HTML
--> --> -->The luminosity of the lightning channel is the result of the combined effect of the transition radiation of different particles in the channel between different energy levels. According to observation, the spectral types of the lightning channel at different stages are different. The attenuation curve of the spectra in the early stage is the same as the curve in the ascending stage, but the curve in the later stage exhibits a different characteristic (Orville, 1968). Therefore, the luminosity of the lightning channel cannot fully reflect the fine structure of lightning discharge.
The lightning spectrum is closely related to the physical processes that occur inside the lightning discharge channel. Each particle in the lightning channel has its own unique spectrum. Different physical processes can stimulate different optical radiation (Salanave, et al., 1962; Warner et al., 2011). The different spectral lines can reflect the states of particles in the lightning channel and the physical processes inside the lightning discharge channel (Liu et al., 2014).
Much effort has been made with respect to the lightning spectrum during the past hundreds of years. Most research has focused on the spectral characteristics and the change of the channel parameters (Salanave et al., 1962; Xue et al., 2015; Mu et al., 2016). Since there are no observational current data matching the corresponding electrical parameters, combined analysis of the spectral characteristics and lightning discharge current is rare. Recently, with the development and application of artificially triggered lightning, it is possible to study spectral characteristics and lightning discharge current characteristics together (Qie et al., 2011). It is an important way to reveal the lightning physics process based on the spectral characteristics in different discharge parameters, which reflect the fine-structure of lightning and the microphysical process of the internal lighting channel (Walker and Christian, 2017).
In this paper, the emission spectrum of artificially triggered lightning in Guangdong was studied using a slitless spectrometer. Based on the direct measurement data from the lightning current, different stages of the artificially triggered lightning channel spectrum were analyzed, the difference between the metal section and air section was discussed, and lastly, the relationship between the total spectral strength and the transferred charge and the current intensity were analyzed.


A slitless grating spectrograph was used for the spectral observation of the triggered lightning. The distance between the spectral observation point and the triggered lightning site was about 1.9 km. The recording system comprised PhotronSA5 and ultima APX-RS high-speed cameras. The temporal resolution were 6000 and 50000 frames per second. The spatial resolutions were 1024×512 and 512×272. The grating was in front of the camera lens; and the first spectral resolution level was 1.1 nm (Zhang et al., 2017).
3.1. Comparison of the air and metal section spectra of the initial continuous current
Figure 3 shows the lightning spectra of the initial continuous current process obtained on 15 June 2017 and 13 June 2016, separately. As can be seen from the figure, due to the gasification of metal wires, the lightning channel produced strong metal spectra, and the metal channel lasted much longer than the air channel. For example, the metal channel spectra on 15 June 2017 lasted about 140 ms, while the air channel lasted only 0.167 ms.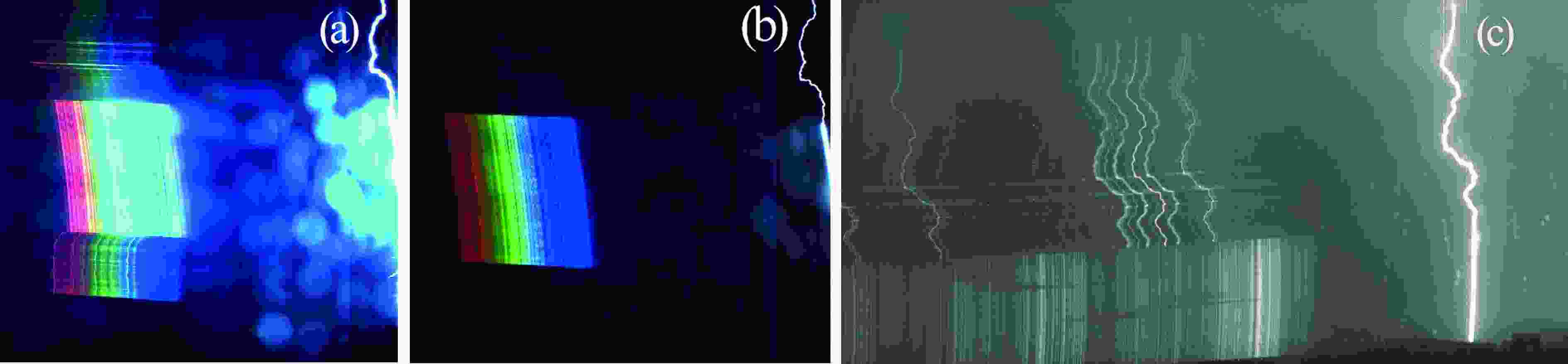
The current in the lightning channel can decompose and ionize the molecules and atoms in the lightning channel instantaneously. In the channel plasma, the particles of NI (netural nitrogen atom), NII (singly ionized nitrogen), OI (netural oxygen atom) and OII (singly ionized oxygen) are predominant. The upper excitation energy of some lines was higher than 30 ev, such as 30.12 ev at NII 517.9 nm, 34.23 ev at OII 425.3 nm, and 31.32 ev at OII 419.0 nm. At the same time, NIII (double ionized nitrogen) and OIII (double ionized nitrogen) ion lines with very short duration were observed in the lightning channel, which is less well reported (Salanave et al., 1962; Yuan et al., 2004; Zhang et al., 2010).
Table 1 shows the ionization energy of nitrogen, oxygen and iron. It can be seen that the ionization energy of FeI, FeII and FeIII was much lower than that of the nitrogen and oxygen of the same level. The ionization energy of FeIII was also lower than that of some spectral lines, such as NII517.9 nm and NII425.3 nm in the lightning channel. Therefore, in the case of the same current intensity, more particles were excited in the metal channel compared with the air channel, which can generate more spectral radiation. In other words, the luminosity of the metal channel in Fig. 3 was much stronger than that of the air segment, and the spectral intensity of the metal segment channel was also much stronger than that of the air segment. Similarly, in the subsequent dissipation phase of the plasma channel, the composite reaction time of the metal segment lightning channel was also longer; that is, the metal segment lasts longer than the air segment.
Excitation energy (ev) | N | O | Fe |
I | 14.53 | 13.62 | 7.902 |
II | 29.60 | 35.12 | 16.20 |
III | 47.44 | 54.94 | 30.65 |
Table1. Ionization energies of nitrogen, oxygen and iron (NIST, 2018).
2
3.2. Comparison of the air and metal section spectra of the return strokes
We obtained the spectra and current of the initial continuous current and the five return strokes (R1–R5) of the negative-polarity, artificially triggered lightning flash on 8 July 2017, as shown in Fig. 4. Meanwhile, Table 2 shows the peak current, 10%–90% rise time, half-peak time, transfer charge, stroke interval, and other parameters for the five return strokes. Due to the weak original spectrum in the continuous current phase, the luminosity of the picture was increased. The third hit back current was low, and the 500.5 nm, 568.0 nm etc. lines can be seen on the original spectrum. When converted to a relative intensity spectrum, it is difficult to distinguish, so there is no analysis of the third return stroke.
Parameter | R1 | R2 | R3 | R4 | R5 |
Peak current (kA) | 8.51 | 10.53 | 3.98 | 9.0 | 8.83 |
10%–90% rise time (μs) | 0.29 | 0.22 | 0.13 | 0.14 | 0.09 |
Half-peak time (μs) | 12.5 | 5.88 | 1.47 | 11.3 | 5.38 |
Charge transfer (C) | 0.46 | 0.53 | 0.18 | 0.42 | 0.38 |
Return interval (ms) | 0 | 34.07 | 13.19 | 18.04 | 21.71 |
Table2. Parameters of current for five return strokes.
Figure 5 shows the spectra of the air and metal sections of the lightning channel for the four strokes. We normalized the air and metal sections to the same coordinates. The horizontal coordinate was the wavelength, and the ordinate was the relative strength. An offset between the two spectra’s waveforms was set, which did not represent a comparison of the relative strength—just a clearer display.

In spectral analysis theory, the spectrum is used to identify the substance and determine its chemical composition. As seen in Fig. 5, the spectra of the air and metal section channels were similar. The line spectra on the continuous spectra were also similar. The line spectra for both the air and metal section were identical to the gas line, which did not contain metallic lines. It can also be seen from the original spectral photo that the line was continuous in the metal and the air section. The main reason was that, after the wire line was gasified by the initial continuous current, the metal particles in the metal section of the lightning channel had basically dissipated. Simultaneously, the spectral line from metal particles could be reduced. Because the continuous background spectrum of the lightning spectrum is stronger in the visible band, the metal spectrum may not be identified. By increasing the temporal resolution and reducing the distance of observation, we can perhaps observe some metal spectral lines; but, with an increase in the number of return strokes, the spectrum of the metal particles will be increasingly less.
The main difference between the spectrum of the air and the metal section was that there was considerable change in the intensity of some spectral lines. According to the intensity of the excitation energy of the spectral line, the spectrum could be divided into two kinds: one was greater than 30 ev (e.g., OII419.0 nm was 31.32 ev, NII 517.9 nm was 30.14 ev); and the other was less than 30 ev. Table 3 shows the spectral parameters of the triggered lightning channel. Comparing the spectral lines of the four return strokes, OII 419 nm was not observed in the air section, and the strength of NII 517.9 nm in the metal section was far greater than that in the air section. The variation of spectral lines less than 30 ev was not obvious. The main reason was that the metal channel had not completely disappeared when the return stroke began and was reheated by the return stroke current, which increases the temperature and density of the channel plasma. Therefore, the spectral lines with higher excitation energy are fully excited. Relative to the line intensity of OII 419.0 nm and NII 517.9 nm in the air section, it increased considerably. The reheating of the lightning channel also increased the brightness and duration of the lightning channel. Due to there being fewer metal particles in the return channel, the radiation of metal particles was a secondary factor. Further observations at close range are needed to increase the temporal resolution.
Lines (nm) | Transitions | weighted transition rates giAk | Excitation energy | |
Essentia llines | 463.0 | NII 2s22p3s–2s22p3p | 3.70×108 | 21.159914 |
480.3 | NII 2s22p3p–2s22p3d | 2.22×108 | 23.246032 | |
500.5 | NII 2s22p3p–2s22p3d | 1.03×109 | 23.141958 | |
568.0 | NII 2s22p3s–2s22p3p | 3.47×108 | 20.665516 | |
594.2 | NII 2s22p3p–2s22p3d | 3.83×108 | 23.246032 | |
648.2 | NII 2s22p3s–2s22p3p | 7.74×107 | 20.409132 | |
656.3 | Hα 3d 2D5/2–2p2P°3/2 | ? | 12.088 | |
High excitation energylines | 407.5 | OII 2p2(3P) 3d 4F9/2–2p2(3P) 3p4D°7/2 | 1.98×109 | 28.7060917 |
419.0 | OII 2p2(1D) 3d 2G9/2–2p2(1D) 3p2F°7/2 | 1.98×109 | 31.319435 | |
425.3 | OII 2p2(1D) 4f2H11/2–2p2(1D) 3d2G9/2 | ? | 34.233 | |
517.9 | NII 2s2p2(4P)3p–2s2p2(4P)3d | 1.18×109 | 30.138938 |
Table3. Spectral parameters of the triggered lightning channel.
The reheating of the metal section of the channel caused the brightness enhancement and duration extension of the metal channel, which correlated closely with the interval time and the current intensity of the return stroke.
2
3.3. Spectral characteristics of different current intensities
When the spectra of the four return strokes in the air and metal segments of the triggered lightning channel are compared (Fig. 6), it can be seen that the spectra of the first and fourth return strokes coincided. Because the second and fifth return strokes produced two spectra, the first spectrum with the weaker continuous radiation could be considered to be the early response of the return strokes.
Figure 7 shows the correlation between the total intensity of the spectrum and the amount of transfer charge and current peak. The total intensity of the spectrum is defined as the area surrounded by the spectral curve and the horizontal coordinate. The total intensity of the spectrum of the second and fifth return strokes was the sum of the two spectra. We can see that the total intensity of the spectrum had a certain linear relationship with the amount of transfer charge, and the relationship with the current intensity was unordered. The transfer charge is an integral reflection of the heat effect caused by the current. Combining the results of Figs. 6 and 7, we can conclude that the correlation between the total intensity of the spectra and transfer charge was higher than that of the current peak.
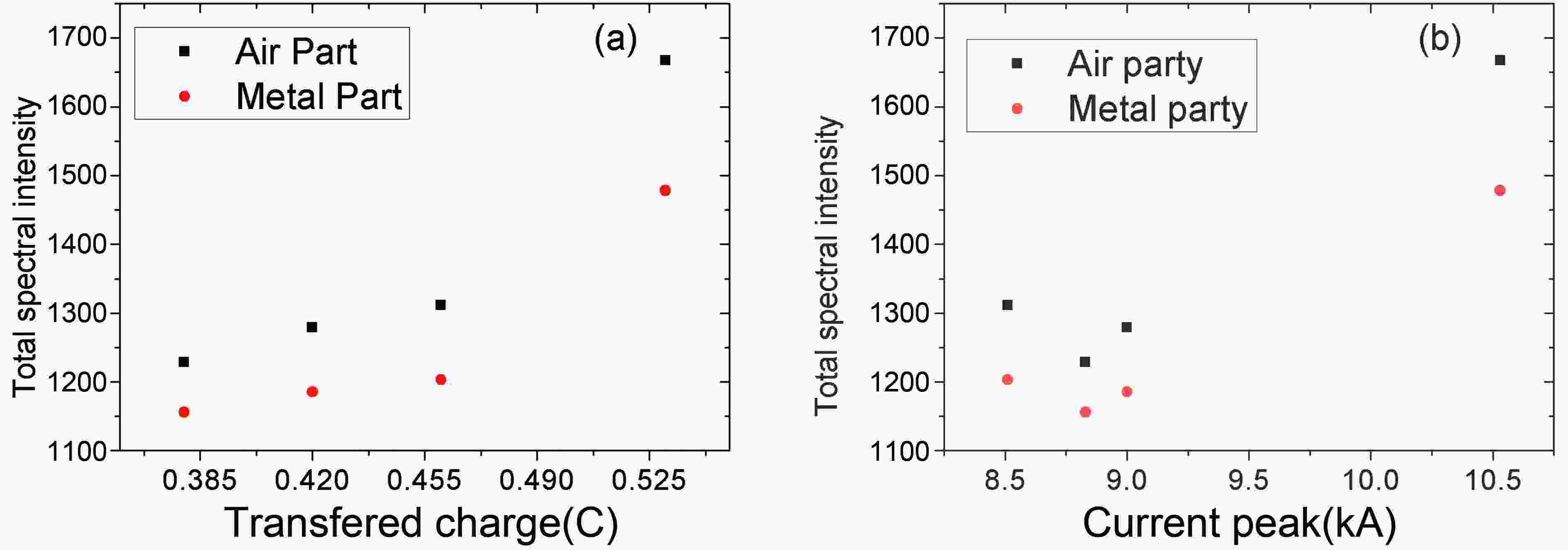
2
3.4. Variation of the total intensity of the spectrum with height
Figure 8 shows the total intensity of the spectra at different heights of the lightning channels for R1 and R4. Since the spectra of R2 and R5 were indistinguishable at multiple heights, the different height spectra of R2 and R5 are not given. It can be seen from Fig. 8 that the total intensity of the spectrum increased with an increase in height of the lightning channel, and the mutation in the curve corresponded to the winding of the lightning channel. Figures 8b and c show the stack of the lightning spectra at different heights of the two strokes.
2
3.5. Temporal evolution of spectral lines
Figure 9 shows the emission spectrum of the last two return channels of a negative-polarity artificially triggered lightning at 10 July 2017, with a temporal resolution of 50 000 frames per second. The spectrum of the lightning strike back channel changes over time in R9 (Fig. 9a) and R10 (Fig. 9b). The lightning channel is on the left.
The spectral lines can be divided into three categories according to duration. The line intensity of the first kind of spectral line reaches a peak within 20 μs, and then attenuates quickly. R9 disappeared within 40 μs, and R10 disappeared within 20 μs, e.g., OII 419.0 nm, OII425.3 nm, NII 444.7 nm, NII 480.3 nm, NII 500.5 nm, NII 517.9 nm, NII 568.0 nm, and NII 616.8 nm. These spectral lines are the transition spectrum of a single ionized ion of nitrogen oxides, and minimum excitation energy was 20.67 ev of NII 568.0 nm.
The difference between the second kind and the first kind of spectral line is the duration time, which was longer than the first one. For example, NII 463.0 nm in R9 lasted to 80 μs, and NII 594.2 nm in R9 lasted to 20 μs, and lasted to 100 μs in R10.The third kind of spectral line was not observed in 0 μs and reached a peak in 20 μs, and then slowly decreased. These spectral lines lasted for a long time, such as OI436.8 nm, OI615.8 nm 600.8 nm, NI 648.2 nm, Hα, Hβ. The strongest line was Hβ. This kind of spectral line is the transition spectral line of neutral nitrogen, oxygen and hydrogen atoms, with low excitation energy between 12 and 13 ev.
(1) The number density of particles of the metal channel was higher than that of the air section after gasification in the initial continuous current phase. Also, the ionization energy, excitation energy and dissociation energy of Fe were much lower than those of nitrogen and oxygen. Thus, there were more excited particles in the metal than in the air channel, which led to the lightning channel generating more radiation, a brighter channel, and stronger spectral intensities. Moreover, the duration of the recombination processes of the metal channel was also longer in the subsequent phase of dissipating the plasma channel.
(2) The reheating of the residual wire channel by the return pulse current increased the temperature and density of the channel plasma, which caused the line with higher excitation energy to be fully excited, resulting in enhanced brightness of the wire channel.
(3) The correlation between the total spectral intensity and transfer charge was higher than the peak current of the return strokes. The total intensity of the spectra increased with the height of the lightning channel.
(4) The line spectra could be divided into three types according to their duration, and the high excitation energy appeared firstly and then attenuated rapidly. At the later time, the weaker current existed in the channel persistently, and the ion recombination processes resulted in more neutral particles at the excited states. The third kind of spectral line could last for a long time, e.g., OI, NI, Hα, Hβ, and so on.
Acknowledgments. This study was supported by the Open Fund of the State Key Laboratory of Severe Weather of the Chinese Academy of Meteorological Sciences (Grant No. 2019LASW-B08), and as a Basic Research Project of the Chinese Academy of Meteorological Sciences (Grant No. 2015Z006.2016Z005).