜中MoO3浓度的继续增加, 薄膜的光致发光峰发生蓝移. 从该图可以看出: 混合薄膜在近红外区域(~760 nm)还有一个明显的吸收峰, 而rubrene和MoO3的光学带隙分别为2.2 eV(~565 nm)和3.49 eV(~356 nm)[5,17,18], 且rubrene的最高占据分子轨道(HOMO)和MoO3的导带(CB)并未发生重叠, 其能级结构示意图如图5(a)所示, 对于rubrene和MoO3, 在近红外区域均不会有吸收. 然而, 当rubrene和MoO3混合时, rubrene和MoO3的真空能级突然中断, 如图5(b)所示, rubrene和MoO3之间形成电荷转移络合物[5], 在近红外区域出现吸收, 说明rubrene和MoO3混合薄膜对近红外光比较敏感, 在近红外区域显示出潜在的应用前景. 该图还说明, MoO3的掺杂能使混合薄膜的PL谱峰强度发生明显的改变.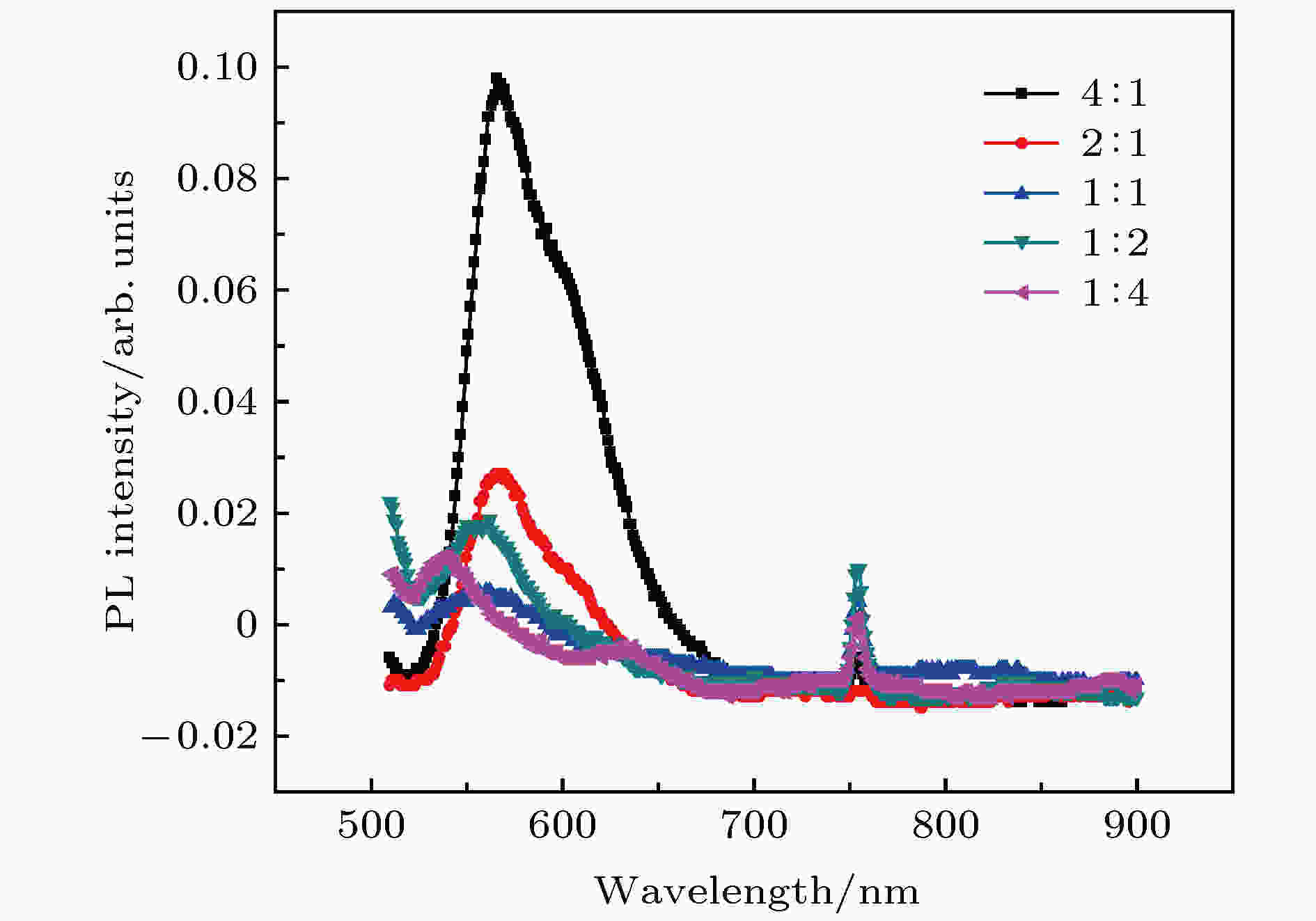
Figure4. The PL spectrum of rubrene∶MoO3 films under different proportion.
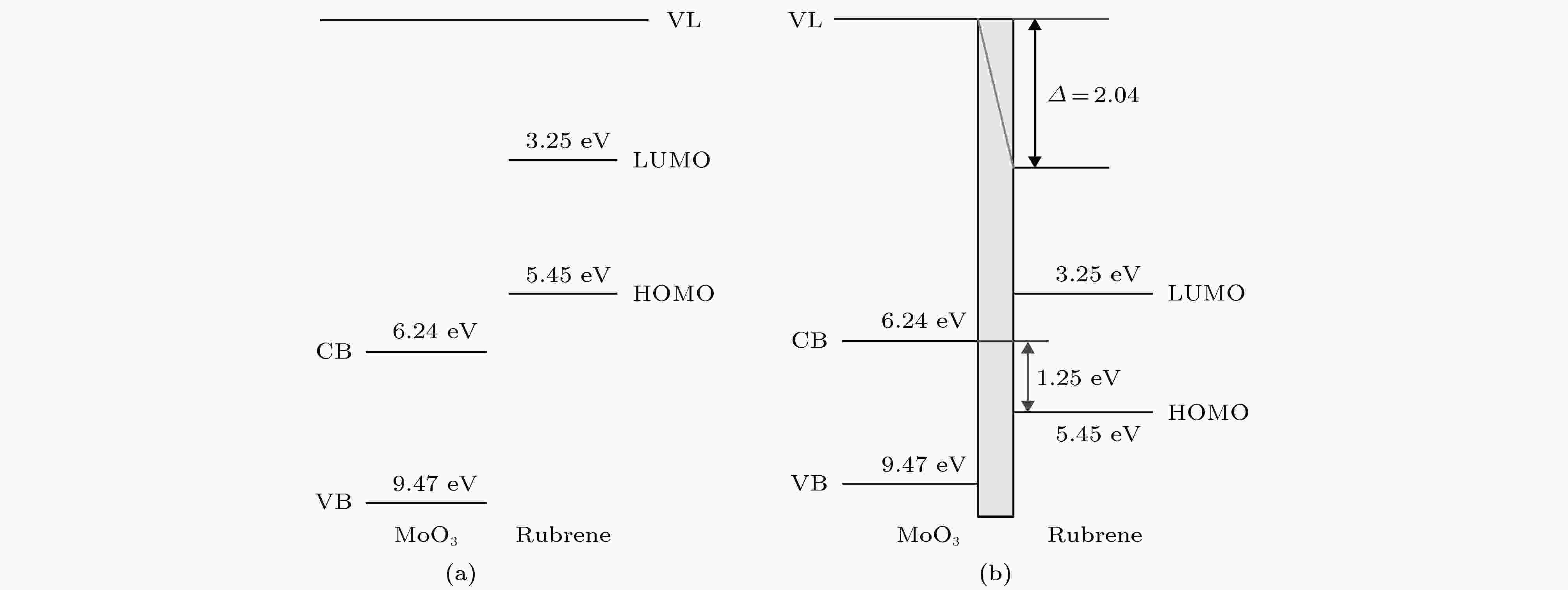
Figure5. A schematic diagram of energy level alignments about MoO3 and Rubrene: (a) Before interaction; (b) after interaction
为了利用带间跃迁理论分析光学吸收[19—21], 更好地研究混合薄膜的光学性质, 给出了混合薄膜的吸收光谱, 如图6所示, 其中纵坐标表示吸收率A. 混合薄膜在496 nm和533 nm处出现了多峰结构, 这种多峰的吸收光谱可以归为红荧烯的S0→S0跃迁的达维多夫(Davydov)分裂, 这种分裂现象的产生与红荧烯的结构性质有关[22—24]. 混合薄膜除了出现多峰结构, 在300 nm附近还有明显的吸收峰出现, 这个吸收峰来自于红荧烯, 由红荧烯薄膜中最高占据分子轨道到最低未占据分子轨道之间的吸收系数决定[25]. 混合薄膜的吸收强度明显增强, 且随着掺杂浓度的不同, 吸收还是略有区别, 在所研究的五种成分当中, 除rubrene与MoO3的比例为4∶1时对应的峰值波长较短, 其余四种比例的峰值波长基本相同, 说明在浅紫外区域, MoO3的掺杂对混合薄膜的吸收影响不大, 但随着MoO3掺杂浓度的增大, 混合薄膜在近红外区域出现明显的吸收, 说明rubrene和MoO3相互作用产生中间能级(如图5(b)), 形成电荷转移络合物. 但除混合比例为2∶1的混合薄膜之外, 其他4种比例对应的薄膜表面平整度不好, 表面比较粗糙, 通过对薄膜电学性质的讨论发现, 薄膜表面平整度对薄膜的电学性质产生较大影响.
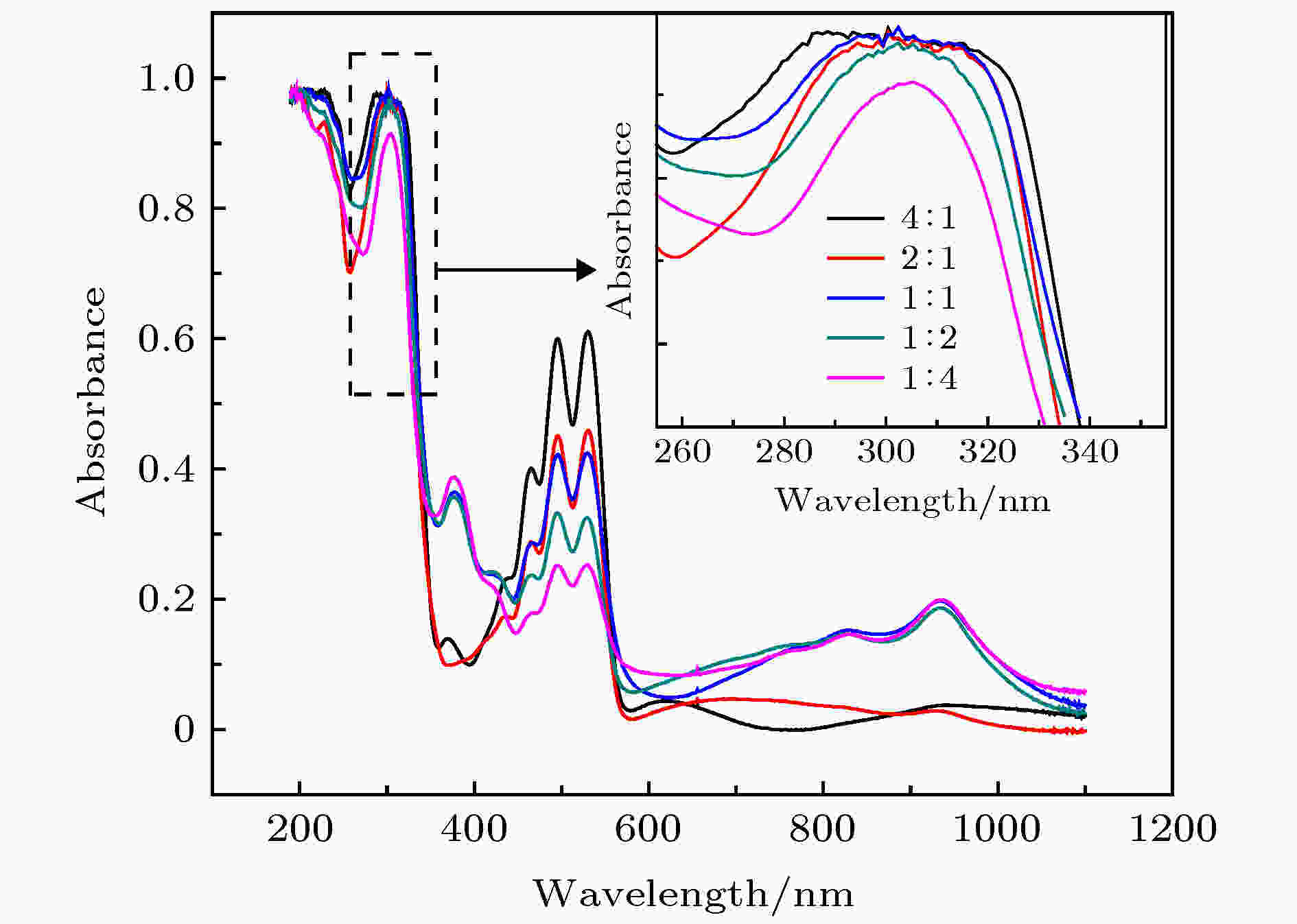
Figure6. The absorption spectra of Rubrene∶MoO3 films under different proportions
根据薄膜的AFM图像(图1)可见, 几种比例的薄膜表面均比较粗糙, 当光照射其表面时会出现漫反射, 薄膜表面的反射光强较弱, 因此可以通过朗伯比尔定律(Beer-Lambert law)计算薄膜的吸收系数, 其计算过程由为
根据(1)式, 吸收系数的误差主要源于透射光强和薄膜的厚度, 而影响透射光强和厚度的因素有混合薄膜的均匀性、平整度等. 在镀膜过程中, 首先在不去掉衬底挡板的前提下进行预蒸镀, 以去除蒸发源表面的杂质分子, 同时保证蒸发速率的稳定性. 待蒸发源的蒸发速率稳定后去掉衬底挡板正式蒸镀. 蒸镀过程中蒸发速率非常缓慢, 这样最大程度上保证了混合薄膜的均匀性, 降低了由于混合薄膜不均匀造成的误差. 通过图1发现, 虽然不同比例的混合薄膜的表面平整度不尽相同, 但观察每个AFM图的scale bar发现, 多数比例薄膜的表面起伏均在几个nm (当Rubrene∶MoO3等于1∶4时的表面起伏在14 nm左右), 这些起伏相对于薄膜的平均厚度(200 nm)而言可以忽略. 综上, 在计算薄膜吸收系数的过程中近似认为薄膜是均匀的.
利用半导体材料的经验公式Tauc公式进行线性拟合计算光学带隙. 运用吸收系数和光子能量的函数关系式为













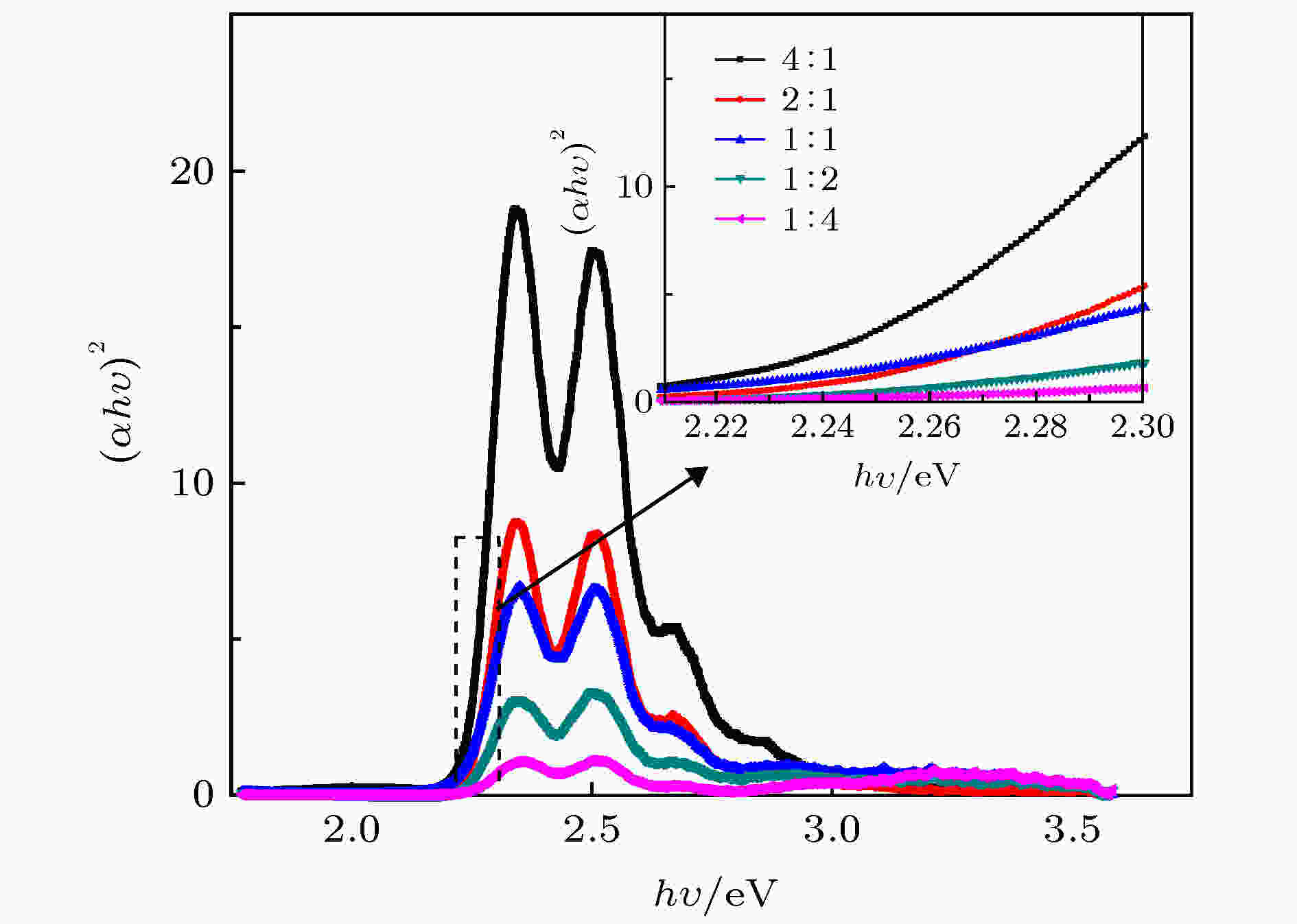


Figure7.


Rubrene∶MoO3 | 4∶1 | 2∶1 | 1∶1 | 1∶2 | 1∶4 |
能隙Eg/eV | 2.24 | 2.23 | 2.25 | 2.25 | 2.25 |
表1利用图7关系所得能隙值
Table1.The value of energy gap received from Fig. 7.
分析表格中的能隙值发现, rubrene∶MoO3混合比为2∶1时的能隙值最小, 而在偏离它的两个方向上, 能隙均较大. 因此, 当混合比例为2∶1时, 薄膜中的电子更容易激发, 而且从图1可知, 此种比例下的薄膜表面平整度也是最好的.
本文研究了4∶1, 2∶1, 1∶1, 1∶2, 1∶4五种比例的混合薄膜的电学性质, 图8是测试混合薄膜电学性质的器件结构示意图. 图9是五种比例下混合薄膜的J-V特性.
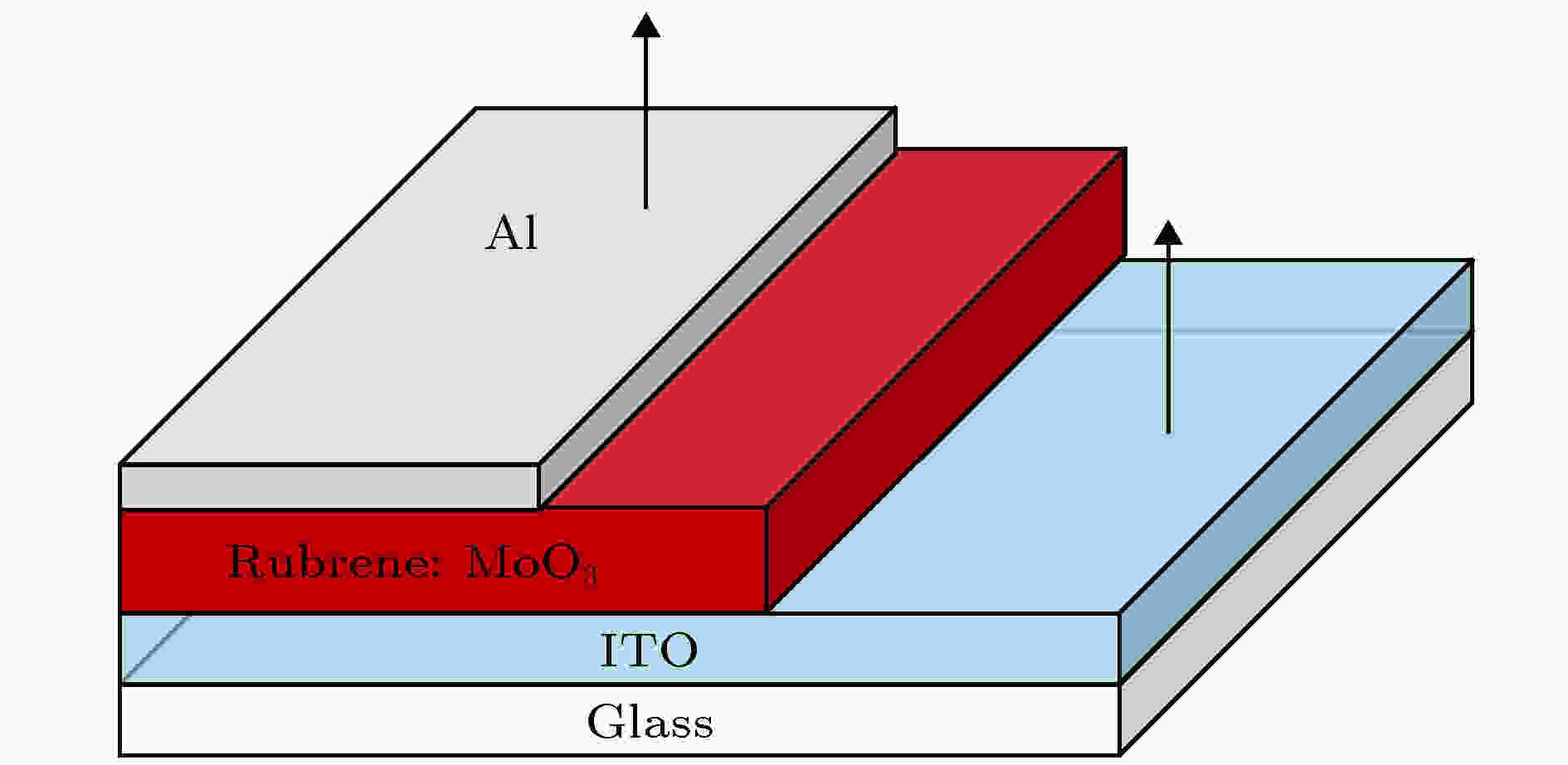
Figure8. Schematic illustration of the Al/rubrene∶MoO3/ITO (0.8 cm × 0.8 cm)
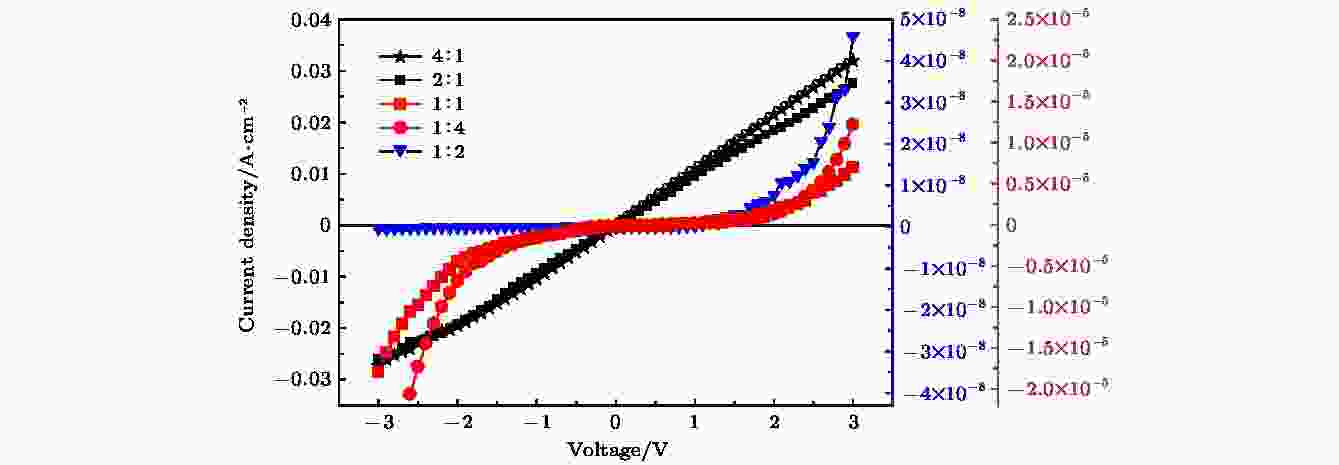
Figure9. Current density-voltage characteristics of rubrene∶MoO3 films under different proportion at room temperature
从图9中可以看出, 当混合比例为1∶1, 1∶2, 1∶4时, 混合薄膜与金属电极的接触表现为肖特基接触, 主要是因为MoO3能带较宽, 导电性能比较差. 当MoO3在薄膜中混合比例过高时, MoO3的性质占据主导地位, 过量的MoO3使得混合薄膜中载流子浓度下降, 显示出绝缘属性; 当器件两端的电压增大时, MoO3的外层电子将获得更多的能量, 实现从价带到导带的跃迁, 更多的电子成为自由电子, 从而使混合薄膜表现出导电性. 从图9中还可以看出, rubrene∶MoO3为1∶1时的电流比1∶2时的电流大, 分析其原因, 从混合薄膜的表面粗糙度(图2)来看, rubrene与MoO3的比例为1∶1时的表面较1∶2时更加平整, 因此与金属电极的接触更加良好. 当混合比例较小(如4∶1, 2∶1)时, 少量的MoO3能够诱导rubrene产生中间能级, 形成电荷转移络合物, 使混合薄膜的导电性能增强, 从而使混合薄膜与金属电极的接触从肖特基接触过渡到欧姆接触. 结合图1发现, 在本文所研究的几种混合比例下, 当混合薄膜的比例较小时, 薄膜表面的平整度也比较好, 表面平整度较好的薄膜的电学接触也更好, 这一特征对于改善有机发光二极管、有机薄膜晶体管等有机光电器件的电极与缓冲层接触提供了帮助. 混合薄膜表面平整度对金属电极的电学接触的影响将在接下来的工作中进一步研究.
通过对rubrene∶MoO3混合薄膜的光学性质研究发现: 在所制备的五种比例的混合薄膜中, 混合比例为2∶1时薄膜的PL谱峰波长最长, 吸收谱的能隙最小, 薄膜中的电子更容易被激发.
通过对rubrene∶MoO3混合薄膜的电学性质研究发现: 在所制备的五种比例的混合薄膜中, 混合比例为4∶1和2∶1的薄膜与金属电极的接触为欧姆接触, 增加了薄膜的导电性能, 为改善有机光电器件的电极与缓冲层的接触提供了帮助. 结合AFM图像, 在所制备的几种比例的混合薄膜中, 当混合比例为2∶1时, 薄膜表面具有最佳的平整度, 同时具有最优的光学和电学性质.