

Nitrogen Cycling in the Crop-Soil Continuum in Response to Elevated Atmospheric CO2 Concentration and Temperature -A Review
ZHANG JinYuan

通讯作者:
责任编辑: 李云霞
收稿日期:2020-06-24接受日期:2020-07-29网络出版日期:2021-04-16
基金资助: |
Received:2020-06-24Accepted:2020-07-29Online:2021-04-16
作者简介 About authors
张锦源,E-mail:

摘要
关键词:
Abstract
Keywords:
PDF (1664KB)元数据多维度评价相关文章导出EndNote|Ris|Bibtex收藏本文
本文引用格式
张锦源, 李彦生, 于镇华, 谢志煌, 刘俊杰, 王光华, 刘晓冰, 吴俊江, Stephen J Herbert, 金剑. 作物-土壤氮循环对大气CO2浓度和温度升高响应的研究进展. 中国农业科学, 2021, 54(8): 1684-1701 doi:10.3864/j.issn.0578-1752.2021.08.009
ZHANG JinYuan, LI YanSheng, YU ZhenHua, XIE ZhiHuang, LIU JunJie, WANG GuangHua, LIU XiaoBing, WU JunJiang, Stephen J HERBERT, JIN Jian.
开放科学(资源服务)标识码(OSID):
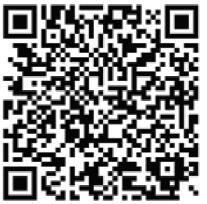
自工业革命以来,煤炭、石油等化石燃料的过度使用和大规模砍伐森林等原因导致了全球气候变化,其中,大气CO2浓度和温度升高是全球气候变化中两个最主要的特征[1]。截止到目前,大气中的CO2浓度已经从1800年的不足280 μmol·mol-1上升到了410 μmol·mol-1。预计到21世纪中叶,大气CO2浓度将达到550 μmol·mol-1,全球平均气温也将升高2℃左右[2]。
大气CO2浓度和温度升高对全球范围内作物养分利用和生产力产生了深刻影响,这已引起了国内外科学家的普遍关注。氮素作为作物生长发育过程中所必需的营养元素之一,是作物从土壤中吸收量最大的矿质元素[3]。而且,氮素也是作物体内蛋白质、叶绿体、核酸以及生长激素的重要组成元素之一,在作物生命活动中具有重要地位,因此氮素具有“生命元素”之称[4]。所以,国内外****开展了有关大气CO2浓度或温度升高对氮循环影响的研究,但是研究者往往把CO2浓度和温度两个因素独立研究,而忽视了CO2浓度和温度同时升高对氮素循环的交互影响。
文中综述了CO2和温度升高对作物氮素吸收和分配,以及与氮有效性密切相关的土壤氮转化的影响,并系统总结了以往研究中二者对作物-土壤氮循环过程产生的交互作用,为科学合理地预测大气CO2浓度和温度升高条件下,农田生态系统中作物氮素需求,以及保障农作物产量稳定供应提供理论依据,对于全面认识全球气候变化背景下的农田生态系统氮素循环及土壤可持续利用具有重要意义。
1 作物氮吸收
氮吸收与作物生产力之间存在着密切联系,大气CO2浓度和温度升高对作物氮吸收的影响主要表现在作物的需氮量,根系形态,蒸腾作用以及豆科作物的根瘤固氮作用的变化等方面。1.1 作物的需氮量与氮吸收
作物的需氮量是指作物从土壤、肥料、空气等外界环境中吸收氮,用于满足作物生长和同化合成新组织所需求的氮量。作物的需氮量主要受其地上部生物量的调节。CO2浓度升高能够使作物地上部的生物量加大[5],长势增高,不断产生新的组织,增加作物对氮的需求(表1)。CO2作为植物光合作用的底物,在其浓度升高时为作物提供了更加充足的光合反应原料。据统计,高CO2浓度能够使C3作物的光合速率提高10%—50%,C4作物的提高幅度小于10% [6]。增幅的差异与C3和C4作物具有不同的光合路径有关。但总的来说,光合作用的增强有利于作物叶片非结构性碳水化合物含量和生物量增加[7],从而提高了作物的需氮量。****利用位于北京昌平的FACE(Free-Air CO2 Enrichment,开放式大气CO2浓度升高)平台研究发现,在550 μmol·mol-1CO2浓度下,大豆的生物量增加17%,其地上部的氮吸收总量显著增加25%[8];通过江苏无锡的FACE平台研究发现,当CO2浓度升高200 μmol·mol-1时,水稻地上部氮素的吸收量比正常CO2浓度下增加了16%[9]。由此也表明,高CO2浓度下作物吸氮量的变化幅度受作物类型影响,不同作物的氮吸收对CO2浓度升高的响应具有差异性。Table 1
表1
表1大气CO2浓度与温度升高下作物氮浓度、氮吸收及生物量变化
Table 1
作物类型 Crop species | CO2浓度/温度处理 CO2 concentration/temperature | 氮浓度 N concentration | 氮吸收 N uptake | 生物量 Biomass | 文献 Reference |
---|---|---|---|---|---|
大豆Soybean | 390/550 μmol·mol-1 | ↓ | ↑ | ↑ | [33] |
大豆Soybean | 390/550 μmol·mol-1 | ↓ | [41] | ||
大豆Soybean | 415/550 μmol·mol-1 | ↓ | ↑ | ↑ | [8] |
水稻Rice | 380/680 μmol·mol-1 | ↓ | ↑ | ↑ | [13] |
水稻Rice | 500 μmol·mol-1 | ↓ | ↑ | ↓ | [12] |
水稻Rice | 390/550 μmol·mol-1 | ↓ | ↑ | ↑ | [51] |
水稻Rice | 370/550 μmol·mol-1 | ↓ | ↑ | [39] | |
水稻Rice | +200 μmol·mol-1 | ↓ | ↑ | [11] | |
小麦Wheat | 390/550 μmol·mol-1 | — | ↑ | [53] | |
小麦Wheat | 360/550 μmol·mol-1 | ↓ | [40] | ||
水稻Rice | 500 μmol·mol-1;+2℃ | ↑ | ↓ | ↓ | [12] |
水稻Rice | 380/680 μmol·mol-1;22/32℃ | — | ↑ | ↑ | [13] |
水稻Rice | +60 μmol·mol-1;+2℃ | ↑ | ↓ | [35] | |
水稻Rice | +60 μmol·mol-1;+2℃ | ↑ | ↑ | [37] | |
水稻Rice | 390/550 μmol·mol-1;+2℃ | ↓ | ↑ | ↑ | [51] |
水稻Rice | +200 μmol·mol-1;+1℃ | ↓ | ↓ | [11] |
新窗口打开|下载CSV
升温通过影响作物的生长发育和生理特性进而影响作物的氮吸收。光合作用对温度升高非常敏感,在环境温度达到作物光合的最适温度之前,温度升高促进光合作用,例如,昼夜温度在30℃/22℃以下时,棉花的光合速率随着温度的升高而上升[10]。当环境温度超过作物的最适温度时,作物净光合速率随着温度的升高而降低,这主要是由于高温能够诱导光系统II活性中心转化为无活性中心,叶绿体的结构发生改变,引起叶绿体的降解,因此,光合作用受到抑制。而且,升温能够缩短作物的生长发育时间,并增强作物的呼吸消耗,使得作物的生物量和产量下降,最终导致作物需氮量降低[11]。通过江苏省常熟市的FACE平台研究发现,增温2℃导致水稻氮吸收总量降低12.3%[12]。然而,由于不同研究中温度升高的幅度和升温持续时间不尽一致,导致结果变异性较大。例如CHENG等[13]研究发现,当夜间温度增加10℃时,水稻的氮吸收总量显著提高2.2%。分析原因是由于夜晚温度较低,在此时间段升温促进了作物的生长发育,而且作物在夜间不进行光合作用,避免了高温对光合作用的胁迫,因此夜间升温可能会促进作物对氮的吸收。
在大气CO2浓度和温度同时升高条件下,作物的需氮量发生变化。首先,作物合成有机物质的最重要途径是光合作用,有研究发现,高温和高浓度CO2对于作物光合速率的影响相互独立,并无交互作用[14],但也有统计发现,随着大气CO2浓度升高,作物光合作用的最适温度会提高5—10℃[15],所以在一定范围内升高CO2浓度和温度对作物光合速率存在协同促进作用。不同研究中二者交互作用的差异与二者升高的幅度和不同作物对CO2和温度的适应阈值有关。其次,对于作物的需氮量,有****认为,温度升高对高CO2下作物需氮量的升高效应没有显著影响,表明作物无论处在低温还是高温条件下,升高CO2浓度均能够增加作物的需氮量,且增加的幅度间无显著差异,反映出二者对作物需氮量没有互作效应[16]。但另一种观点则认为,虽然高温导致作物生物量和需氮量降低,但是在此基础上升高CO2浓度能够缩小作物需氮量的降低幅度,表明CO2浓度升高有利于减轻高温对氮素吸收产生的抑制作用。例如研究发现,温度高于37℃抑制水稻生长并导致水稻不育,降低水稻干物质量和氮吸收量,然而,在此基础上,将大气CO2浓度上升200 μmol·mol-1时,水稻的吸氮量降低幅度显著小于单独升温条件下的降低幅度[11]。从另一个角度来说,二者的交互作用表现为在高温条件下,高CO2浓度对作物需氮量的促进作用被减弱,即升温抑制了高CO2浓度对水稻氮吸收的促进作用。例如CHENG等[13]研究发现,温度为22℃时,升高CO2浓度使水稻吸氮量增加了12.7%;而当温度升至32℃时,高CO2浓度下的水稻吸氮量仅仅增加了2.2%。由此可见,温度和CO2浓度作为两个重要的环境因子,二者对作物的生长和需氮量会产生明显的互作效应,这与单独升高CO2浓度或温度下作物氮素吸收和利用的变化具有显著的差异。
1.2 作物根系形态与氮吸收
根系作为作物吸收土壤无机氮的器官,其在土壤中的空间分布以及与土壤的接触面积均对氮素吸收起关键作用[17]。研究表明,不同玉米品种的产量不同,其主要原因是其根系长度和根系表面积的差异导致了其吸氮量的不同[18];有****认为氮素水平提高之所以能提高作物的氮素吸收能力,是由于其促进了作物根系表面积显著增加[19],因此,根系的生长发育和形态对于作物氮素吸收具有重要意义。CO2浓度升高可以通过改变作物根系形态影响作物氮素吸收,大气CO2浓度升高可以促进作物根系的生长发育,扩大根系在土壤中的分布,从而增加对氮素的吸收。如利用位于瑞士苏黎世的FACE平台研究发现,C3作物根系的生物量平均增加47%[20];CO2浓度升高可以使作物直径小于1 mm细根生物量增加63%,直径1—3 mm的根生物量增加37%,粗根生物量增加16%[21]。****通过位于江苏江都的稻麦轮作FACE平台也发现,在水稻的各个生育时期,提高200 μmol·mol-1大气CO2浓度能够显著增加水稻的总根长和根尖数[22],从而促进水稻对土壤中可利用氮素的截获和吸收;而且CO2升高能够促进小麦、高粱和大豆根系分支的生长[23],增加根系侧根长度和根表面积[24],因此使作物根系在土壤中具有更强的穿透力以及与土壤更大的接触面积,有利于作物高效吸收土壤中的氮素养分。CO2浓度改变根系形态的作用机理可能与作物体内激素水平的变化有关。例如,CO2浓度升高能够显著促进番茄根毛的形成和生长,是由于高CO2浓度条件下,番茄体内的吲哚乙酸以及乙烯的释放量显著升高[25]。有关高CO2条件下根系形态变化与根系氮吸收效率的量化关系还需要从根系的空间分布,不同直径根系的比例变化以及根活力等方面深入研究,以探讨作物可能通过改变氮利用效率而提高对气候变化适应能力的机制。
温度对作物根系形态产生影响。WEI等对8个品种的油菜研究发现,将昼夜温度从23℃/17℃上升到27℃/24℃时,高温导致了油菜总根长下降11.9%,比表面积降低12.2%,根体积下降10.6%[26]。然而,升温的程度与根系生长和氮吸收的关系还应从不同作物种类对温度升高的敏感程度方面进行比较研究。然而,有关温度和CO2浓度同时升高对作物根系形态结构的影响鲜有报道。二者对作物氮吸收的根系影响机制值得深入研究。
1.3 作物蒸腾作用与氮吸收
蒸腾作用是作物体内的水分以气态方式从植物表面向外界散失的过程,直接驱动着作物根系吸收和运输水分。作物根系吸收氮素主要是消耗代谢能的主动吸收过程,虽然根系吸氮并不依赖于作物的蒸腾作用,但作物有效的蒸腾作用能够造成根际土壤与原土体之间出现明显的水势差,从而促进土壤溶液中的NO3-,NH4+离子随着水流向根表迁移,在一定程度上为作物根系的氮吸收提供了有利条件[27]。CO2浓度升高导致作物叶片气孔张开度缩小,部分气孔关闭,降低气孔导度,从而导致单位叶面积蒸腾强度下降[28]。KANG等[29]研究发现,在高大气CO2浓度(700 μmol·mol-1)条件下,小麦和棉花的叶面气孔导度均下降,蒸腾作用均显著降低,从而可能抑制了作物的氮吸收。在一定温度范围内,升温有利于增加作物叶片的气孔导度,提高水分子从细胞表面蒸发和通过气孔的扩散速率,最终增强了作物的蒸腾作用,但是当温度升高到超过适合作物生长的阈值范围,则会对作物造成胁迫,使作物的气孔导度减小,蒸腾作用降低。不同植物类型的适合生长的环境温度阈值不同,例如水稻、小麦和玉米的最适温度范围一般分别为30—32、20—22和30—32℃,其最高温度阈值范围分别为36—38、30—32和40—44℃[30],而且同种类型作物的不同品种对温度的敏感性也可能不同,这些因素均导致高温或高浓度CO2条件下作物生理活动影响氮吸收的差异性。1.4 根瘤固氮与氮吸收
豆科作物的共生固氮能力是影响其吸收氮以及生产力的较大因素。研究表明,CO2浓度升高能够增加豆科作物如白羽扇豆、白三叶、豌豆和大豆的根瘤重量和数量[31],提高固氮酶的活性[32];例如LI等[33]利用OTC(Open Top Chamber,开顶式生长室)对东北8个大豆典型品种研究发现,高CO2浓度(550 μmol·mol-1)显著增加了大豆生殖生长期的根瘤数,可以使根瘤数从正常CO2浓度(390 μmol·mol-1)下的平均每株79个增加到每株113个,从而增强了大豆生殖生长期共生固氮的能力,使大豆成熟期地上部氮吸收总量平均增加41%。单独升高温度对豆科植物的生物固氮影响鲜有报道,仅有的一些研究发现,在高CO2浓度(560 μmol·mol-1)下升温1.5℃时,大豆植株氮中来源于根瘤固氮的比例降低[34],但由于大豆吸氮总量增加,因此,尽管来源根瘤固氮的比例会降低,但是其固氮量可能仍会增加。然而,目前同时考虑CO2和温度升高交互作用对豆科作物固氮的影响还缺乏足够有说服力的证据,还有待进一步探索研究。1.5 作物不同生育时期和熟期与氮吸收
作物的氮吸收总量是由作物不同生育时期的养分吸收特征所决定。CO2和温度升高对氮吸收的影响因生育时期发生变化,从而会影响二者的互作效应。例如,在水稻生育早期,特别是分蘖期,大气CO2浓度升高60 μmol·mol-1并同时升温2℃导致水稻全株氮素含量增加10.8%,说明在水稻营养生殖阶段,二者会促进水稻植株对土壤氮素的吸收,但是在分蘖期以后,水稻的全氮含量无显著变化[35],说明分蘖期后CO2浓度和温度对氮吸收没有产生正向互作。此外,也有研究发现,增温幅度,特别是作物关键生育时期增温幅度的差异,以及施氮量等因素会对CO2和温度的互作效应产生影响[36]。不同熟期的作物氮吸收对CO2和温度同时升高的响应也不同。例如,王斌等[37]研究发现,早稻在单独增温2℃条件下氮吸收量降低2.8%,在单独CO2升高60 μmol·mol-1条件下氮吸收量增加15.7%,而同时升高CO2浓度和温度时,早稻氮吸收量增加5.1%;然而,对于晚稻,在单独增温2℃条件下氮吸收量增加7.3%,在单独CO2升高60 μmol·mol-1条件下氮吸收量增加10.2%,但二者同时升高时,晚稻氮吸收量增加了15.0%,因此说明了CO2和温度升高对早稻氮吸收产生反向的互作效应,对晚稻则表现为正向的互作效应。
2 作物氮浓度
2.1 大气CO2浓度升高与作物氮浓度
作物氮浓度,特别是作物的叶片氮浓度,是决定作物光合作用和生产力的重要生理指标之一[38],因此研究大气CO2浓度升高条件下作物氮浓度的变化具有重要意义。大量研究表明,大气CO2浓度升高降低了作物氮浓度(表1),例如在高CO2浓度(500 μmol·mol-1)下,水稻叶片氮浓度在拔节、开花和成熟3 个生育时期分别比对照降低了26.0%、14.9% 和22.0%,穗的氮浓度降低了0.6%[12];YANG等[39]通过位于江苏无锡的FACE平台对水稻的研究也发现,高于环境CO2浓度200 μmol·mol-1显著降低了水稻地上部的氮浓度,在分蘖早期、分蘖中期、穗分化期、孕穗期、抽穗期和籽粒成熟期分别降低了1.8%、6.1%、12.2%、14.3%、12.1%和6.9%,其中叶片氮浓度降低最为显著;SINCLAIR等[40]通过位于美国亚利桑那州的FACE平台研究发现,CO2浓度升高200 μmol·mol-1导致禾本科C3作物叶片氮浓度平均降低9%。对于豆科作物,LI等[41]利用OTC对24个大豆品种研究发现,在CO2升高条件下,成熟期大豆根系、茎秆和籽粒平均氮浓度分别降低24%、25%和6%。COTRUFO等[42]通过对关于不同作物在大气CO2升高条件(CO2浓度从450 μmol·mol-1到1500 μmol·mol-1不等,其中约一半为700 μmol·mol-1)的78个研究中的378个作物氮浓度的数据进行了meta分析,发现不同作物的各个器官的氮浓度变化范围为下降60%到升高50%,作物整体氮浓度平均下降14%,根系氮浓度平均下降9%。关于高CO2浓度降低作物氮浓度机制的研究,****认为,高CO2浓度降低作物氮浓度是由于“生长稀释效应”。“生长稀释效应”是指在高CO2浓度下,作物生长速度加快,作物将无机物转化为有机物的能力提高,光合作用产生的淀粉、糖和非结构性碳水化合物等在作物体内的积累量增加,因此导致作物氮浓度下降[43];但FENG等[44]研究表明,高CO2浓度下作物氮浓度的降低幅度与生物量的增加幅度并没有相关性,所以他认为“生长稀释效应”假说并不成立;另一种解释是,作物在高 CO2浓度下,其叶片的气孔导度和蒸腾作用下降,从而抑制了土壤溶液中的氮向根系的质流过程,这可能会导致根际土壤有效氮含量以及作物根系氮浓度的降低。研究发现,在CO2升高条件下,小麦的叶片氮浓度与其蒸腾速率均下降,且二者呈正相关关系[45]。此外,氮浓度降低也可能与作物对氮素利用效率的提高有关,有研究发现,CO2浓度升高导致C3作物对碳固定效率提高,导致其利用少量的1,5-二磷酸核酮糖羧化酶和叶片蛋白质氮素就能够产生更多的干物质,因此高CO2浓度提高C3作物的氮素利用效率,从而导致作物的氮浓度下降[46]。
2.2 温度升高与作物氮浓度
升温一方面通过直接改变作物的氮吸收对作物氮浓度产生影响,另一方面通过间接改变土壤-作物氮素循环影响氮浓度[47]。通常情况下,升温有利于土壤有机氮的矿化,提高了土壤氮素有效性,从而促进了作物对氮素的吸收并增加了作物氮浓度。例如,夜间温度由22℃上升到32℃时,加速了土壤有机氮的矿化,水稻叶片中的氮浓度显著升高7.8%[13];通过位于江苏常熟的FACE平台研究也发现,温度升高2℃,水稻叶片中氮浓度在拔节、开花和成熟期分别比常温下增加30.3%、21.8%、20.7%,水稻穗中氮浓度增加5.8%[12]。LI等[11]研究发现升温能够促进作物氮浓度增加,在平均增温0.8℃条件下,水稻茎叶的平均氮浓度在灌浆前期增加了24%,在灌浆后期增加了64%,并分析氮浓度的升高可能与升温条件下水稻生物量降低有关。然而,TIAN等[48]通过为期3年的FACE试验对小麦研究发现,平均升高1.5℃条件下,虽然小麦总吸氮量以及茎叶中的氮浓度增加,但是降低了小麦籽粒的氮浓度。这可能是由于高温抑制了小麦的灌浆,导致籽粒氮积累和氮浓度下降,甚至造成了小麦面粉品质的下降[49]。此外,在年平均温度较高的地区,升温对氮素矿化的促进作用很快就会消失,并且随着时间的推移,升温导致作物叶片氮浓度显著下降,产生这种差异可能与作物所处温度带以及增温幅度的不同有关[50]。2.3 作物氮浓度对大气CO2浓度和温度同时升高的响应
大气CO2浓度和温度同时升高对作物氮浓度产生影响。一方面,有****认为二者没有互作效应,例如ROY等[51]利用位于印度的OTC对水稻研究发现,在高CO2浓度(550 μmol·mol-1)并同时升温2℃下,虽然水稻的茎和根中的氮浓度均显著降低,但是二者对水稻氮浓度的影响不产生交互作用;而且,作物籽粒的蛋白质浓度与氮浓度呈显著正相关关系。研究发现,单独升高CO2浓度至500 μmol·mol-1,水稻和小麦籽粒的蛋白质浓度分别下降了7%和15%,单独升温2℃条件下,小麦籽粒的蛋白质浓度无显著变化而水稻增加了9%,而在同时升高CO2浓度至500 μmol·mol-1并升温2℃条件下,小麦和水稻的籽粒蛋白质浓度无显著变化,并统计分析升温和升高CO2浓度没有交互作用[52]。另一些研究发现CO2和温度同时升高对作物氮浓度具有互作效应,例如,通过位于澳大利亚的作物FACE平台以及江苏扬州的FACE平台研究发现,当大气CO2浓度从390 μmol·mol-1升高到550 μmol·mol-1或从360 μmol·mol-1上升到560 μmol·mol-1,水稻籽粒中的氮浓度下降,在此基础上同时升温10℃左右或者升温0.7—0.9℃后,水稻籽粒氮浓度降低幅度减少,说明高温减轻了高CO2对氮浓度的抑制作用[11,53]。WANG等[54]研究也发现,在水稻抽穗期,单独升高200 μmol·mol-1CO2浓度使叶片氮浓度下降了6%,单独升温1.4℃使水稻叶片的氮浓度增加了11%,然而,同时升高200 μmol·mol-1 CO2和升温1.4℃提高了水稻叶片的氮浓度,也同样表明温度升高可以减轻高CO2对水稻叶片氮浓度的抑制作用。此外,LI等[11]研究发现,单独升温0.8℃条件下,水稻茎叶的氮浓度在灌浆期前后显著升高24%—64%,而同时升高200 μmol·mol-1 CO2浓度和升温0.8℃条件下,水稻茎叶氮浓度与正常环境条件下没有显著差异,表明了高CO2浓度削弱了高温对作物氮浓度的促进作用,二者产生了相互抵消的互作效应。值得注意的是,以上研究结果的差异可能与CO2浓度及温度升高的幅度、试验方法、甚至是土壤类型和气候条件的差异有关。3 作物氮分配
在作物生长过程中,氮素的分配对籽粒产量的形成具有重要的意义。作物生长前期的氮素大多分配给茎叶等营养器官以供其充分地进行光合作用,促进干物质生产,生长后期将一部分氮素再分配到生殖器官。例如小麦籽粒中有70%—90%的氮素来源于其茎叶氮素的再分配[55]。作物对氮素的分配机制为作物高产奠定了基础。3.1 大气CO2浓度升高与作物氮分配
CO2是光合作用的原料,环境中CO2浓度的变化会对光合作用以及光合氮利用效率产生影响,进而对作物氮分配产生影响[56]。研究发现,高CO2浓度有利于增加氮素向籽粒进行分配。例如对水稻研究发现,当CO2浓度升高200 μmol·mol-1时,氮素向水稻的茎和叶分配显著降低,但是向穗的分配增加了35.4%[57]。HAN等[58]通过位于北京的FACE平台对小麦研究发现,高CO2浓度(550 μmol·mol-1)条件下,小麦叶片中的氮分配降低了6.5%,而小麦穗中的氮分配增加了1.5%。这可能是由于在大气CO2浓度升高条件下,作物生殖生长期的籽粒生物量增加而导致其氮素库容量显著增大,对氮素的需求量也随之增加,因此在根系吸氮量有限的条件下,通过提高茎秆和叶片中氮素向籽粒的再分配来补充作物籽粒中的氮素。在作物的不同生育时期,CO2升高对作物氮素的分配的影响也不同,这与作物在不同生育时期对氮素需求及生理特性动态的差异有关。例如,马红亮等[59]研究发现,CO2浓度升高200 μmol·mol-1导致水稻叶片氮素含量在分蘖期与拔节期分别比正常CO2浓度下升高4.1%和5.7%,但在抽穗期与成熟期分别降低0.3%和7.4%;穗中氮素含量在抽穗期增加61.3%,成熟期增加6.4%;通过位于江苏无锡的FACE平台研究发现,CO2浓度升高200 μmol·mol-1时,水稻在孕穗前的茎和叶片中氮素约占地上部总含氮量的60%—66%,但在孕穗期之后所占比例明显下降且显著低于正常CO2条件,在籽粒成熟期达到最低,水稻穗中分配的氮素随着水稻的生长发育而逐渐增加,抽穗期穗中氮素占地上部总氮的10.1%,到籽粒成熟期时增加至60.7%,且显著高于正常CO2浓度下穗中氮分配比例[39]。以上均说明CO2升高有利于氮素向籽粒的分配,并且对比不同时期分配情况的变化可以发现,随着作物的生长发育,高CO2浓度下氮素向籽粒的分配的趋势更加明显。
3.2 温度升高与作物氮分配
温度影响作物氮分配与高温下作物生长过程中的生理变化有关[47]。研究发现,如果水稻在开花期经历高温,则会导致花药开裂困难,花粉量减少,且抑制其花粉管伸长,影响水稻授粉受精过程以致结实率降低;灌浆期的持续高温会导致水稻早衰、有效灌浆期缩短,使得同化产物积累量下降[60],因此,此时期高温最终导致了水稻茎和叶中的氮素向籽粒的转运降低。例如,通过FACE试验对水稻研究发现,相比环境温度增温2℃导致水稻茎中氮分配比例增加52.5%,叶片中氮分配比例增加27.2%,但是穗中氮分配比例降低了17.8%[12]。MACABUHAY等[53]指出,当环境温度超过小麦适宜生长的温度阈值上限(35℃)时,小麦的CO2同化率降低,光合化产物减少,干物质和氮素的积累也随之减少,而且,高温胁迫会在小麦开花期导致花朵败育从而减少了籽粒数量;在作物灌浆期,高温胁迫也会降低小麦向籽粒中氮素养分的供应,也随之降低了籽粒重量。因此,高温也会导致氮素向小麦籽粒中的分配。此外,有研究发现,作物在高温下通过叶绿素的降解来减少接收太阳辐射的光量子数,以防止体内温度过高或产生多余的自由基对植物造成伤害[56],而叶绿体的降解导致作物叶片氮素分配比例降低。3.3 作物氮分配对大气CO2浓度和温度同时升高的响应
有关CO2和温度同时升高对作物氮素的分配是否具有交互作用的研究较少。KIM等[61]通过韩国的TGC(Temperature Gradient Chamber,温度梯度室)研究发现,温度从 25.2℃升高到27.3℃时,水稻茎中籽粒氮分配比例降低28.8%;CO2浓度从380 μmol·mol-1升高到622 μmol·mol-1时,水稻籽粒氮分配比例增加35.7%;CO2浓度和温度同时升高时,籽粒氮分配比例降低19.6%。此外,在不同条件下,水稻向茎和根的氮分配比例均与籽粒分配比例呈负相关关系。分析原因主要是增温诱导了水稻开花不育而抑制了氮素向籽粒分配,但这种抑制作用可以被大气CO2浓度升高所缓解。然而,张立极等通过位于江苏常熟的FACE平台研究发现,单独升高CO2浓度至500 μmol·mol-1或者单独升温2℃时,水稻穗中氮分配比例分别降低12.4%和17.8%,而当CO2浓度和温度同时升高时,水稻穗中氮分配比例降低9.1%,表明升高CO2浓度和温度对氮素的分配具有反向的交互作用[12]。造成这种差异的原因可能与CO2的升高幅度以及土壤类型不同有关,因此,在不同土壤类型上,不同作物的氮分配对CO2浓度和温度升高的响应规律及生理机制值得进一步研究。目前,CO2浓度和温度升高影响氮转运和分配机制的研究开展得也不多,在二者同时升高的条件下,作物的1,2-硝酸盐转运蛋白受到抑制,所以可能会减缓硝酸盐在作物中的运输[62],从而抑制了氮素在作物中的转运和分配,但是其如何影响氮素向不同器官中转运和分配的过程尚不清楚。
4 土壤氮转化
土壤微生物作为土壤有机质中最活跃且最不稳定的部分,也是土壤氮素循环和能量流动的重要推动力,影响土壤氮素的转化和氮有效性。土壤氮库中的氮素主要以有机氮的形式存在,有机氮占土壤全氮的95%以上,但作物主要吸收土壤中可溶性无机态氮。土壤铵态氮(NH4+-N)和硝态氮(NO3--N)是作物生长发育过程中的主要氮源。土壤NH4+-N可以被作物直接吸收并同化,而作物在吸收NO3--N后,需要在硝酸还原酶的作用下先将NO3--N还原为NH4+-N,然后NH4+-N在多种酶的作用下进一步合成氨基酸才能供作物利用。因此,土壤氮库中的有机氮只有先通过微生物的矿化作用才能成为可被作物吸收的无机态氮,有机氮的矿化速率决定了土壤有效氮含量。除土壤有机氮的矿化作用以外,硝化过程与反硝化过程也对土壤氮的有效性产生深刻影响。由于土壤中CO2浓度大约是大气中的10—50倍,因此大气中CO2浓度升高对土壤微生物几乎不产生直接影响,而是通过影响作物根系分泌物和脱落物对土壤微生物产生间接影响,然后再进一步影响土壤氮转化[63]。当大气CO2浓度升高后,作物同化碳增加,20%—50%的光合产物运送到地下,增加了作物根系生物量以及根系分泌物,同时也增加了作物残体的生物量[64],改变了根际土壤的化学及生物特性,进而影响到根际微生物的群落组成、结构、活性以及功能,这其中包括参与土壤氮转化的微生物,而且土壤中氮矿化、硝化和反硝化等过程均受到影响[65,66]。温度是控制土壤氮转化的重要因子之一,温度升高通过影响参与土壤有机氮矿化、氨化、硝化、反硝化等过程中的微生物群落的组成结构、微生物多样性、酶的活性等来影响土壤氮转化[67]。
4.1 土壤酶
土壤氮素的转化及矿质化是在土壤酶参与的酶促反应下进行的。土壤酶活性能够反映出土壤中生化过程的方向和强度,土壤中有效氮的含量与土壤酶的活性有着密切关系。大气CO2浓度升高加快作物根系的生长代谢,增加光合碳向根系的运输量,并加速根系的凋落和分解,土壤表层有机质含量升高,促进微生物的生长。土壤微生物和作物根系作为分泌土壤酶的主要来源,二者在大气CO2浓度升高条件下的积极反应增加了土壤酶含量,并提高了土壤酶活性。例如,有研究表明,大气CO2浓度升高显著提高了土壤水解酶、脲酶、蛋白酶和多酚氧化酶等的酶活性,但也会降低反硝化酶的活性[68]。
温度升高可通过多方面因素对土壤酶产生直接或间接影响,如影响酶动力学、土壤养分矿化速率、土壤微生物等。升高温度可以提高酶动力学常数,而且有利于增加酶促反应的底物,从而显著提高土壤酶的活性。有研究表明,增温5℃条件下,春小麦根际土壤的脲酶活性在拔节期、分蘖期、抽穗期分别提高了77%、33%、20%,土壤转化酶活性分别提高了56%、39%、7%[69]。然而,吴金水研究团队等通过在不同温度下(5、15、25、35℃)厌氧培养水稻土,发现参与氮循环关键酶之一的几丁质酶,只有在添加外源不稳定碳之后才表现出对温度敏感性,在低温条件下,由于底物可利用性满足土壤微生物的需求,因此,此时温度是限制土壤酶活性的主要因子;而随着温度的升高,快速消耗的底物含量可能成为酶活性的主要因素[70]。此外,升温可能导致土壤含水量降低,进而也会对土壤酶活性产生影响。
大气CO2浓度和温度互作可能通过改变土壤理化性质、微生物区系及活性、微生物呼吸作用等影响土壤酶活性。刘远等[71]研究表明,麦田土壤酶活性对温度响应比CO2更为敏感,这是由于温度直接影响土壤酶活性,而大气CO2浓度升高对土壤酶的影响主要通过作物的间接作用引起的。此外,应该注意到,CO2浓度与温度升高对土壤酶活性的影响与二者升高的幅度有关,研究发现,土壤酶活性对小幅度增加温度的响应明显小于季节性温度变化引起的响应[72]。
4.2 土壤有机氮矿化作用
土壤有机氮的矿化作用是指有机氮在微生物分解作用下的氨化过程,即有机氮分解后形成NH4+-N的过程。在一定条件下,土壤有机氮的矿化程度决定了土壤中可被作物吸收利用的有效氮的水平,而大气 CO2浓度升高对土壤有机氮的矿化产生影响。研究发现,CO2浓度升高后,作物根系分泌物显著增加[73,74],土壤的微生物量和活性增高[75],加速了土壤有机氮的矿化。此外,大气CO2浓度升高降低了作物的气孔导度,所以有利于保持土壤含水量,促进土壤中的微生物分泌的木质素酶、蛋白酶和脲酶等酶的活性[76],进而促进了土壤中有机氮的矿化,为作物提供可利用的无机氮[77]。高大气CO2浓度下的作物残体对土壤有机氮的矿化过程产生影响[66]。作物残体进入土壤后,土壤中有效氮的含量受到微生物的固持作用和有机氮矿化作用二者动态变化的影响。一方面,高大气CO2浓度下作物残体C/N含量较高,土壤微生物在获得丰富碳源的同时,增加了对土壤中无机氮的固持,虽然微生物可能促进土壤有机氮的矿化以利用更多的无机氮,但是总体上表现为矿质氮的净生物固持,土壤中有效氮含量降低,这可能是限制作物氮浓度的原因之一。另一方面,土壤中C/N随着有机体分解和CO2释放而降低,微生物对氮的固持速率逐渐小于有机氮的矿化速率,此时表现为有机氮的净矿化[78]。因此,在CO2升高条件下,作物残体虽然短期内加强了微生物的氮固持,但最终可能会促进土壤有机氮的矿化,增加了土壤中有效氮含量[79,80],有利于作物吸收利用。TORBERT等[81]总结了采用FACE和OTC方法模拟CO2浓度升高对农田生态系统中土壤有机氮分解影响的研究,发现CO2浓度升高条件下有机氮矿化速率减少,认为作物残体分解速率受到限制的原因是土壤微生物对氮素的固持。因此,高CO2条件下氮矿化与固化是多因素耦合过程,应从土壤碳氮平衡角度综合系统分析。
升温通过直接和间接两种方式影响土壤有机氮矿化,一方面,温度升高会增加微生物新陈代谢和酶活性[82],从而加速土壤有机氮的矿化过程,导致土壤中可供微生物及作物吸收和利用的有效态氮素增加[83]。另一方面,高温影响微生物的耗氧量和土壤的通气环境,因此改变了参与土壤有机质矿化的微生物的群落组成。一般认为,在5—35℃温度范围内,随着温度的升高,微生物活动明显增强,在此温度范围内,氮的矿化速率与温度呈正相关。RUSTAD等[67]利用meta分析方法研究了全球32个生态系统变暖试验中土壤氮素矿化规律,结果发现,其中在0.3—6℃增温范围内进行2年以上的增温实验,均显著提高了土壤表层净氮矿化速率,使土壤净氮矿化速率平均提高了46%,并且发现升温幅度与净氮矿化量之间存在显著的正相关关系。BAI等[84]也整合了51项研究中的528个有机氮矿化数据,发现温度升高导致土壤有机氮矿化速率提高了约52.2%。与前者相比,提高有机氮矿化速率幅度产生差异的原因可能是试验环境,增温方法以及增温幅度的不同,但都揭示了升温有利于有机氮矿化的规律。在大气CO2浓度和温度同时升高对土壤有机氮矿化影响的研究方面,HAGHNIA等[85]研究发现,单独升温10℃会加速有机氮矿化,而同时将CO2浓度从350 μmol·mol-1升高至700 μmol·mol-1时,土壤矿化速率增加幅度更大,说明CO2和温度对土壤有机氮矿化具有正向的互作效应。DAI等通过整合135项研究中的1 270份氮转化数据发现,土壤氮矿化速率与土壤CO2排放速率呈显著正相关,并表明微生物碳代谢增强能够促进土壤氮循环过程,全球气候变化下的微生物驱动的碳和氮转化过程存在耦合机制[86]。由于CO2浓度升高导致作物向土壤中输入的光合碳增加,而升温又加速了土壤碳的转化,因此,土壤碳转化的变化可能是CO2和温度同时升高对土壤氮矿化等过程产生互作效应的原因之一。其次,CO2和温度同时升高条件下,作物光合碳输入的增加也同样会引起土壤的激发效应,从而促进土壤有机氮的矿化过程,也为作物提供了更多的有效氮,促进了作物氮吸收。
4.3 土壤硝化作用
土壤硝化作用是在有氧状态下,硝化微生物将铵盐氧化成硝酸盐的过程,该过程分为氨氧化和亚硝酸氧化两个阶段,其中,氨氧化过程是硝化过程的限速步骤。土壤硝化过程主要由化能自养氨氧化细菌(AOB)和古菌(AOA)和亚硝酸盐氧化细菌(NOB)进行[87]。如前文所述,在大气CO2浓度升高条件下,作物根系分泌物和凋落物的增加促进了土壤有机氮的矿化,因此,矿化产物NH4+的增多促进了土壤硝化速率的提高。然而,有研究发现,由于大气CO2浓度升高降低了作物的气孔导度和蒸腾作用,增加了土壤含水量,因此降低了土壤孔隙中的O2含量;并且,由于CO2浓度升高增加了根系沉积物导致了土壤异养微生物活性增加,与硝化微生物对O2产生竞争关系,从而也可能会抑制土壤硝化作用[68]。虽然有****发现升高大气CO2浓度降低了AOB的丰度等发面的研究[88],然而只是从相关基因的丰度方面难以下结论,有关土壤硝化过程或硝化微生物基因表达对高CO2浓度的响应还有待进一步研究。
升温影响土壤硝化作用。BRADY等研究发现,土壤硝化作用的最适温度范围一般为25—35℃,高于50℃时,土壤硝化作用基本停止[89]。张树兰等[90]研究也发现,耕作土壤的硝化速率随温度升高而加快,在30℃条件下反应速率最快,而在40℃时土壤硝化速率非常微弱。分析原因是,升高温度后,导致土壤含水量降低,改善土壤通气性,土壤空气中的氧气含量增多有利于土壤硝化过程的进行。然而在另一方面,土壤溶液中O2的溶解度会随着温度的升高而降低,而且升温可能会增加异养微生物对O2的需求,因此土壤中的O2含量是两个过程动态平衡的结果,不能一概而论。此外,需要注意的是,不同温度带土壤中的硝化菌对土壤温度的阈值存在一定的差异性。例如研究发现, 美国北部的土壤硝化作用的最适温度为20—25℃, 而南部的土壤则为35℃[91]。
有关温度和CO2浓度同时升高对土壤硝化作用的影响研究中发现,小麦开花期和成熟期的硝化潜力显著降低,而单独升高CO2浓度对土壤硝化潜力无影响。但是,当同时升高CO2浓度和温度时,土壤硝化潜力呈现下降趋势[68]。需要说明的是,土壤硝化潜力是在实验室条件下测定的,不能代表田间原位土壤的硝化过程,而且田间条件下,土壤温度的升高一般滞后,因此,其研究结果需进一步的田间试验验证。LIU等[92]对田间原位研究发现,CO2浓度升高至500 μmol·mol-1并同时升温2℃条件下,显著改变了水稻根际土壤氨氧化细菌的群落结构,并且升温抑制了高CO2浓度对硝化作用的促进作用。利用位于江苏常熟的FACE平台研究发现,单独升高CO2浓度至500 μmol·mol-1能够显著提高小麦成熟期土壤硝化速率,其原因是在小麦成熟期,土壤氨氧化古菌和氨氧化细菌数量在CO2浓度升高处理中显著提高。但是在高CO2浓度条件同时增温2℃时,小麦成熟期的土壤硝化速率没有显著变化[71],同样说明了高温抑制了CO2浓度升高对土壤硝化速率的促进作用,二者之间存在反向的交互作用。然而,NGUYEN等[93]对种植棉花土壤研究发现,同时升高CO2浓度(+132 μmol·mol-1)和温度(+1.1℃)显著促进了土壤硝化速率。目前对土壤根际硝化过程在CO2浓度和温度升高条件下的变化机制仍未明确,为更真实、更准确地预测未来大气CO2升高及全球变暖对土壤硝化过程的影响, 还有待于继续研究探索。
4.4 土壤反硝化作用
土壤反硝化作用是指在厌氧条件下,由反硝化微生物将硝酸盐还原成NO、N2O和N2的过程,反硝化作用造成了土壤无机氮的损失。由于反硝化细菌将土壤中的硝酸盐还原为氮气,降低了土壤中可供作物吸收利用的氮库,所以探究高CO2浓度下反硝化作用特征具有重要意义。目前有关CO2浓度升高对土壤反硝化过程的影响的结论不一致。INESON等[94]通过瑞士的FACE平台研究发现,高CO2浓度(600 μmol·mol-1)促进了土壤反硝化微生物的活性,使得土壤N2O排放量增加27%。分析原因是由于,如前文所述,CO2升高使得更多的碳(包括根系沉积物和分泌物等)进入到土壤中,从而为反硝化微生物提供了充足的碳源和能量[71],并且,碳源投入的增多加剧了土壤中氧气的消耗,例如作物根系以及土壤微生物的呼吸作用增强,形成的厌氧环境有利于反硝化过程的进行。然而,CO2升高条件下,作物根系对NO3-的吸收量增加,导致了反硝化微生物可利用的底物减少,因此根系与微生物对氮素的利用产生竞争作用,这甚至也可能会导致根系氮素的亏缺。BARNARD等[65]通过meta分析总结了CO2升高对土壤反硝化过程以及N2O排放的影响,结果发现,高CO2浓度显著降低了反硝化酶活性,平均降幅为20%,并分析在CO2浓度升高条件下,NO3--N等电子受体的减少可能导致了对反硝化酶的抑制作用。此外,CHENG等[95]研究表明,在10年的田间试验中,CO2浓度升高200 μmol·mol-1对硝化作用无显著影响。除了试验材料和田间气候的不同外,产生这种差异的原因可能与土壤类型和CO2浓度的升高幅度有关。
温度是影响土壤反硝化作用的一个重要因素,反硝化作用的适宜温度范围为5—75℃,温度过高或过低都会抑制反硝化作用。在适宜温度范围内,随着温度的升高,反硝化作用速率也加强。研究发现在大于15℃时,反硝化速率随着温度升高而升高,并在60—67℃时达到最大值[96]。N2O作为反硝化过程的重要产物之一,LI等[97]整合46项全球气候变化研究中的N2O排放数据,进行meta分析发现,全球变暖导致陆地生态系统N2O平均排放量显著增加了33%。有关升温促进土壤反硝化过程的机制主要体现以下几个方面:首先,升温加速了土壤矿化过程,促进土壤中无机态氮含量增加,从而使得反硝化过程的电子受体增多[84];其次,如前文所述,升温促进了作物根系的生长并加速了细根的周转,根系分泌物和沉积物的增加为反硝化微生物提供了更多的碳源和能源;最后,SMITH等[98]研究发现,升温由于加速了作物根系呼吸作用,从而消耗了更多的氧气,为反硝化过程提供了适宜的厌氧环境。反硝化过程是农田生态系统中氮气损失的主要途径之一,反硝化作用的增强降低了土壤中有效氮含量,不利于作物的吸收利用。
CO2浓度和温度升高对于土壤的反硝化过程产生交互影响,RAKSHIT等[99]对麦田生态系统研究发现,单独升高CO2浓度至650 μmol·mol-1时,土壤硝酸还原酶活性下降14%—20%,反硝化细菌的数量在小麦开花期减少了14%,在成熟期减少了8%,但在此基础上同时升高3℃时,小麦开花期的反硝化细菌数量急剧下降,而在成熟期保持不变,表明温度和CO2的升高对土壤反硝化作用具有一定的交互作用。土壤反硝化作用对CO2浓度和温度升高的响应受不同土壤环境及作物类型的影响。
5 研究展望
人类活动的扰动已加剧了全球气候变化,这不仅影响了地上作物的生长和产量,还深刻影响着由微生物介导的氮素生物地球化学循环过程(图1)。全球范围内持续的升温加速了农田土壤有机氮的矿化,越来越高的大气CO2浓度增加了农田作物对土壤氮素的吸收量,最终可能会导致土壤氮素输出量增多。农田生态系统长期的大量土壤氮素输出可能会导致土壤质量下降,并且影响农田生态系统的可持续利用。全球气候变化形势日趋严重,因此,在CO2浓度和温度不断升高的背景下,农田生态系统中土壤氮素平衡能否维持,不断增加的氮素输出是否造成了农田土壤氮素的亏缺,是否需要通过提高施肥量等方式增加氮素输入以满足作物生长发育的需要,这些问题亟需研究与解决。图1
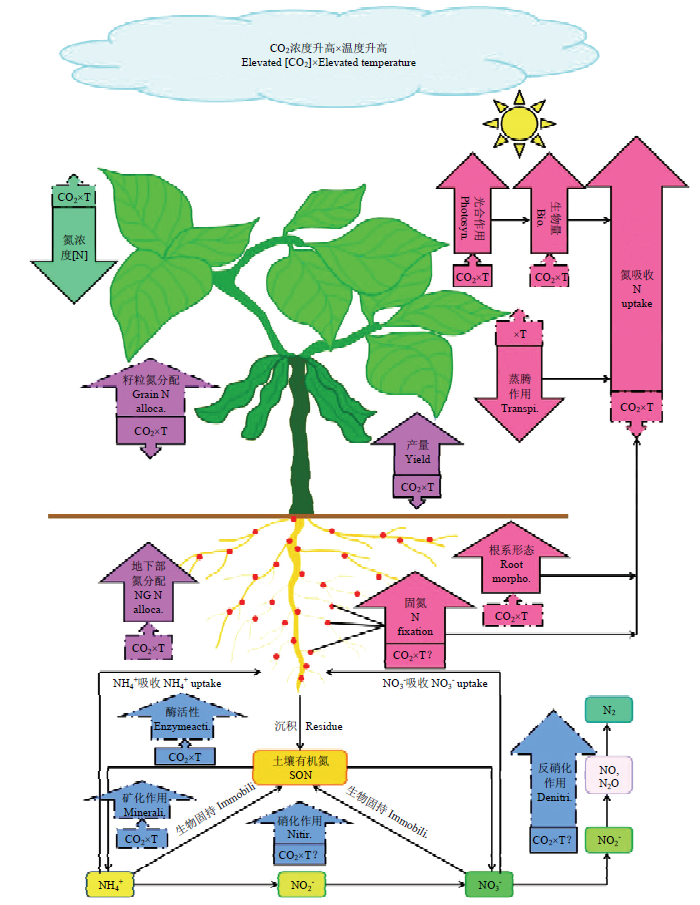
图1大气CO2浓度升高与温度升高对作物-土壤氮循环的交互影响
图中实线箭头表示现有结果较为一致;虚线箭头表示现有研究结果不一致;CO2×T表示CO2浓度和温度互作,问号表示互作影响未知
Arrows with solid and dot line indicate consistent and inconsistent results from published data; CO2×T indicates the interaction between CO2 and temperature, question mark means the interaction is unknown






T: Temperature; Photosyn: Photosynthesis; Bio.: Biomass; Transpi.: Transpiration; [N]: N concentration; Grain N alloca.: Grain N allocation; NG N alloca.: Underground N allocation; Root morpho.: Root morphology; Enzyme acti.: Enzyme activity; Immobili.: Immobilization; Minerali.: Mineralization; Nitri.: Nitrification; Denitri.: Denitrification
Fig. 1Interaction between atmospheric CO2 concentration and temperature on crop-soil continuum nitrogen cycle
随着人们对全球气候变化的关注,地上部分即大气-作物相关的研究已取得了长足进展,但地下部分土壤微生物及其与地上部分之间的耦合关系仍比较薄弱,特别是微生物主导的氮循环过程及其对全球气候变化的反馈机制仍是研究的关键。CO2浓度和温度升高对土壤氮转化有着深刻影响,土壤氮转化必须在微生物的参与和驱动下完成,但是由于植物-土壤-微生物系统的复杂性, 以及土壤微生物与土壤生态系统功能和过程的复杂关联。氮转化有关微生物是如何通过调节自身机制去响应、适应和反馈大气CO2浓度和温度升高仍是一个亟待解决的问题。由于CO2浓度和温度升高条件下,土壤-作物氮代谢耦合过程涉及土壤-微生物、土壤-根系、根系-微生物等界面,因此需要针对我国不同气候和土壤类型的农田生态系统中,研究不同界面上调控氮素转化的各因素之间的协同关系,并分析土壤、微生物和作物响应CO2浓度和温度升高的变化如何影响氮代谢和循环过程,最终为应对全球气候变化下农田生态系统的氮素循环变化提出作物-土壤-微生物系统的协同调控原理和措施。然而,现阶段有关升高CO2浓度和温度互作对参与土壤氮转化的微生物影响的研究比较少,气候变化下土壤微生物对氮转化的调控机理尚不清楚。原因是据估算,每克土壤最多含有100亿微生物个体,100万种不同微生物[100],因此,从这些难以计数的土壤微生物中,原位鉴别参与土壤氮素循环的微生物驱动者难度较大,并且之前的研究方法很难将特定微生物结构与其功能相互联系,无法获得微生物网络间的相互作用及其代谢的直接信息。采用15N同位素标记的方法已经被国内外****广泛应用在氮循环研究。而且随着分子生物学的飞速发展,16S rRNA方法结合DGGE、T-RFLP、qPCR以及RT-PCR技术是目前用来反映氮循环微生物丰度、多样性及功能的主要手段,近年来,如稳定性同位素探针(Stable Isotope Probing,SIP)等技术不断发展和成熟,能够将复杂环境中微生物物种组成及其生理功能进行耦合分析,该技术采用“稳定同位素”原位标记环境样品,如土壤中的“特定微生物核酸DNA/RNA”,采用分子生物学方法分析稳定同位素标记的核酸DNA/RNA,从而能够揭示地上-地下氮循环过程的微生物驱动机理。因此,新技术在根际微生态学领域的应用能够为我们提供许多关于微生物遗传多样性的信息[101]。然而,要理解土壤氮转化微生物群落结构的行为和生理特性仅靠基因丰度的定量以及转录组学是不够的,还需要在蛋白质水平上土壤样品进行检测,即应当重视有关“基因表达”的相关研究技术,这是因为蛋白质能直接反映生物功能和活性[102],因此对微生物群落的蛋白质结构进行分析的蛋白组学在研究土壤微生物群落功能性方面更加直接,能够有效地帮助人们建立微生物基因与功能之间的关系[103]。所以,通过同位素标记技术和氮循环微生物的原位技术(如SIP技术),结合蛋白质组学技术等新兴技术的研究方法,不仅可以明确根际土壤中参与土壤氮循环过程的关键微生物功能种群,而且能够更加准确地揭示大气CO2和温度升高下与土壤有机氮矿化,硝化与反硝化等有关氮循环微生物的调控过程,对深入了解气候变化影响农田生态系统中作物-土壤氮素循环的过程具有重要意义。因此,未来应该重视大气CO2浓度和温度升高互作背景下,作物-土壤-微生物之间的耦合作用和分子机理研究。
参考文献 原文顺序
文献年度倒序
文中引用次数倒序
被引期刊影响因子
DOI:10.1126/science.aaa4984URLPMID:25931559 [本文引用: 1]

Current predictions of extinction risks from climate change vary widely depending on the specific assumptions and geographic and taxonomic focus of each study. I synthesized published studies in order to estimate a global mean extinction rate and determine which factors contribute the greatest uncertainty to climate change-induced extinction risks. Results suggest that extinction risks will accelerate with future global temperatures, threatening up to one in six species under current policies. Extinction risks were highest in South America, Australia, and New Zealand, and risks did not vary by taxonomic group. Realistic assumptions about extinction debt and dispersal capacity substantially increased extinction risks. We urgently need to adopt strategies that limit further climate change if we are to avoid an acceleration of global extinctions.
[本文引用: 1]
DOI:10.1093/aob/mcq085URLPMID:20430785 [本文引用: 1]

BACKGROUND: Plants require at least 14 mineral elements for their nutrition. These include the macronutrients nitrogen (N), phosphorus (P), potassium (K), calcium (Ca), magnesium (Mg) and sulphur (S) and the micronutrients chlorine (Cl), boron (B), iron (Fe), manganese (Mn), copper (Cu), zinc (Zn), nickel (Ni) and molybdenum (Mo). These are generally obtained from the soil. Crop production is often limited by low phytoavailability of essential mineral elements and/or the presence of excessive concentrations of potentially toxic mineral elements, such as sodium (Na), Cl, B, Fe, Mn and aluminium (Al), in the soil solution. SCOPE: This article provides the context for a Special Issue of the Annals of Botany on 'Plant Nutrition for Sustainable Development and Global Health'. It provides an introduction to plant mineral nutrition and explains how mineral elements are taken up by roots and distributed within plants. It introduces the concept of the ionome (the elemental composition of a subcellular structure, cell, tissue or organism), and observes that the activities of key transport proteins determine species-specific, tissue and cellular ionomes. It then describes how current research is addressing the problems of mineral toxicities in agricultural soils to provide food security and the optimization of fertilizer applications for economic and environmental sustainability. It concludes with a perspective on how agriculture can produce edible crops that contribute sufficient mineral elements for adequate animal and human nutrition.
[本文引用: 1]
[本文引用: 1]
[本文引用: 1]
DOI:10.1111/pce.2015.38.issue-9URL [本文引用: 1]
URLPMID:12561186 [本文引用: 1]

The effect of atmospheric CO2 and temperature increment on crop growth, photosynthesis, yield, and water and nutrient use efficiency was summarized and discussed in this paper. Under high CO2 concentration in the future, the photosynthetic rate of crops may be enhanced to some extent, and their biomass and yield may also be increased. Their stomatal conductance may be reduced, while water use efficiency (WUE) may be increased under elevated CO2 concentration. The aboveground biomass and root production, especially fine root production, will be greatly enhanced, and consequently, litter production increased, C/N ratios increased, plant litter decomposition rates declined under CO2 enrichment. Plants grown under elevated CO2 concentration may release more substrate into rhizosphere, which may lead to an increased activity of soil microorganisms, acclerated mineralization of nutrients, and improved nutritional status of the plant.
URLPMID:12561186 [本文引用: 1]

The effect of atmospheric CO2 and temperature increment on crop growth, photosynthesis, yield, and water and nutrient use efficiency was summarized and discussed in this paper. Under high CO2 concentration in the future, the photosynthetic rate of crops may be enhanced to some extent, and their biomass and yield may also be increased. Their stomatal conductance may be reduced, while water use efficiency (WUE) may be increased under elevated CO2 concentration. The aboveground biomass and root production, especially fine root production, will be greatly enhanced, and consequently, litter production increased, C/N ratios increased, plant litter decomposition rates declined under CO2 enrichment. Plants grown under elevated CO2 concentration may release more substrate into rhizosphere, which may lead to an increased activity of soil microorganisms, acclerated mineralization of nutrients, and improved nutritional status of the plant.
DOI:10.1016/j.agrformet.2015.12.061URL [本文引用: 2]
DOI:10.1016/j.fcr.2005.09.015URL [本文引用: 1]
DOI:10.1016/0378-4290(94)00104-KURL [本文引用: 1]
DOI:10.1007/s11284-017-1450-7URL [本文引用: 7]
[本文引用: 7]
[本文引用: 7]
DOI:10.1016/j.agrformet.2010.05.001URL [本文引用: 5]
DOI:10.1086/506154URL [本文引用: 1]
DOI:10.1146/annurev.arplant.48.1.609URL [本文引用: 1]
DOI:10.1016/j.eja.2013.10.008URL [本文引用: 1]

A field experiment was carried out to assess the impact of elevated carbon dioxide (CO2) and temperature on phosphorous (P) nutrition in relation to organic acids exudation, soil microbial biomass P (MBP) and phosphatase activities in tropical flooded rice. Rice (cv. Naveen) was grown under chambered control (CC), elevated CO2 (EC, 550 mu mol mol(-1)) and elevated CO2 + elevated temperature (ECT, 550 mu mol mol(-1) and 2 degrees C more than CC) in a tropical flooded soil under open top chambers (OTCs) along with unchambered control (UC) for three years. Root exudates were analyzed at different growth stages of rice followed by organic acids determination. Rhizospheric soil was used for analysis of soil phosphatase, MBP and available P. The total organic carbon (TOC) in root exudates was increased by 27.5% and 30.2% under EC and ECT, respectively over CC. Four different types of organic acids viz, acetic acid (AA), tartaric acid (TA), malic acid (MA) and citric acid (CA) were identified and quantified as dominant in root exudates, concentration of these was in the order of TA > MA > AA > CA. The TA, MA, M and CA content were increased by 34.4, 31.1, 38.7 and 58.3% under ECT compared to that of UC over the period of 3 years. The P uptake in shoot, root and grain under elevated CO2 increased significantly by 29, 28 and 22%, respectively than CC. Soil MBP, acid and alkaline phosphatase activity was significantly higher under elevated CO2 by 35.1%, 27 and 36%, respectively, compared to the CC. Significant positive relationship exists among the organic acid exudation, MBP, phosphatase activities and P uptake by rice. The enhanced organic acid in root exudates coupled with higher soil phosphatase activities under elevated CO2 resulted in increased rate of soil P solubilization leading to higher plant P uptake. (C) 2013 Elsevier B.V.
[本文引用: 1]
[本文引用: 1]
DOI:10.11674/zwyf.2001.0308URL [本文引用: 1]

By use of field experiments and pot culture experiments, seed yield per day, nitrogen absorption efficiency, root morphology, leaf nitrate reductase (NRA), canopy photosynthesis eco physiological characteristics etc. were studied with 7 different corn varieties The results showed that 5 varieties of DK743, DK656, YY22, ZD2 and HD1 had high nitrogen use efficiency (NUE), but another two varieties of JD2 and SY905 had low NUE NUE were correlated with root dry weight, root length, root surface area, leaf NRA, net photosynthetic rate (Pn), stoma resistance(Sr) etc Pn, Sr, leaf NRA, total dry matter, seed yield were increased by more N applying, and the range of increasing were different among 7 varieties
DOI:10.11674/zwyf.2001.0308URL [本文引用: 1]

By use of field experiments and pot culture experiments, seed yield per day, nitrogen absorption efficiency, root morphology, leaf nitrate reductase (NRA), canopy photosynthesis eco physiological characteristics etc. were studied with 7 different corn varieties The results showed that 5 varieties of DK743, DK656, YY22, ZD2 and HD1 had high nitrogen use efficiency (NUE), but another two varieties of JD2 and SY905 had low NUE NUE were correlated with root dry weight, root length, root surface area, leaf NRA, net photosynthetic rate (Pn), stoma resistance(Sr) etc Pn, Sr, leaf NRA, total dry matter, seed yield were increased by more N applying, and the range of increasing were different among 7 varieties
URLPMID:17763743 [本文引用: 1]

Soil nitrogen (N) is one of the mineral elements absorbed in large amount by plant roots, while global change could affect its availability, and furthermore, affect the carbon (C) allocation in terrestrial ecosystem. Therefore, the study of plant root N uptake and regulation becomes an important issue in predicting the structure and function of ecosystem. In the biosphere, plants are exposed to different N forms, and long-term biological evolution and environmental adaptation resulted in a significant distinction of plant root N uptake regions and metabolic processes, as well as the regulation of the N uptake. However, plant has formed different mechanisms and strategies for N uptake, because of their living in the soil with dominant sole N form for generations. In this paper, the research advances on how plant root absorbs N and which factors control the N absorption processes were reviewed, with the biological availability of different soil N forms (nitrate, ammonium and organic N), N uptake regions in root, N loading and transport in xylem, and uptake mechanisms of different N forms emphasized. The signal regulation of N uptake and the effects of environmental factors were also considered. Several issues about the present researches on plant root N uptake were discussed.
URLPMID:17763743 [本文引用: 1]

Soil nitrogen (N) is one of the mineral elements absorbed in large amount by plant roots, while global change could affect its availability, and furthermore, affect the carbon (C) allocation in terrestrial ecosystem. Therefore, the study of plant root N uptake and regulation becomes an important issue in predicting the structure and function of ecosystem. In the biosphere, plants are exposed to different N forms, and long-term biological evolution and environmental adaptation resulted in a significant distinction of plant root N uptake regions and metabolic processes, as well as the regulation of the N uptake. However, plant has formed different mechanisms and strategies for N uptake, because of their living in the soil with dominant sole N form for generations. In this paper, the research advances on how plant root absorbs N and which factors control the N absorption processes were reviewed, with the biological availability of different soil N forms (nitrate, ammonium and organic N), N uptake regions in root, N loading and transport in xylem, and uptake mechanisms of different N forms emphasized. The signal regulation of N uptake and the effects of environmental factors were also considered. Several issues about the present researches on plant root N uptake were discussed.
DOI:10.1046/j.1365-2486.2000.00359.xURL [本文引用: 1]
DOI:10.1126/science.257.5077.1672URLPMID:17841166 [本文引用: 1]

Carbon, nutrient, and water balance as well as key plant and soil processes were simultaneously monitored for humid tropical plant communities treated with CO(2)-enriched atmospheres. Despite vigorous growth, no significant differences in stand biomass (of both the understory and overstory), leaf area index, nitrogen or water consumption, or leaf stomatal behavior were detected between ambient and elevated CO(2) treatments. Major responses under elevated CO(2) included massive starch accumulation in the tops of canopies, increased fine-root production, and a doubling of CO(2) evolution from the soil. Stimulated rhizosphere activity was accompanied by increased loss of soil carbon and increased mineral nutrient leaching. This study points at the inadequacy of scaling-up from physiological baselines to ecosystems without accounting for interactions among components, and it emphasizes the urgent need for whole-system experimental approaches in global-change research.
[本文引用: 1]
[本文引用: 1]
DOI:10.1046/j.1469-8137.2000.00678.xURL [本文引用: 1]
DOI:10.2307/2390280URL [本文引用: 1]
DOI:10.1016/S1002-0160(09)60151-XURL [本文引用: 1]

Abstract
A hydroponic experiment was carried out to study the effect of elevated carbon dioxide (CO2) on root growth of tomato seedlings. Compared with the control (350 μLL−1), CO2 enrichment (800 μLL−1) significantly increased the dry matter of both shoot and root, the ratio of root to shoot, total root length, root surface area, root diameter, root volume, and root tip numbers, which are important for forming a strong root system. The elevated CO2 treatment also significantly improved root hair development and elongation, thus enhancing nutrient uptake. Increased indole acetic acid concentration in plant tissues and ethylene release in the elevated CO2 treatment might have resulted in root growth enhancement and root hair development and elongation.DOI:10.1016/j.agrformet.2020.107954URL [本文引用: 1]
DOI:10.1093/jxb/ert367URL [本文引用: 1]

Transpiration may enhance mass-flow of nutrients to roots, especially in low-nutrient soils or where the root system is not extensively developed. Previous work suggested that nitrogen (N) may regulate mass-flow of nutrients. Experiments were conducted to determine whether N regulates water fluxes, and whether this regulation has a functional role in controlling the mass-flow of nutrients to roots. Phaseolus vulgaris were grown in troughs designed to create an N availability gradient by restricting roots from intercepting a slow-release N source, which was placed at one of six distances behind a 25 m mesh from which nutrients could move by diffusion or mass-flow (termed mass-flow treatment). Control plants had the N source supplied directly to their root zone so that N was available through interception, mass-flow, and diffusion (termed interception treatment). Mass-flow plants closest to the N source exhibited 2.9-fold higher transpiration (E), 2.6-fold higher stomatal conductance (g(s)), 1.2-fold higher intercellular [CO2] (C-i), and 3.4-fold lower water use efficiency than interception plants, despite comparable values of photosynthetic rate (A). E, g(s), and C-i first increased and then decreased with increasing distance from the N source to values even lower than those of interception plants. Mass-flow plants accumulated phosphorus and potassium, and had maximum concentrations at 10mm from the N source. Overall, N availability regulated transpiration-driven mass-flow of nutrients from substrate zones that were inaccessible to roots. Thus when water is available, mass-flow may partially substitute for root density in providing access to nutrients without incurring the costs of root extension, although the efficacy of mass-flow also depends on soil nutrient retention and hydraulic properties.
DOI:10.1046/j.1365-2486.2001.00406.xURL [本文引用: 1]
DOI:10.1023/A:1014244413067URL [本文引用: 1]

Three species, wheat, maize and cotton, were grown in pots and subjected to high (85–100% field capacity,





[本文引用: 1]
[本文引用: 1]
DOI:10.1104/pp.109.144113URLPMID:19755541 [本文引用: 1]
DOI:10.1093/aob/mcv140URLPMID:26346721 [本文引用: 1]

BACKGROUND AND AIMS: Additional carbohydrate supply resulting from enhanced photosynthesis under predicted future elevated CO2 is likely to increase symbiotic nitrogen (N) fixation in legumes. This study examined the interactive effects of atmospheric CO2 and nitrate (NO3(-)) concentration on the growth, nodulation and N fixation of field pea (Pisum sativum) in a semi-arid cropping system. METHODS: Field pea was grown for 15 weeks in a Vertosol containing 5, 25, 50 or 90 mg NO3(-)-N kg(-1) under either ambient CO2 (aCO2; 390 ppm) or elevated CO2 (eCO2; 550 ppm) using free-air CO2 enrichment (SoilFACE). KEY RESULTS: Under aCO2, field pea biomass was significantly lower at 5 mg NO3(-)-N kg(-1) than at 90 mg NO3(-)-N kg(-1) soil. However, increasing the soil N level significantly reduced nodulation of lateral roots but not the primary root, and nodules were significantly smaller, with 85% less nodule mass in the 90 NO3(-)-N kg(-1) than in the 5 mg NO3(-)-N kg(-1) treatment, highlighting the inhibitory effects of NO3(-). Field pea grown under eCO2 had greater biomass (approx. 30%) than those grown under aCO2, and was not affected by N level. Overall, the inhibitory effects of NO3(-) on nodulation and nodule mass appeared to be reduced under eCO2 compared with aCO2, although the effects of CO2 on root growth were not significant. CONCLUSIONS: Elevated CO2 alleviated the inhibitory effect of soil NO3(-) on nodulation and N2 fixation and is likely to lead to greater total N content of field pea growing under future elevated CO2 environments.
DOI:10.3389/fpls.2017.01546URLPMID:28959266 [本文引用: 2]

Nitrogen deficiency limits crop performance under elevated CO2 (eCO2), depending on the ability of plant N uptake. However, the dynamics and redistribution of N2 fixation, and fertilizer and soil N use in legumes under eCO2 have been little studied. Such an investigation is essential to improve the adaptability of legumes to climate change. We took advantage of genotype-specific responses of soybean to increased CO2 to test which N-uptake phenotypes are most strongly related to enhanced yield. Eight soybean cultivars were grown in open-top chambers with either 390 ppm (aCO2) or 550 ppm CO2 (eCO2). The plants were supplied with 100 mg N kg(-1) soil as (15)N-labeled calcium nitrate, and harvested at the initial seed-filling (R5) and full-mature (R8) stages. Increased yield in response to eCO2 correlated highly (r = 0.95) with an increase in symbiotically fixed N during the R5 to R8 stage. In contrast, eCO2 only led to small increases in the uptake of fertilizer-derived and soil-derived N during R5 to R8, and these increases did not correlate with enhanced yield. Elevated CO2 also decreased the proportion of seed N redistributed from shoot to seeds, and this decrease strongly correlated with increased yield. Moreover, the total N uptake was associated with increases in fixed-N per nodule in response to eCO2, but not with changes in nodule biomass, nodule density, or root length.
DOI:10.1016/j.agee.2019.04.033URL [本文引用: 1]
[本文引用: 2]
[本文引用: 2]
DOI:10.1016/j.anifeedsci.2008.07.003URL [本文引用: 1]
DOI:10.3724/SP.J.1006.2015.01295URL [本文引用: 2]

Preventing 2°C of warming and restricting the CO2 level to 450 µL L-1 are the safety threshold for climate change based on the Copenhagen Consensus. It is an important reference for the security of rice yields to study the influence of elevated air temperature and CO2 concentration on the nitrogen use of rice. In this paper, a modified open-top chamber (OTC) device was used to simulate relative 60 µL L-1 CO2 concentration rise (based on CO2 background concentration of 390 µL L-1) and 2°C temperature increase scenario in a double rice field experiment with Liangyou 287 and Xiangfengyou 9 as the early and late rice varieties respectively. There were five treatments with three replications: 1) UC: Paddy field without OTC cover; 2) CK: Check OTC with the similar temperature and CO2 concentration to the field environment; 3) CT: OTC with 2°C temperature increase; 4) CC: OTC with 60 µL L-1 CO2 concentration elevated; 5) CTC: OTC with 2°C temperature increase and 60 µL L-1 CO2 concentration elevated. The nitrogen accumulation, translocation and utilization in different treatments were explored. The results showed significant interactions between temperature increase and CO2 concentration elevated on the yield and nitrogen use. In early rice CC and CTC achieved an increase for grain yield (19.7% and 2.0%) and nitrogen accumulation (15.7% and 5.1%) compared with CK while CT presented a decrease. In late rice warming and high CO2 concentration (CT, CC, and CTC) benefited the grain yield and nitrogen uptake, increasing 9.2%, 14.4%, 18.8% and 7.3%, 10.2%, 15% compared with CK respectively. Nitrogen translocation and contribution efficiency (from stem and leaf to grain) of CC and CTC was lower than that of CK in early rice, and higher than that of CK in late rice. Nitrogen recovery efficiency of CC and CTC reached to 45.7% and 48.5% in early and late rice respectively, achieving the highest increase of 35.3% and 33.1% compared with that of CK. CC and CTC got the highest nitrogen agronomic efficiency by 23.1 kg kg-1and 26.9 kg kg-1 in early and late rice respectively, and CC got the highest nitrogen physiological efficiency by 50.7 kg kg-1 and 56 kg kg-1in both early and late rice. There existed no significant difference between CK and UC, which suggested the impact on rice growth under OTC covering was slight. In conclusion, it tends to a negative effect with 2°C temperature increase on yield and nitrogen utilization for early rice, while a positive effect for late rice. A positive effect with 60 µL L-1 CO2 concentration elevated always exists during double rice growth. The condition of 2°C temperature increase and 60 µL L-1 CO2 concentration elevated has an antagonistic effect on early rice, while a synergistic effect on late rice.
DOI:10.3724/SP.J.1006.2015.01295URL [本文引用: 2]

Preventing 2°C of warming and restricting the CO2 level to 450 µL L-1 are the safety threshold for climate change based on the Copenhagen Consensus. It is an important reference for the security of rice yields to study the influence of elevated air temperature and CO2 concentration on the nitrogen use of rice. In this paper, a modified open-top chamber (OTC) device was used to simulate relative 60 µL L-1 CO2 concentration rise (based on CO2 background concentration of 390 µL L-1) and 2°C temperature increase scenario in a double rice field experiment with Liangyou 287 and Xiangfengyou 9 as the early and late rice varieties respectively. There were five treatments with three replications: 1) UC: Paddy field without OTC cover; 2) CK: Check OTC with the similar temperature and CO2 concentration to the field environment; 3) CT: OTC with 2°C temperature increase; 4) CC: OTC with 60 µL L-1 CO2 concentration elevated; 5) CTC: OTC with 2°C temperature increase and 60 µL L-1 CO2 concentration elevated. The nitrogen accumulation, translocation and utilization in different treatments were explored. The results showed significant interactions between temperature increase and CO2 concentration elevated on the yield and nitrogen use. In early rice CC and CTC achieved an increase for grain yield (19.7% and 2.0%) and nitrogen accumulation (15.7% and 5.1%) compared with CK while CT presented a decrease. In late rice warming and high CO2 concentration (CT, CC, and CTC) benefited the grain yield and nitrogen uptake, increasing 9.2%, 14.4%, 18.8% and 7.3%, 10.2%, 15% compared with CK respectively. Nitrogen translocation and contribution efficiency (from stem and leaf to grain) of CC and CTC was lower than that of CK in early rice, and higher than that of CK in late rice. Nitrogen recovery efficiency of CC and CTC reached to 45.7% and 48.5% in early and late rice respectively, achieving the highest increase of 35.3% and 33.1% compared with that of CK. CC and CTC got the highest nitrogen agronomic efficiency by 23.1 kg kg-1and 26.9 kg kg-1 in early and late rice respectively, and CC got the highest nitrogen physiological efficiency by 50.7 kg kg-1 and 56 kg kg-1in both early and late rice. There existed no significant difference between CK and UC, which suggested the impact on rice growth under OTC covering was slight. In conclusion, it tends to a negative effect with 2°C temperature increase on yield and nitrogen utilization for early rice, while a positive effect for late rice. A positive effect with 60 µL L-1 CO2 concentration elevated always exists during double rice growth. The condition of 2°C temperature increase and 60 µL L-1 CO2 concentration elevated has an antagonistic effect on early rice, while a synergistic effect on late rice.
DOI:10.1111/nph.2006.170.issue-2URL [本文引用: 1]
DOI:10.1016/j.fcr.2006.07.003URL [本文引用: 3]
DOI:10.1016/S0167-8809(99)00146-2URL [本文引用: 2]
[本文引用: 2]
DOI:10.1046/j.1365-2486.1998.00101.xURL [本文引用: 1]
DOI:10.1146/annurev.ecolsys.37.091305.110039URL [本文引用: 1]
DOI:10.1111/gcb.12938URLPMID:25846203 [本文引用: 1]

A key part of the uncertainty in terrestrial feedbacks on climate change is related to how and to what extent nitrogen (N) availability constrains the stimulation of terrestrial productivity by elevated CO2 (eCO2 ), and whether or not this constraint will become stronger over time. We explored the ecosystem-scale relationship between responses of plant productivity and N acquisition to eCO2 in free-air CO2 enrichment (FACE) experiments in grassland, cropland and forest ecosystems and found that: (i) in all three ecosystem types, this relationship was positive, linear and strong (r(2) = 0.68), but exhibited a negative intercept such that plant N acquisition was decreased by 10% when eCO2 caused neutral or modest changes in productivity. As the ecosystems were markedly N limited, plants with minimal productivity responses to eCO2 likely acquired less N than ambient CO2 -grown counterparts because access was decreased, and not because demand was lower. (ii) Plant N concentration was lower under eCO2 , and this decrease was independent of the presence or magnitude of eCO2 -induced productivity enhancement, refuting the long-held hypothesis that this effect results from growth dilution. (iii) Effects of eCO2 on productivity and N acquisition did not diminish over time, while the typical eCO2 -induced decrease in plant N concentration did. Our results suggest that, at the decennial timescale covered by FACE studies, N limitation of eCO2 -induced terrestrial productivity enhancement is associated with negative effects of eCO2 on plant N acquisition rather than with growth dilution of plant N or processes leading to progressive N limitation.
DOI:10.1016/j.envexpbot.2006.04.009URL [本文引用: 1]
[本文引用: 1]
[本文引用: 2]
[本文引用: 1]
DOI:10.1111/jac.2005.191.issue-2URL [本文引用: 1]
[本文引用: 1]
[本文引用: 3]
[本文引用: 1]
[本文引用: 3]
[本文引用: 1]
[本文引用: 1]
[本文引用: 1]
[本文引用: 2]
[本文引用: 2]
[本文引用: 1]
[本文引用: 1]
[本文引用: 1]
[本文引用: 1]
[本文引用: 1]
[本文引用: 1]
[本文引用: 1]
[本文引用: 1]
[本文引用: 1]
[本文引用: 1]
[本文引用: 1]
[本文引用: 1]
[本文引用: 2]
DOI:10.1007/s11104-006-9093-4URL [本文引用: 2]

A major uncertainty in predicting long-term ecosystem C balance is whether stimulation of net primary production will be sustained in future atmospheric CO2 scenarios. Immobilization of nutrients (N in particular) in plant biomass and soil organic matter (SOM) provides negative feedbacks to plant growth and may lead to progressive N limitation (PNL) of plant response to CO2 enrichment. Soil microbes mediate N availability to plants by controlling litter decomposition and N transformations as well as dominating biological N fixation. CO2-induced changes in C inputs, plant nutrient demand and water use efficiency often have interactive and contrasting effects on microbes and microbially mediated N processes. One critical question is whether CO2-induced N accumulation in plant biomass and SOM will result in N limitation of microbes and subsequently cause them to obtain N from alternative sources or to alter the ecosystem N balance. We reviewed the experimental results that examined elevated CO2 effects on microbial parameters, focusing on those published since 2000. These results in general show that increased C inputs dominate the CO2 impact on microbes, microbial activities and their subsequent controls over ecosystem N dynamics, potentially enhancing microbial N acquisition and ecosystem N retention. We reason that microbial mediation of N availability for plants under future CO2 scenarios will strongly depend on the initial ecosystem N status, and the nature and magnitude of external N inputs. Consequently, microbial processes that exert critical controls over long-term N availability for plants would be ecosystem-specific. The challenge remains to quantify CO2-induced changes in these processes, and to extrapolate the results from short-term studies with step-up CO2 increases to native ecosystems that are already experiencing gradual changes in the CO2 concentration.
DOI:10.1007/s004420000544URLPMID:28547240 [本文引用: 2]
[本文引用: 3]
[本文引用: 1]
[本文引用: 1]
[本文引用: 1]
[本文引用: 3]
[本文引用: 3]
[D].
[本文引用: 1]
[D].
[本文引用: 1]
[本文引用: 1]
[本文引用: 1]
[本文引用: 1]
[本文引用: 1]
[本文引用: 1]
[本文引用: 1]
[本文引用: 1]
[本文引用: 1]
[本文引用: 1]
[本文引用: 1]
[本文引用: 1]
[本文引用: 1]
[本文引用: 1]
[本文引用: 2]
[本文引用: 1]
DOI:10.1111/gcb.15211URLPMID:32614503 [本文引用: 1]

We assessed the response of soil microbial nitrogen (N) cycling and associated functional genes to elevated temperature at the global scale. A meta-analysis of 1,270 observations from 134 publications indicated that elevated temperature decreased soil microbial biomass N and increased N mineralization rates, both in the presence and absence of plants. These findings infer that elevated temperature drives microbially mediated N cycling processes from dominance by anabolic to catabolic reaction processes. Elevated temperature increased soil nitrification and denitrification rates, leading to an increase in N2 O emissions of up to 227%, whether plants were present or not. Rates of N mineralization, denitrification and N2 O emission demonstrated significant positive relationships with rates of CO2 emissions under elevated temperatures, suggesting that microbial N cycling processes were associated with enhanced microbial carbon (C) metabolism due to soil warming. The response in the abundance of relevant genes to elevated temperature was not always consistent with changes in N cycling processes. While elevated temperature increased the abundances of the nirS gene with plants and nosZ genes without plants, there was no effect on the abundances of the ammonia-oxidizing archaea amoA gene, ammonia-oxidizing bacteria amoA and nirK genes. This study provides the first global-scale assessment demonstrating that elevated temperature shifts N cycling from microbial immobilization to enhanced mineralization, nitrification and denitrification in terrestrial ecosystems. These findings infer that elevated temperatures have a profound impact on global N cycling processes with implications of a positive feedback to global climate and emphasize the close linkage between soil microbial C and N cycling.
[D].
[本文引用: 1]
[D].
[本文引用: 1]
[本文引用: 1]
[本文引用: 1]
[本文引用: 1]
[本文引用: 1]
[本文引用: 1]
[本文引用: 1]
[本文引用: 1]
[本文引用: 1]
[本文引用: 1]
[本文引用: 1]
[本文引用: 1]
URLPMID:31554024 [本文引用: 1]
[本文引用: 1]
[本文引用: 1]
URLPMID:16123304 [本文引用: 1]
DOI:10.1126/science.1113435URLPMID:16099988 [本文引用: 1]

Microorganisms living in anoxic rice soils contribute 10 to 25% of global methane emissions. The most important carbon source for CH4 production is plant-derived carbon that enters soil as root exudates and debris. Pulse labeling of rice plants with 13CO2 resulted in incorporation of 13C into the ribosomal RNA of Rice Cluster I Archaea in the soil, indicating that this archaeal group plays a key role in CH4 production from plant-derived carbon. This group of microorganisms has not yet been isolated but appears to be of global environmental importance.
[本文引用: 1]
DOI:10.1007/s10532-009-9261-3URLPMID:19381451 [本文引用: 1]

BTEX compounds such as benzene are frequent soil and groundwater contaminants that are easily biodegraded under oxic conditions by bacteria. In contrast, benzene is rather recalcitrant under anaerobic conditions. The analysis of anoxic degradation is often hampered by difficult sampling conditions, limited amounts of biomass and interference of matrix compounds with proteomic approaches. In order to improve the procedure for protein extraction we established a scheme consisting of the following steps: dissociation of cells from lava granules, cell lysis by ultrasonication and purification of proteins by phenol extraction. The 2D-gels revealed a resolution of about 240 proteins spots and the spot patterns showed strong matrix dependence, but still differences were detectable between the metaproteomes obtained after growth on benzene and benzoate. Using direct data base search as well as de novo sequencing approaches we were able to identify several proteins. An enoyl-CoA hydratase with cross species homology to Azoarcus evansii, is known to be involved in the anoxic degradation of xenobiotics. Thereby the identification confirmed that this procedure has the capacity to analyse the metaproteome of an anoxic living microbial community.