HTML
--> --> -->The dynamic conditions, viz. lower-tropospheric relative vorticity, weak vertical wind shear and Coriolis force required to generate initial torque and thermodynamic conditions such as ocean thermal energy, enhanced mid-tropospheric relative humidity (RH) and conditional instability, are necessary for the formation of a TC (Gray, 1968, 1979). However, these conditions are not always sufficient for the formation of a TC (Emanuel, 2003; Krishnamohan et al., 2012; Sumesh and Ramesh Kumar, 2013). The variability of TC frequency may be linked to the changes in the dynamic and thermodynamic conditions of both the atmosphere and the ocean. Various studies have been carried out in different ocean basins about the decadal variability of TCs. The sea surface temperature (SST) and atmospheric profiles of temperature and humidity together determine the maximum potential intensity (MPI) of the TC. Bister and Emanuel (2002) observed that thermodynamic potential intensity is the main causative factor for the seasonal and decadal variability of TC intensity and frequency with respect to their location and season.
It is well established that a minimum value of SST (> 26.5°C) is necessary for cyclogenesis. Wang et al. (2013) found that interdecadal changes in the TC count over the South China Sea are related with the increase in SST of the tropical Indian Ocean. In the ocean surface energy balance, the latent heat flux (LHF) and sensible heat flux (SHF) play a major role in the cyclogenesis and its further intensification process (Grodsky et al., 2009; Zeng and Wang, 2009; Ma et al., 2015). LHF is primarily linked to the change in SST, humidity at the surface and ocean surface wind speed (Liu and Curry, 2006; Yu et al., 2007). Zhou et al. (2015) showed that in the West North Pacific the change in sea?air humidity difference makes a direct contribution to surface LHF anomalies, and this difference in sea?air humidity contributes to the cyclogenesis during the warm basic state. Chen et al. (2014) found that the changes in LHF are critical in the intensification stage of TCs, depending on their traveling pathways. Almanza et al. (2012) found that LHF and SHF played a major role in the intensification of TC Nargis. These results show that LHF and SHF are the main determinants of cyclogenesis and its further intensification.
Environmental conditions like El Ni?o?Southern Oscillation (ENSO), Pacific Decadal Oscillation (PDO), Madden?Julian Oscillation (MJO) and the Indian Ocean Dipole (IOD), play major roles in the variability of TCs. Chan (2007) found that interdecadal variability of TC landfall in the Philippines is related to the different phases of ENSO and PDO. Over the NIO, a substantial proportion (60%) of TCs form in association with favorable phases of intraseasonal oscillation (ISO) events. Kikuchi and Wang (2010) found that over 70% of ISO-related genesis is associated with the northward-propagating boreal summer ISO mode, and up to 30% with the eastward-propagating MJO mode. Pattanaik (2005), Chan (2009) and Sebastian and Behera (2015) analyzed oceanic as well as atmospheric parameters during high- and low-frequency periods of TC formation and found that the main cause of the variability in TC activity is the variability of large-scale atmospheric circulation, rather than SST. In a recent study, Jangir et al. (2016) showed that the TC heat potential (TCHP) alone is not a significant oceanic parameter responsible for intensification and propagation of TCs.
During El Ni?o events, TC frequency varies over most of the ocean basins and in most cases the events suppress the formation of TCs. Ng and Chan (2012) found that the interannual variation of TC activity over the BOB during October?December can be attributed to the variation in the atmospheric dynamic and thermodynamic conditions that are largely forced by ENSO’s effect. Many model studies have been carried out recently in relation to TCs and SST over different ocean basins (e.g., Zhang et al., 2015, 2019; Strazzo et al., 2016). Zhang et al. (2019) proposed that a strong association exists between tropical Atlantic SST and cyclone frequency over the ARB. They found that reduced vertical wind shear is linked to Atlantic SST warming through a Matsuno?Gill response by favoring anomalous upper-level westerlies and lower-level easterlies. Zhao et al. (2018) studied the interdecadal change of the lagged relationship between TC frequency and local SST over the western North Pacific (WNP). They found an interdecadal change in the lagged interannual relationship between large-scale factors affecting TCs and local SST over the WNP. During 1998?2014, the previous-year local SST shows a significant negative correlation with the mid-level moisture and a significant positive correlation with the vertical wind shear over the main development region of WNP TC genesis. Almost opposite relationships are seen during 1979?1997, with a smaller magnitude of the correlation coefficients. These changes are consistent with the changes of the lagged interannual relationship between upper- and lower-level winds and local SST over the WNP. Due to the small sample size of TCs over the NIO during the pre-monsoon season, we do not carry out a correlation analysis between TC frequency and individual parameters. However, our analysis does confirm the existence of epochal changes in TC frequency that can be attributed to wind shear, mid-level thermal instability (MTI) and SST.
Zhao and Wang (2019) proposed that, due to a regime shift in Pacific climate state, a stronger interannual relationship between ENSO and TCs can be found since 1998, which is closely associated with the change of Central Pacific SST commonly known as El Ni?o Modoki events. It enhances the associations of environmental factors, including vertical wind shear and mid-level RH, with TCs, and thus increases the interannual relationship between ENSO and TCs, thereby influencing the WNP storm genesis and track. Using global coupled models, Jiang et al. (2018) suggested that the predictability of TC genesis is closely linked to the model predictability of several large-scale dynamic and thermodynamic fields. Change-point detection analysis of accumulated cyclonic energy (ACE) carried out by Balaji et al. (2018) revealed that there is a shift in ACE in recent decades. Specifically, ACE started increasing from 1997, and the observed twofold increase in ACE was due to the increase in the number and duration of very severe cyclonic storms in the satellite era (1981?2014). They also pointed out a longitudinal eastward shift of 2.3° in the mean genesis location of intense cyclones of the BOB during the same period. Rajeevan et al. (2013) suggested that epochal variation in the intensity of TCs over the ARB is associated with the epochal variation of vertical wind shear and TC heat potential. Evan and Camargo (2011) studied the climatology of the variation in cyclone frequency and its relationship with large-scale environmental variables over the ARB. The uniqueness of the present study is that we examine all individual thermodynamic and dynamic parameters along with Genesis Potential Index (GPI) and MPI to understand the role of each parameter on the distinct frequency variation of TCs over the ARB and BOB. Although Ng and Chan (2012) studied the variability of TCs during the post-monsoon season, they did not explain the reason for the pre-monsoon TC variability owing to the small sample size. Hence, there is a need exist to understand the epochal variability in TC frequency over the NIO region during the pre-monsoon season. In this study, we analyze the individual influences of both dynamic and thermodynamic factors responsible for the TC variability as well as the combined influence of the most favorable thermodynamic and dynamic parameters.
This paper examines the role of dynamic and thermodynamic factors on the epochal variation in TC frequency. An attempt is also made to explain the observed variability in cyclogenesis and its link to the vertical thermal structure of the atmosphere during different epochs. Following this introduction, section 2 explains the data and methods used, the results are presented and discussed in section 3, and conclusions are drawn in section 4.
The SST data for the NIO region are from the UK Met Office Hadley Centre’s Ice and SST dataset, version 1, known as HadISST1. The SST data are available from 1871 with a grid resolution of 1° × 1° (Rayner et al., 2003). In the present study, we use the SST data from 1955 onwards for the ARB and BOB.
In the present study, our focus is mainly on the frequency of cyclones formed during the onset phases of the study period extending for 60 years from 1955. For better understanding and comparison, the study period 1955?2014 is divided into three epochs of 20 years each based on the epochal variation of the IOD Mode Index (DMI), which exhibits a multidecadal variability. In order to understand the role of Indian Ocean warming on the epochal variability of cyclones, the epochs have been selected based on the pre-monsoon DMI, which is a relative measure of Indian Ocean warming between the east and west. The oscillation of SST between the western equatorial Indian Ocean (10°S?10°N, 50°?70°E) and southeastern equatorial Indian Ocean (10°S?0°, 90°?110°E) is used to define the IOD. The anomalous SST gradient is usually represented as the difference in SST between western and eastern boxes and denoted as the DMI. Positive values of the DMI represent the positive phase of the IOD, and vice versa (Saji et al., 1999). This unique air?sea interaction process is independent of ENSO and has inherent ability to alter the circulation pattern over the Indian Ocean. Several studies have demonstrated the influence of the IOD on the frequency of cyclones (Saji and Yamagata, 2003; Singh, 2008). This variation of the DMI is used to classify the study period into three epochs under the observed Indian ocean warming (e.g., Barnett et al., 2005; Levitus et al., 2005; Alory and Meyers, 2009; Rao et al., 2012; Chakravorty et al., 2014; Ratna et al., 2016). The time series of DMI reveals a clear pattern of a 20-year average positive IOD, in which the DMI is found to be increasing from 1955 to 1974, an average negative IOD with decreasing DMI from 1975 to 1994, and an average near-neutral to positive IOD period with a slight increasing DMI from 1995 to 2014 (Fig. 1).

Our analysis mainly revolves around many established cyclogenesis potential parameters as used in Gray (1968), such as the convergence at 850 hPa, mid-tropospheric (850?500 hPa) RH, temperature difference between 850 and 500 hPa, and vector wind shear between 200 hPa and 850 hPa. Out of these four parameters, only the vector wind shear is inversely proportional to the cyclonic activity, and the other factors are directly related (Gray, 1979). The frequencies of cyclones (wind speed > 34 knots) over both the ARB and BOB are considered for the present analysis. The formation area is defined based on the 60-year climatology of the pre-monsoon cyclone genesis location. For the time series and trend analysis, cyclogenesis potential parameters are averaged within boxes centered over the ARB (7°?17°N, 58°?73°E) and BOB (5°?17°N, 82°?95°E).
We try to analyze the well-accepted GPI developed by Emanuel and Nolan (2004). This index describes a nonlinear multiplication of four major parameters that favor TC genesis, and is given by the formula
where η is the absolute vorticity at 850 hPa (in units of s?1), RH is the relative humidity at 700 hPa (in units of %), Vshear denotes the magnitude of vertical wind shear between 850 and 200 hPa, and Vpot represents the MPI of the TC (both in units of m s?1). The MPI defined by Bister and Emanuel (2002) has the following formula:
where Ck and CD represent the exchange coefficients associated with enthalpy and drag, respectively; Ts is the SST; and To is the mean outflow temperature. The quantity CAPE* represents the saturation convective available potential energy (CAPE) at the radius of maximum wind, while CAPEb denotes the value of CAPE at the ambient boundary layer. It is noted that, for the same amount of SST perturbation, the size of Vpot is determined mainly by (CAPE* ? CAPEb), whose magnitude tends to be larger in a warmer and/or moister atmosphere (Bister and Emanuel, 2002). Camargo et al. (2007) used GPI for validating the TC genesis in climate model simulations. They found that the ability of the climate models in simulating the storms depends on the structure, dynamics and thermodynamics of the model simulated storms, rather than the large-scale environment for storm genesis, and thus each model exhibited a unique relationship between GPI and TC frequency. They further concluded that the correlation between the interannual anomalies of GPI and the number of TCs in a given basin also differs significantly from one model to the next. For consistency, the SST data are re-gridded into a 2.5° × 2.5° horizontal resolution prior to the calculation.
3.1. Epochal variation of TCs over the BOB and ARB
Figures 2a and b present a boxplot analysis of the frequency of TCs formed around the pre-onset and onset phases of the monsoon during the different epochs, viz. 1955?74 (early epoch), 1975?94 (middle epoch) and 1995?2014 (recent epoch), over the ARB and BOB respectively. In general, the number of cyclones over the ARB is lower compared to that over the BOB. As seen from Fig. 2a, the cyclonic activity over the ARB is suppressed in the middle epoch as compared to the early epoch, and then revived in the recent epoch. An absence of years with more than one cyclone is found during the middle epoch, whereas there are years with more than one cyclone in the early and recent epochs.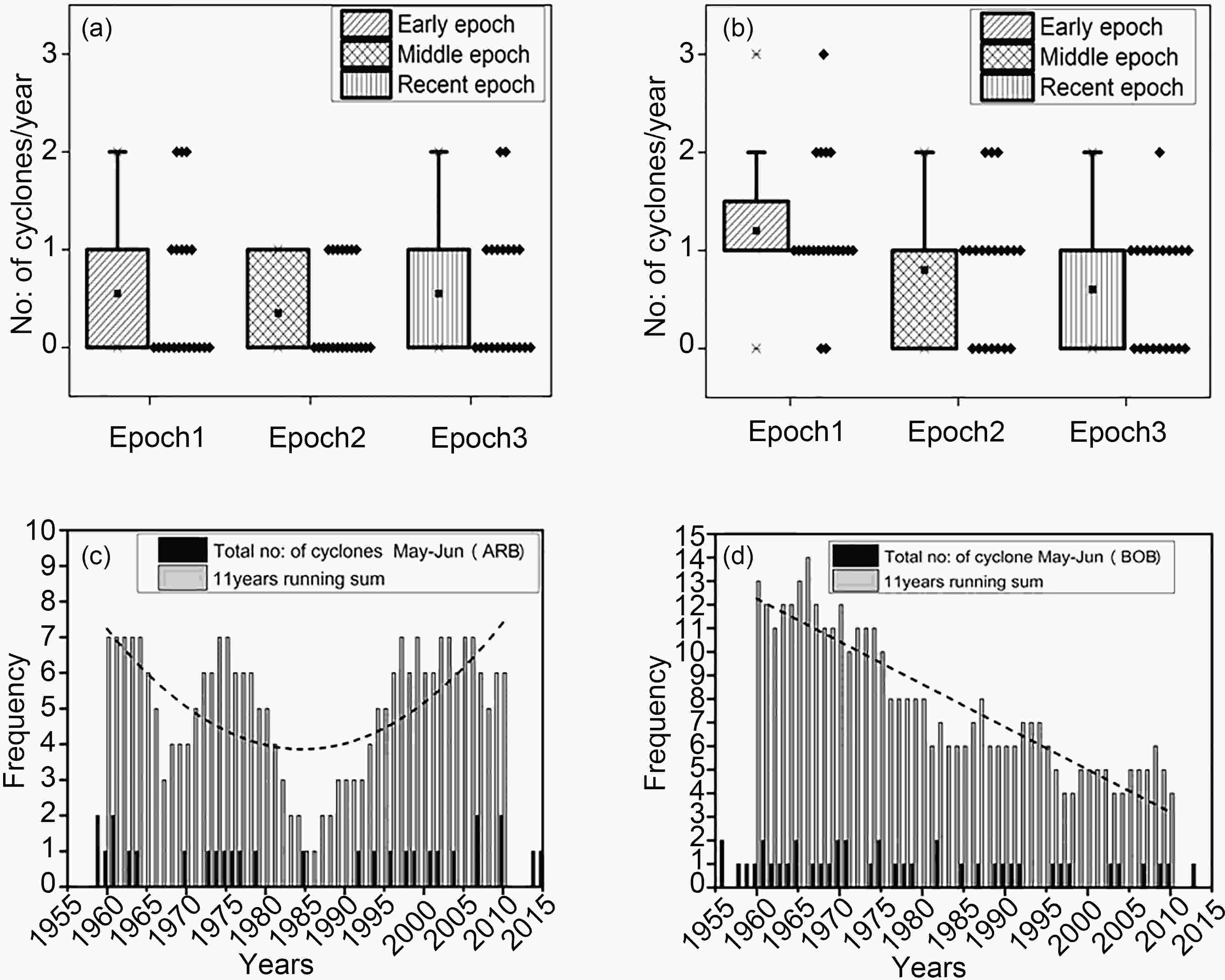
It is evident from the boxplots that the frequency of TCs decreases from the early to the recent epoch over the BOB (Fig. 2b). This is consistent with the findings of Ng and Chan (2012) and Rajeevan et al. (2013). The epoch-wise statistics of the cyclones over the BOB and ARB can be found in Tables 1a and b. The number of years without cyclones increases from two in the early epoch to seven in the middle epoch and nine in the recent epoch. Whereas, the number of years with a single cyclone decreases from 13 in the early epoch to 10 in both the middle and recent epochs. Similarly, years with two cyclones gradually decrease from four in the early epoch to three in the middle epoch and one in the recent epoch. Interestingly, a year with three cyclones is found only in the early epoch.
(a) Arabian Sea | |||||
Epoch | Years with 0 cyclones | Years with 1 cyclone | Years with 2 cyclones | Years with 3 cyclones | Total number of cyclones |
1955?74 (early) | 12 | 5 | 3 | 0 | 11 |
1975?94 (middle) | 13 | 7 | 0 | 0 | 7 |
1995?2014 (recent) | 11 | 7 | 2 | 0 | 11 |
(b) Bay of Bengal | |||||
Epoch | Years with 0 cyclones | Years with 1 cyclone | Years with 2 cyclones | Years with 3 cyclones | Total number of cyclones |
1955?74 (early) | 2 | 13 | 4 | 1 | 24 |
1975?94 (middle) | 7 | 10 | 3 | 0 | 16 |
1995?2014 (recent) | 9 | 10 | 1 | 0 | 12 |
Table1. Frequency of cyclones in each epoch in the (a) Arabian Sea and (b) Bay of Bengal.
Unlike in the BOB, there is no single incidence of three cyclones in a year in the ARB. The variability of each individual cyclogenesis parameter is evaluated to understand the observed epochal changes in the frequency of TCs over the BOB and ARB. In order to delineate the characteristics of the epochal variability in the frequency of cyclones in both basins, we have constructed an 11-year running sum of the number of cyclones formed during the pre-onset and onset phases of the monsoon from 1955 to 2015 (Figs. 2c and d). From Fig. 2c it is clear that the ARB exhibits epochal variation in the frequency of cyclones. On the other hand, Fig. 2d shows that cyclones in the BOB decrease throughout the epochs. The variation of individual cyclogenesis parameters and their relationships with the epochal variability of cyclone frequency is evaluated in the following section.
2
3.2. Lower-level convergence and upper-level divergence
Lower-level convergence at 850 hPa is identified as one of the influential parameters for cyclogenesis and is used as an input to the formulation of many cyclogenesis potential parameters (Zehr, 1992; Evan and Camargo, 2011). The converging air rises, and the water vapor within cools and eventually condenses into cloud droplets and rain droplets. As lower-level convergence is generally associated with the formation of low-pressure systems, time series plots of area-averaged convergence at 850 hPa over both the ARB and BOB for the period 1955?2014 are shown in Figs. 3a and b respectively. Even though the values of convergence in the BOB are higher than in the ARB, it decreases throughout the epochs; whereas, over the ARB it decreases during the early epoch and reaches a minimum value in the middle epoch before then increasing in the recent epoch. An interesting feature observed over the ARB is that the magnitude of convergence is positive in the early and recent epochs and negative during the middle epoch. The negative (positive) value of large-scale lower-level convergence generally suppresses (enhances) the formation of low-pressure systems. The minimum value of convergence observed during the middle epoch also supports the minimum in the cyclone number over the ARB during the same period. In the BOB, as the value is positive and decreasing during the early and middle epoch, the variation in the magnitude of convergence over the BOB is less as compared to the ARB. Convergence, though, is slightly higher in the recent epoch than the middle epoch. During the middle epoch, there is not much variability seen in the TC count over the BOB. However, there is a decreasing trend in the TC count over the BOB from the early to the recent epoch (Fig. 2d). As per the cyclogenesis formation mechanism, the upper-level divergence needs to be evaluated along with the lower-level convergence to link with the cyclone frequency.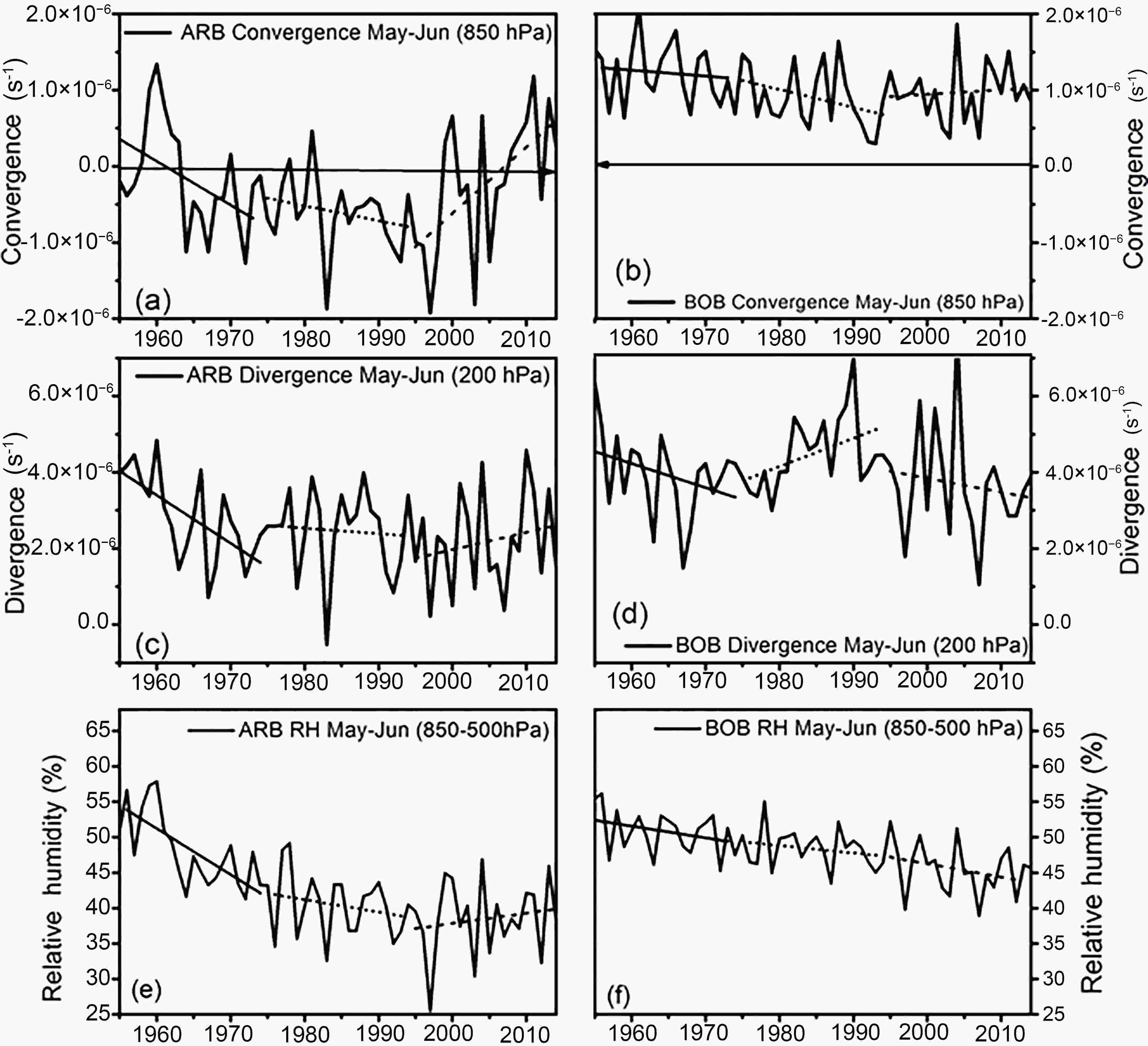
Upper-level divergence at 200 hPa is generally considered as one of the chief contributing factors enhancing the lower-level convergence. The role of upper-level divergence in cyclogenesis has been discussed by many authors. Using Atmospheric Model Intercomparison Project ensembles, Boyle (1994) established good correspondence between seasonally averaged upper-level divergence and mean sea level cyclone frequency. It was also remarked that the divergence at 200 hPa has maximum variability over the tropical Indian Ocean and the location of the center of convergence and divergence can be a useful tool for intercomparison of global atmospheric simulations. Upper-level anticyclonic circulation associated with upper-level divergence is more pronounced in developing systems. Developing systems have an upper-level anticyclone at the 200-hPa level, whereas it is absent for non-developing storms (Erickson and Gray, 1977). Time series of area-averaged divergence at 200 hPa for the three epochs over the ARB and BOB are presented in Figs. 3c and d respectively. Along with lower-level convergence, divergence at 200 hPa decreases during the early epoch over the ARB; whereas, in the middle epoch, convergence decreases and divergence becomes steady. In the recent epoch, both convergence and divergence increase over the ARB. The increase in convergence along with the increase in divergence favors storm development and its further intensification. The decrease in convergence retards the growth of systems in the middle epoch with a steady divergence. In the BOB, convergence values in the early epoch are steady and divergence decreases slightly. In the middle epoch, convergence decreases and divergence increases. During the recent epoch, convergence becomes steady and divergence decreases. The higher value of background convergence in the BOB in general helps the formation of more systems in the BOB compared to the ARB. In the recent epoch, steady convergence along with low values of divergence inhibit the formation of systems as well as their intensification over the BOB. This also supports the general decreasing trend in the frequency of cyclones over the BOB throughout the epochs.
2
3.3. Humidity
Mid-tropospheric humidity (MRH) is one of the key factors determining the genesis and further intensification and decay of cyclonic storms. Humidity contributes mainly to the positive feedback mechanism associated with CISK (Conditional Instability of the Second Kind) and favors the formation and further organization of low-pressure systems (Gray, 1968). High MRH also plays an important role in limiting the dilution process associated with entrainment, and hence is considered to be one of the main determining factors in cyclogenesis and its further development. Time series analysis of MRH (850?500 hPa) averaged over the formation area in the ARB (Fig. 3e) shows that MRH decreases in the early epoch, reaches a minimum in the middle epoch, and thereafter increases in the recent epoch. Whereas, in the BOB, MRH decreases (Fig. 3f) throughout the entire period. This trend in humidity also supports the observed frequency variation of TCs over the BOB and ARB.2
3.4. Wind shear
Wind shear plays an important role in cyclogenesis and its further intensification, and is known as one of main limiting factors for the intensification of storms. Hence, the role of wind shear in the epochal variability of cyclones must be evaluated in detail. The vector wind shear between 200 hPa and 850 hPa averaged over the formation area in the ARB (Fig. 3g) shows decreasing shear values in the early epoch and thereafter increasing values in the middle epoch and decreasing values again in the recent epoch. This shear pattern also backs up the epochal frequency variation of cyclones over the ARB in all three epochs. Whereas, in the BOB, the shear pattern shows a slight decreasing trend in all epochs (Fig. 3h). Notably, the shear values are higher and the trend is smaller over the BOB compared to the ARB.2
3.5. Thermal instability
In an unstable atmosphere, lifting of air parcels will take place and deep convective currents lead to thunderstorm development when enough moisture is present. Over warm ocean waters and within a region of the troposphere with enough lower-level convergence, such thunderstorm activity can grow in a much wider area and develop into a TC (Gray, 1979). Instability can be expressed using different indices; namely, the Lifted index, K index, total totals, Showalter index, amongst others. In the total totals index, the temperature difference between 850 and 500 hPa is the main deciding factor in the calculation. So, in this study also, we choose the temperature difference between 850 and 500 hPa as a measure of thermal instability.Stability of the atmosphere depends on the temperature profile, or in other words stability of the atmosphere depends on the environmental lapse rate (ELR). When the ELR is less than dry adiabatic lapse rate (DALR), the atmosphere is stratified and inhibits the rising motion of the air parcel. Apart from vertical wind shear (Lin and Chan, 2015; Murakami et al., 2011), the mid-level moisture content (Wang et al., 2010), tropical tropopause layer (Emanuel et al., 2013), and the environmental stratification (Shen et al., 2000; Hill and Lackmann, 2011; Tuleya et al., 2016; Kieu and Zhang, 2018) also play a major role in TC long-term intensity variations. Several model studies have reported that a more stable troposphere tends to be inimical to the development of deep convection, thereby limiting the growth of TC intensity. The thermal instability in the lower and middle atmosphere is analyzed in this section. The temperature difference between 1000 and 850 hPa represents the lower-level thermal instability (LTI), and that between 850 and 500 hPa represents the MTI. The time series of the LTI averaged over the cyclone formation area shows a decreasing trend throughout the epochs over the ARB (Fig. 3k). However, the LTI over the BOB increases in the early epoch and then decreases in the middle and recent epochs (Fig. 3l). The time series analysis of the LTI over the ARB and BOB shows that the difference decreases during the course of time, inferring that the lower troposphere is warmer than the surface (Figs. 3k and l). In general, the lower troposphere also warms in response to the surface warming over both the BOB and ARB during the middle and recent epochs. That is, the fractional increase in temperature or warming at 850 hPa is more than that at the surface. As a result, the lower atmosphere becomes more stable.
Interestingly, a steeper decrease in temperature difference is observed over the ARB than the BOB in the lower levels, implying the ARB is more stable than the BOB in the lower levels. The opposite scenario occurs in the middle level (850?500 hPa), where the temperature gradient increases up to the middle epoch and thereafter stabilizes before decreasing in the recent epoch over the BOB (Fig. 3j). On the other hand, the ARB shows similar variation in the mid-level instability up to the middle epoch, and then increases in the recent epoch. This increase in the MTI during the recent epoch also supports the observed increase in cyclones over the ARB during the recent epoch. As stability increases in the lower level, it requires a stronger lifting mechanism to generate any disturbance. Even though the low-level stability over the BOB is less than that over the ARB, the MTI controls the formation of clouds and hence lower-level disturbances. Although lower-level stability presents over the ARB, the increase in instability in the middle level compensates for the effect in the lower levels. Bhat (2006) reported the presence of lower-level thermal inversion over the ARB and presented analysis to confirm this. This may be another limiting factor for the development of cyclones and thus the lower number of cyclones in the ARB compared to the BOB.
To clarify further, we have similarly analyzed the CAPE and convective inhibition (CIN) over both the ARB and BOB (Figs. 3m and n). It is clear that the BOB features larger CAPE with low CIN and the ARB poses comparatively lower values of CAPE and large CIN. These results match with the variability of LTI and MTI as discussed earlier. Over the ARB, CAPE shows an increasing trend during the early and middle epoch and steady variability in the recent epoch. Over the BOB, CAPE shows a slight increasing trend during the early epoch and decreasing trend during the middle and recent epoch. This is in agreement with the trend in MTI as shown in Fig. 3j. The variability of CAPE and thermal instability parameters over the BOB support the observed decreasing trend in cyclones over the BOB during the onset phase of the monsoon. At the same time, CAPE increases during the early and middle epochs over the ARB and then becomes steady during the recent epoch. The CIN values decrease in the early epoch and then become steady during the middle and recent epochs over the BOB. This pattern also matches with the observed trend in lower-level instability. Interestingly, the ARB exhibits relatively higher CIN as compared to the BOB. It is apparent that CIN initially decreases in the early epoch, followed by an increase in the middle epoch. Then, CIN slightly decreases over the ARB and becomes steady during the recent epoch. This slight increase in CAPE along with the slight decrease in CIN also supports the observed frequency variation of cyclones over the ARB. This increase in integrated instability energy along with the increase in MTI in the recent epoch also confirms the increase in the cyclone number during the same period over the ARB, as compared to the BOB, close to the onset phase of the monsoon.
2
3.6. Vertical temperature profile
The physical properties of the surface vary substantially according to location and this produces strong horizontal variations in near-surface temperature. On monthly and longer time scales, variability in surface irregularities are smoothed out to some extent by atmospheric circulation and the distribution of temperature in the troposphere usually differs from that at surface. Therefore, temperature variations with height vary according to location in response to vertical air motion controlled by large-scale circulation and determine the vertical temperature distribution. We also make an attempt to analyze this epochal variability in the temperature profile and its link to TC frequency.The vertical profiles of temperature anomalies averaged over the cyclonic formation area of the ARB and BOB during each epoch are presented in Figs. 4a and b respectively. The early and recent epoch are a mirror image and the middle epoch shows little variation from the normal pattern. It is evident that not only the surface temperatures, but the temperatures of the entire troposphere, have increased in recent decades as compared to the early epoch, with the middle epoch being the transition period with little or no change in temperatures except in the lower and upper levels. A gradual increase in the mid-tropospheric temperature (between 3 and 5 km) is observed from the early to the recent epoch over the BOB. However, the mid-tropospheric temperature over the ARB increases from the early to the middle epoch, and a slight decrease in temperature is evident in the recent epoch, as compared to that over the BOB. As a result, the middle troposphere is slightly stable over the ARB during the middle epoch and this might have also contributed to the reduction in the frequency of cyclones during the middle epoch over the ARB as compared to the BOB. This pattern is consistent with the time series of the temperature difference plots presented in Figs. 3i and j. This increase in stability in the lower levels combined with warming in the middle troposphere also supports the reduction in the number of cyclones during the middle epoch over the ARB. This implies that instability in the middle layers during the recent epoch is more than that of the middle epoch. This factor also supports the observed increase of TCs in the recent epoch over the ARB. The analysis of vertical profiles of temperature is consistent with the time series analysis of thermal instability in the middle layer as discussed in the previous sections.

2
3.7. Spatial variability of dynamic and thermodynamic genesis potential parameters
Many studies have reported that, during El Ni?o and La Ni?a periods, cyclones follow a particular track compared to that in normal years. That is, in a warming climate, the cyclone frequency, track, intensity and structure also change with the background climate state. Figure 5 presents the tracks of cyclones in all three epochs. We have compared the IMD tracks with the Joint Typhoon Warning Center (JTWC) for the available period and found that the number and tracks of TCs between these two datasets correspond well. The storm track from the IMD e-atlas and that from the JTWC are presented in Fig. 5. Though the storm classification of the IMD and JTWC differ slightly, the storm tracks are in good agreement between the two independent datasets. Since our analysis is not dealing with the day-to-day intensity variation and track changes, small differences in the datasets may not influence the analysis much. In the BOB, though the cyclone numbers decrease throughout the epochs, their general track remains the same. Most of the time, the cyclones are moving towards the head bay or making landfall over the eastern BOB around the Bangladesh?Myanmar coast. However, northward- and westward-moving cyclones are present over the ARB during the early epoch. Westward-moving cyclones are comparatively reduced during the middle and recent epochs, with more tendencies of re-curvature tracks in the recent epoch. The cyclogenesis region shifts towards the Indian west coast during the recent epoch, which is a matter of grave concern as far as Indian west coastal states are concerned.
Unlike the Pacific and Atlantic Ocean basins, the TC index or cyclone frequency (TCI) is much lower over the North Indian Ocean. If we consider the pre-monsoon season of the ARB and BOB separately, there are 12 non-cyclone years over the ARB in the early epoch, 13 in the middle epoch, and 11 in the recent epoch. The non-cyclone years in the BOB, meanwhile, are two in the early epoch, seven in the middle epoch, and nine in the recent epoch. Hence, obtaining significant correlations with TCI and different cyclogenesis parameters epoch-wise is difficult. However, we make an attempt to evaluate the spatial distribution of cyclogenesis parameters during each epoch separately. Hence, for explaining the temporal and spatial variability in the TC over the ARB and BOB, analysis of the spatial variation of thermodynamic and dynamic potential parameters is also found to be necessary. We have analyzed the surface thermodynamic and air?sea turbulent fluxes to obtain reliable background climate signals that elucidate a reasonable link to the frequency variation in TCs over the NIO.
2
3.8. Spatial variability of cyclogenesis potential parameters over the ARB
The spatial variations of the epochal anomalies of LTI and MTI presented in Figs. 6a and b are consistent with the time series of area-averaged values discussed earlier. The epochal differences significant at the 99% confidence level using the t-test are highlighted as hatched areas in the spatial figure. As discussed in terms of the results presented in Fig. 3k, due to the warming of the entire lower levels in the atmosphere, the LTI decreases from the early to the recent epoch over the ARB. However, as seen from Fig. 3i, the MTI increases from the early to recent epoch in the ARB. The increase in MTI over the western ARB is more pronounced in the recent epoch and this spatial variability is consistent with the time series of epochal variability discussed in relation to Fig. 3. The epochal difference in LTI and MTI over the ARB are mostly significant at the 99% level during the early and middle epoch.
The anomalies of the spatial variation of LHF over the ARB exhibit (Fig. 6c) positive values during the early epoch, whereas negative anomalies dominate during the middle epoch. However, the western ARB is characterized by positive anomalies of LHF during the recent epoch. The difference in LHF over the ARB is mostly significant at the 99% confidence level during the three epochs. The spatial variability of SHF (Fig. 6d) is in-phase with that of LHF, and these together explain the observed frequency variation of TCs. It is apparent from Fig. 6e that SST increases over the ARB from the early to the recent epoch. This basin-wide warming of SST over the ARB contributes largely to the increasing frequency of severe TCs in the recent epoch. These patterns are consistent with the variability in LHF and SHF. The co-evolution of surface LHF, SHF and SST is directly linked to the warming basic state (Li et al., 2011). It may be concluded that ocean warming and associated modulation in the surface-level turbulent fluxes create necessary thermodynamically favorable background atmospheric and oceanic states over which cyclogenesis takes place.
The epochal anomalies of the spatial variation of dynamical parameters such as lower-level convergence, upper-level divergence and wind shear are shown in Figs. 7a-c. The spatial variability of convergence (Fig. 7a) shows positive anomalies over the south and central ARB and negative anomalies over the north ARB during the early epoch. Over these two highlighted regions, the differences are significant at the 99% level. This is in agreement with the tracks of cyclones during the early epoch (Fig. 5). On the other hand, negative anomalies of convergence with 99% significance are seen over most of the ARB, which supports the minimal TC frequency during the middle epoch. Nevertheless, the ARB is characterized by significant (greater than 99% confidence level) positive anomalies of convergence during the recent epoch. This is supported by the observed epochal variability of cyclones over the ARB and the time series analysis of cyclogenesis parameters discussed in the previous sections.

Large positive upper-level divergence significant at the 99% level is seen over the southeast ARB during the early epoch (Fig. 7b). Significant (greater than 99% confidence level) negative anomalies of upper-level divergence are found over the central ARB during the middle epoch. The upper-level divergence over the central ARB again increases in the recent epoch, which is significant at the 99% level. The spatial distribution of divergence at 200 hPa during the three epochs (Fig. 7b) shows that most of the westward movement of the cyclones is following the positive divergence anomaly area (Fig. 5). In the recent epoch, the ARB tracks are northward or northwestward. The wind shear (Fig. 7c) exhibits positive anomalies in the early epoch over the north and south ARB and weak negative anomalies over the central ARB. The positive wind shear anomalies over the north and south ARB during the early epoch are significant at the 99% level. However, in the middle epoch, the wind shear increases in the central ARB and supports reduced cyclogenesis. During the recent epoch, the negative anomaly of wind shear becomes favorable over most of the ARB, which also supports both the spatial and temporal variation of cyclone tracks during the recent epoch. This result over the ARB is consistent with the findings of Rajeevan et al. (2013).
2
3.9. Spatial variability of cyclogenesis potential parameters over the BOB
The anomalies of LTI (Fig. 6a) over the BOB show that LTI decreases from the early to the recent epoch. As in the ARB, due to the warming of the entire lower levels in the atmosphere, the LTI decreases from the early to the recent epoch over the BOB. However, along the east coast and head bay, LTI shows positive anomalies during the recent epoch. The distribution of MTI over the BOB is different from that over the ARB, especially during the recent epoch. Most of the ARB shows positive MTI anomalies, but the head bay region exhibits negative anomalies. As discussed earlier, this increase in background MTI favors cyclogenesis in the ARB during the recent epoch as compared to the BOB. These epochal differences in LTI and MTI over the BOB are significant at the 99% level. The anomalies of the spatial variation of LHF over the BOB exhibit (Fig. 6c) positive values during the early epoch and negative anomalies in the middle epoch. During the recent epoch, positive anomalies of LHF present only over the south BOB. However, the central BOB exhibits negative anomalies. The spatial variability in SHF (Fig. 6d) is in-phase with that of LHF and these together explain the observed frequency variation of TCs. It is apparent from Fig. 6e that SST increases over the BOB from the early epoch to the recent epoch. However, the central BOB shows small negative anomalies of SST during the recent epoch.Lower-level convergence shows positive anomalies over the central and southwest BOB and negative anomalies over the eastern BOB along the costal boundary. The convergence over the central BOB further decreases during the middle epoch and increases over the eastern BOB in the recent epoch. However, weak negative convergence anomalies (less than 95% confidence level) are found over the BOB during the recent epoch. The upper-level divergence over the BOB during the early epoch shows significant negative anomalies over the southeast BOB and a small pool of positive anomalies over the head bay and southwest bay region. During the middle epoch, positive anomalies are found over the south BOB and negative anomalies over the head bay region. During the recent epoch, small negative anomalies of upper-level divergence are found over the southeast BOB and positive anomalies over the southeast and head bay region. A more northeastward track is prominent in the BOB during the recent epoch (Fig. 5), which is consistent with the positive upper-level divergence over the eastern BOB. Over the BOB, wind shear anomalies increase from negative to positive over the head bay region during the three epochs (Fig. 7c). The difference in shear values over the BOB are significant at the 99% level during all three epochs. It is also worth noting that the values of shear over the central ARB are lower than those over the central BOB.
The coherent evolution of lower-level convergence, upper-level divergence and wind shear corresponds well with the frequency and spatial variation of TCs over both the ARB and BOB. The epochal variability in the track is mostly determined by the dynamic parameters, such as wind shear and upper-level divergence. The upper-level divergence guided by the steering flow dictates the re-curving cyclone tracks.
Following Camargo et al. (2007), many studies have applied GPI in observations and models as a useful tool in analyzing the TC frequency variation and its association with different individual cyclogenesis parameters at different time scales. Jiang et al. (2012) studied the modulation of TC genesis over the eastern Pacific by intraseasonal variability (ISV) in a high-resolution atmospheric model. Considering the predictability of ISV at a lead time of 2?4 weeks, their analysis shows some optimism for predicting TCs on ISV time scales. It is found that, while relative roles of GPI factors are dependent on ISV phase and location, lower-level cyclonic vorticity, enhanced mid-level RH, and reduced vertical wind shear can all contribute to the observed active TC genesis over the Eastern Pacific during particular ISV phase. Zhao et al. (2015b) found that cyclogenesis is enhanced over the WNP during the active phase of MJO and aligns along the monsoon shear line. They further examined the GPI and its individual parameters in cyclogenesis on the intraseasonal time scale and found that more positive GPI anomalies are observed during the active phase of MJO. It was proposed that low-level vorticity and mid-level humidity played dominant roles in the enhanced cyclogenesis during the active MJO phase. In another study by Zhao et al. (2015a) it was proposed that the combined coherent influence of two leading ISV modes over the WNP, known as the 40?60-day MJO mode and 16-day biweekly mode, contribute to the majority of TC activity. They suggested that individual influential cyclogenesis parameters, such as low-level relative vorticity and mid-level RH, contribute to the favorable cyclogenesis in terms of positive GPI anomalies.
We have analyzed the role of each individual parameter included in the formulation of GPI. Individual GPI parameters such as thermal instability, mid-level humidity, SST, vorticity, convergence and wind shear are presented in Figs. 6 and 7. These parameters, with proper scaling, are used in the calculation of GPI. Further, the spatial variability of GPI during the three epochs is presented in Fig. 8 (left-hand panel). The MPI, which has not been presented before, is also included in Fig. 8 (right-hand panel). During the early epoch (Fig. 8a), the central, southwest and northeast ARB show positive GPI anomalies and the northwest ARB exhibits negative anomalies. The central BOB shows large positive anomalies of GPI during the early epoch. During the middle epoch (Fig. 8b), the GPI anomalies become negative over the ARB and the positive anomalies are reduced over the BOB. In the recent epoch (Fig. 8c), the western part of the ARB shows positive anomalies, and the anomalies over the central BOB become negative. This spatial variation in GPI is also consistent with the previous analysis and confirms the multidecadal variability in the thermodynamic and dynamic background state in modulating the TC frequency variation over the ARB and BOB. Instability and SSTs are invariably used for the calculation of GPI. Hence, MPI usually represents the combined effects of SST warming and epochal changes in mid-level instability and RH. The variabilities of these individual parameters have been discussed in previous sections. The spatial variability of the MPI (Figs. 8d and e) clearly shows the impact of sea surface warming from the early to recent epoch. As discussed earlier, the frequency variation of TCs over the NIO is not only linked to thermodynamic basic states, but the epochal changes in the spatial variability of wind shear and lower-level convergence also contribute.
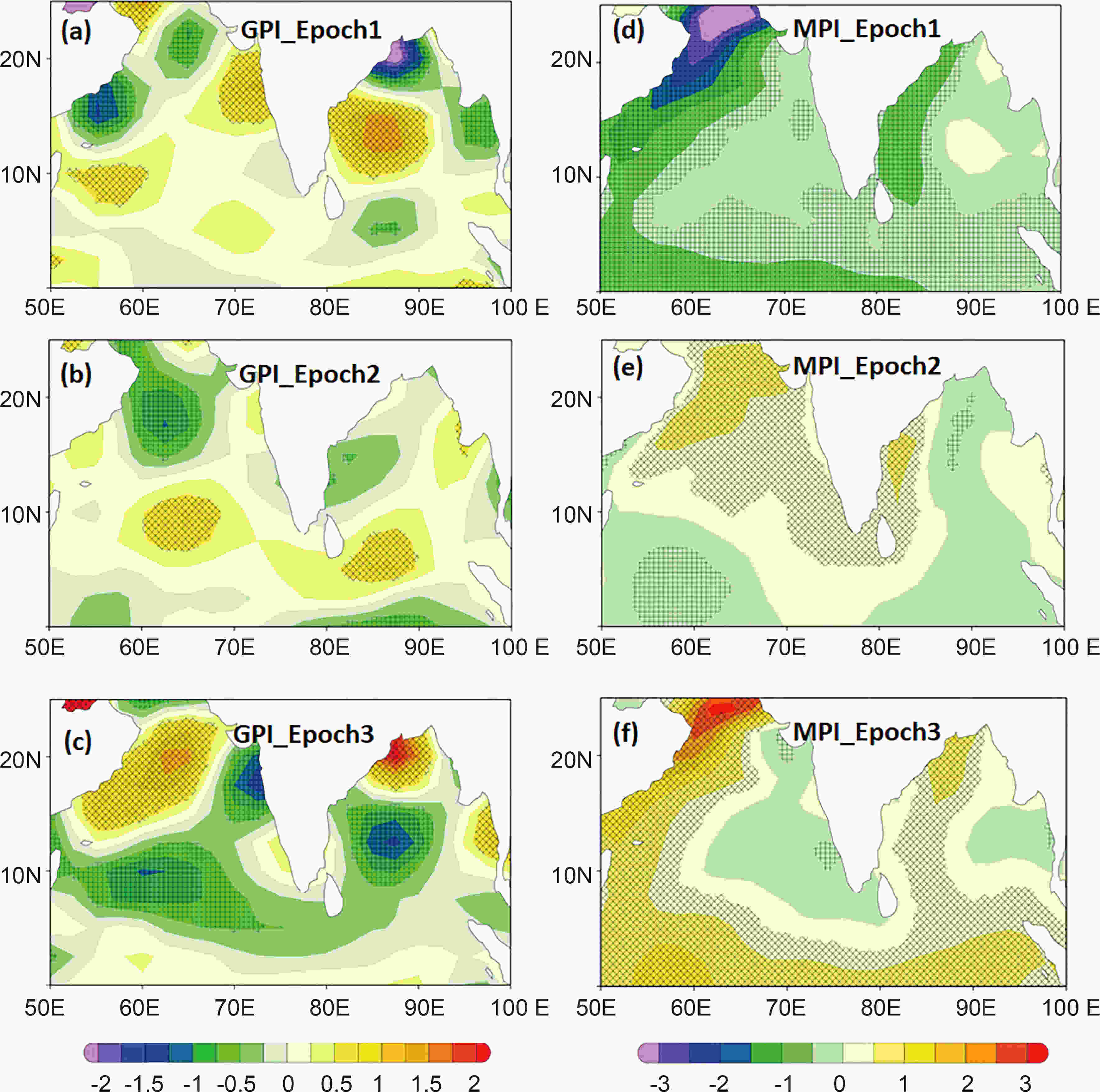
Along with positive anomalies of LHF and SHF, the convergence and LTI favor greater cyclogenesis over the BOB than the ARB in the early epoch. However, the negative anomalies of convergence and LTI are responsible for decreasing the cyclone frequency during the middle and recent epochs over the BOB. Due to the lack of favorable cyclogenesis potential parameters, the number of cyclones reduces in both the ARB and BOB in the middle epoch. In general, surface turbulent fluxes such as LHF and SHF, SST, lower-level convergence, upper-level divergence and wind shear favor cyclogenesis over the ARB during the recent epoch. Whereas, over the BOB, positive SST anomalies are seen only over the south BOB and weak negative anomalies are seen in the central and north BOB. Epochal variability is predominantly controlled by the balance between low-level convergence, upper-level divergence and stability in the lower atmosphere. As proposed by Sebastian and Behera (2015), the SST of the pre-monsoon period has a better correlation coefficient with the power dissipation index calculated for the NIO. Consistent with the findings of Li et al. (2011), the increase in SST may be the reason for the enhanced surface turbulent fluxes of LHF and SHF over the ARB. As can seen from Tables 1a and 1b, ARB cyclones increase and BOB ones decrease during the recent epoch around the onset phase of the monsoon in response to the warming basic climate state. Murakami et al. (2017) suggested that the recent increasing frequency of extremely severe cyclonic storms over the ARB during the post-monsoon season is due to anthropogenic influence rather than natural variability. The present analysis during the pre-onset and onset phases of the monsoon shows epochal variability over the ARB, and a consistent decreasing trend of TCI is observed over the BOB. The thermodynamic and dynamic basic states are entirely different over the ARB and BOB. This distinction in background state of the two ocean basins during the three epochs elucidates the individual roles played by each cyclogenesis parameter in the observed frequency variation of TCs. The role of the decrease in cyclone frequency over the BOB and increase in cyclone numbers over the ARB around the onset phase of the monsoon on the monsoon onset process and its further advancement during the early phase of the monsoon needs to be investigated further. The main finding of the study is that the ARB shows epochal variability in cyclone frequency; however, over the BOB, TC numbers decline throughout the three epochs. This frequency variation is consistent with the thermodynamic and dynamic background variability and is supported by the epochal variability in GPI. The distinct behaviors of the observed epochal variability of cyclones over the BOB and ARB are linked to the epochal decadal variability in the equatorial Indian Ocean SST and vertical variation of the thermal profiles during the three epochs in response to the warming of both atmosphere and ocean.
Acknowledgements. The authors are grateful to NCEP?NCAR, WHOI OAFlux, the UK Met Office Hadley Centre and the IMD for providing datasets used in this study. S. ABHILASH thanks UGC BSR for providing facilities and support. We thank C. S ABHIRAM for helping with analyzing the data.