Lijun Fang, Hongkun Liu,Yan Bian,Yudong Liu , Yali Yang
(School of Energy Power & Mechanical Engineering, North China Electric Power University,Baoding 071003, Hebei, China)
Abstract:
In order to study the effects of nanoparticles on the CO2 absorption in ammonia, nanofluids with different ammonia concentration and different nanoparticle solid loading were prepared by a two-step method. The nanofluids-enhanced gas absorption test devices were also established. The CO2 absorption in TiO2, CuO, SiO2 nanofluids, which nanoparticles solid loading were 1.0-8.0 g/L, was tested respectively. In comparison with the blank absorption experiment, the effects of nanoparticle solid loading, nanoparticle types, ammonia concentration on the removal efficiency and removal rate were obtained. Experimental results show that adding nanoparticles can enhance the removal efficiency and removal rate, which increase first and then decrease with the increase of nanoparticle solid loading, and there exists an optimum solid loading of TiO2 nanoparticles. The effect of SiO2 nanofluid is inhibitory on the reaction. The enhancement factor of CuO nanofluid is always hovering around 1, which does not show the obvious enhancement or inhibition on the reaction. The optimum solid loading decreases gradually with the increase of ammonia concentration. In addition, according to the experimental results, the mechanism of enhanced absorption was analyzed theoretically.
Key words: nanofluids bubble absorption ammonia CO2 capture enhanced absorption
DOI:10.11916/j.issn.1005-9113.15246
Clc Number:TQ021.4
Fund:
Lijun Fang, Hongkun Liu, Yan Bian, Yudong Liu, Yali Yang. Experimental Study on Enhancement of Bubble Absorption of Gaseous CO2 with Nanofluids in Ammonia[J]. Journal of Harbin Institute of Technology, 2017, 24(2): 80-86. DOI: 10.11916/j.issn.1005-9113.15246.

Fund Sponsored by the Hebei Natural Science Foundation of Hebei Province of China (Grant No.B2014502056) Corresponding author Yan Bian, E-mail:by19910330@126.com Article history Received: 2015-08-26
Contents Abstract Full text Figures/Tables PDF
Experimental Study on Enhancement of Bubble Absorption of Gaseous CO2 with Nanofluids in Ammonia
Lijun Fang, Hongkun Liu, Yan Bian


School of Energy Power & Mechanical Engineering, North China Electric Power University, Baoding 071003, Hebei, China
Received: 2015-08-26
Fund: Sponsored by the Hebei Natural Science Foundation of Hebei Province of China (Grant No.B2014502056)
Corresponding author: Yan Bian, E-mail:by19910330@126.com
Abstract: In order to study the effects of nanoparticles on the CO2 absorption in ammonia, nanofluids with different ammonia concentration and different nanoparticle solid loading were prepared by a two-step method. The nanofluids-enhanced gas absorption test devices were also established. The CO2 absorption in TiO2, CuO, SiO2 nanofluids, which nanoparticles solid loading were 1.0-8.0 g/L, was tested respectively. In comparison with the blank absorption experiment, the effects of nanoparticle solid loading, nanoparticle types, ammonia concentration on the removal efficiency and removal rate were obtained. Experimental results show that adding nanoparticles can enhance the removal efficiency and removal rate, which increase first and then decrease with the increase of nanoparticle solid loading, and there exists an optimum solid loading of TiO2 nanoparticles. The effect of SiO2 nanofluid is inhibitory on the reaction. The enhancement factor of CuO nanofluid is always hovering around 1, which does not show the obvious enhancement or inhibition on the reaction. The optimum solid loading decreases gradually with the increase of ammonia concentration. In addition, according to the experimental results, the mechanism of enhanced absorption was analyzed theoretically.
Key words: nanofluids bubble absorption ammonia CO2 capture enhanced absorption
1 IntroductionIn recent years, the greenhouse effect has caused a variety of climate issues, which has aroused wide concern. CO2 is one of the main greenhouse gases that cause global warming[1-2], so CO2 emissions reduction is imperative. According to international energy data, the CO2 emissions of China is 8.106 109 t and CO2 emissions from the consumption of coal of China is 6.153 109 t in 2012[3]. However, in the coal fired power plant, CO2 partial pressure is lower, SOx, NOx and heavy metals in the flue gas increase the difficulty of removing CO2. China has promised to cut its CO2 emissions per unit of GDP by 40%-45% below 2005 levels by 2020[4]. So the chemical absorption method is the best choice for the power plant that has been built. At present, a lot of researches have been done in the domestic and overseas and the technology has been basically mature, but there have been many deficiencies in the method of alcohol amine[5]. Some scholars proposed to use ammonia as the absorbent to capture CO2, and the research showed that the removal efficiency of ammonia can reach 100%, the absorptive capacity and the renewable energy consumption are better than MEA[6-7]. However, ammonia is volatile, leading to ammonia escape and increasing the cost of removal, according to the existing research, the mass transfer process can be enhanced by the addition of fine particles in the reaction solution, fine particles can reduce the mass transfer resistance and increase the mass transfer rate, besides, it can also reduce the equipment size and save investment in the same working conditions.
The concept of nanofluids was first proposed by Choi[9] in 1995. Dagaonkar[10] found that micron sized TiO2 particles can enhance the speed of water, sixteen alkanes and sunflower oil to absorb CO2. Kim et al. found that nanoscale Cu, CuO and Al2O3 particles strengthened the ammonia absorption process. Pineda[13] made the methanol as the base fluid, finding that TiO2, Al2O3 nanoparticles can enhance mass transfer. Lu [14] found that activated carbon in water solution had significant effect on the reaction enhancement, but in cyclohexane solution, Al2O3, silica gel enhanced CO2 absorption. Ma[15] found that activated carbon and nano SiO2 can promote gas absorption to reach saturation in advance, it was also concluded that the material not containing pore cannot enhance mass transfer by the experiment which used glass beads as additives. Tang [16]used bubble reactor, took C2H5OH as the base fluid, added Al2O3, MgO, TiO2 and SiO2 nanoparticles respectively to study the strengthening effect of particles on the absorption and the strengthening mechanism of particles was analyzed.
In the paper, a CO2 bubble test bench is built to explore the effect of nano SiO2, TiO2 and CuO on the CO2 absorption in aqueous solution. The objective of the paper is to find out the effects of nanoparticle solid loading, nanoparticle types, ammonia concentration on the removal efficiency and removal rate.
2 Preparations of Nanofluids2.1 ReagentReagent: ammonia, mass fraction is 25%-28%, Tianjin Chemical Co., Ltd.; Carbon dioxide gas, purity is 99.99%, nitrogen, purity is 99.99%, Baoding North Special Gas Co., Ltd.; Nanoparticles (SiO2, TiO2 and CuO), provided by the Nanjing Aipurui Nano Material Co., Ltd. Figs. 1(a), (b) are the TEM photo of TiO2, CuO nanoparticles respectively that particle size is 15 nm.
Figure 1
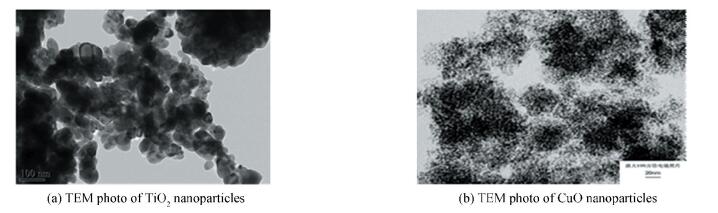
2.2 Preparation MethodFrom the experiment of Li [17], in the lower mass fraction of nanofluids, the dispersion stability of nanofluids can be obtained by mechanical dispersion. In the paper, the 'two step method' is used to prepare the nanofluids. First of all, to weigh nano CuO, SiO2 and TiO2 by balance, to measure some water needed, then to mechanically disperse for 20 min and add a certain amount of ammonia, then to continue to mechanically disperse for 10 min to prepare nanofluids of different mass fraction.
In order to investigate the stability of nanofluids, let the different kinds of nanoparticles of nanofliuds and the different solid loading of nanofliuds static for 12 h. Fig. 2 are the photos that the nanofluids static 12 h ago and later. No aggregation and precipitation appeared to the eyes, which showed that the stability of the nanofluids was good, and the experimental results were basically achieved.
Figure 2
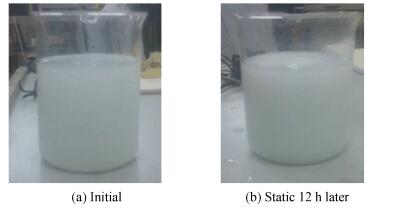
3 Experimental Schematic and Principle3.1 Experimental SchematicExperimental schematic is shown in Fig. 3, mainly including the bubble absorption tower, flue gas distribution system, exhaust measurement and processing system.
Figure 3
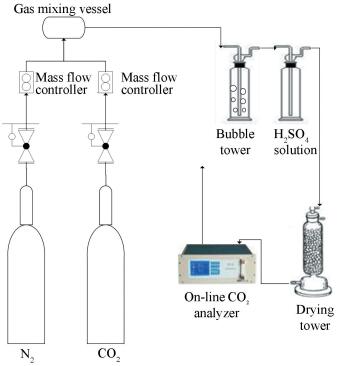
3.2 Experimental Conditions and Experimental ProcedureExperimental conditions are shown in Table 1.
表 1
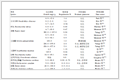
298 0.12 400 15, 60 TiO2, CuO, SiO2 1.0-8.0 0.5, 0.8, 1
Table 1 Experimental conditions
Experimental procedure:
1) To calculate the amount of nanoparticles, ammonia and distilled water needed for the preparation of nanofluids before the experiment, to weigh the quality of nanoparticles and add it to distilled water, to mechanically disperse for 20 min, then to add a certain amount of ammonia, then to continue to mechanically disperse for 10 min to prepare nanofluids of different mass fraction.
2) To open analyzer and preheat 30 min, to purge the whole system by N2, when the number of flue gas analyzer fall to zero, to fill the mixed bottle with nitrogen and carbon dioxide in a proportion and put them into the analyzer after mixing evenly until the number reaches the preset value and keeps stable.
3) To put the prepared nanofluids into reaction tower, at the same time, to start counting, and count every 5 s and stop counting until the number no longer reduces. To place the prepared nanofluids in a sealed container, standing after 48 h, without a lot of agglomeration, sedimentation phenomenon, the stability of nanofluids is better.
In order to reduce the impact of accidental error, the experiment is carried out for 5 times, and then the average value is obtained.
3.3 Experiment PrincipleAbsorbing CO2 by ammonia water is a typical chemical reaction of gas-liquid two phase. Its basics on reaction equation are as follows:
${\rm{C}}{{\rm{O}}_{2, {\rm{G}}}} \to {\rm{C}}{{\rm{O}}_{2, {\rm{L}}}} + {{\rm{H}}_{\rm{A}}}$ (1)
${\rm{C}}{{\rm{O}}_2} + {{\rm{H}}_{\rm{2}}}{\rm{O}} \leftrightarrow {\rm{HCO}}_3^ - + {{\rm{H}}^ + }$ (2)
${\rm{N}}{{\rm{H}}_3} + {{\rm{H}}_{\rm{2}}}{\rm{O}} \leftrightarrow {\rm{NH}}_4^ + + {\rm{O}}{{\rm{H}}^ - }$ (3)
${\rm{HCO}}_3^ - \leftrightarrow {\rm{CO}}_2^{2 - } + {{\rm{H}}^ + }$ (4)
${{\rm{H}}_{\rm{2}}}{\rm{O}} \leftrightarrow {\rm{O}}{{\rm{H}}^ - } + {{\rm{H}}^ + }$ (5)
${\rm{N}}{{\rm{H}}_3} + {\rm{HCO}}_3^ - \leftrightarrow {\rm{N}}{{\rm{H}}_2}{\rm{CO}}{{\rm{O}}^ - } + {{\rm{H}}_{\rm{2}}}{\rm{O}}$ (6)
In the process of absorbing CO2 in ammonia solution, reaction (1) is CO2 gas absorbed by liquid phase, which occurs mainly in the gas-liquid contact interface. Therefore, in order to increase the mass transfer rate, on the one hand, the surface renewal rate can be speeded up by physical and chemical methods; on the other hand, gas-liquid contact area can be increased. The main reaction step (6) is the reversible ion reaction. The absorption rate of HCO3- is increased by increasing the concentration of CO2, so the reaction (6) is moved to the right direction, which enhances the whole reaction.
3.4 Experiment Result ProcessingIn the experiment, the initial volume concentration of CO2 in the flue gas (the volume concentration of carbon dioxide at the entrance of the absorber) is known, and it is denoted by cin. After the washing, carbon dioxide concentration, which is in the flue gas from the top of the absorber, can be measured by the infrared carbon dioxide detector, and it is denoted by cout. Due to a semi continuous absorption process, the removal efficiency of CO2 is gradually decreased with the increase of CO2 in the absorber. In comparison of the removal efficiency, besides considering the real-time removal efficiency, the average removal efficiency of ammonia solution in a period of time is also given attention, because the latter can reflect the removal effect of the absorbent more better, so the paper is calculated by the latter, and cout is the volume concentration when the reaction reaches the final equilibrium. Due to the nitrogen is not involved in the reaction, the amount of reaction is constant, and the efficiency of carbon dioxide removal is obtained according to nitrogen conservation. The calculation formulas are shown as follows.
According to N2 conservation, it can be obtained:
${Q_{{\rm{in}}}}\left( {1 - {c_{{\rm{in}}}}} \right) = {Q_{{\rm{out}}}}\left( {1 - {c_{{\rm{out}}}}} \right)$ (7)
${Q_{{\rm{out}}}} = \frac{{{Q_{{\rm{in}}}}\left( {1 - {c_{{\rm{in}}}}} \right)}}{{1 - {c_{{\rm{out}}}}}}$ (8)
The removal efficiency of CO2:
$\eta = \left( {1 - \frac{{{Q_{{\rm{out}}}}{c_{{\rm{out}}}}}}{{{Q_{{\rm{in}}}}{c_{{\rm{in}}}}}}} \right) \times 100\% $ (9)
Combination of Eqs. (8) and (9) leads to Eq. (10):
$\eta = \frac{{{c_{{\rm{in}}}} - {c_{{\rm{out}}}}}}{{{c_{{\rm{in}}}}\left( {1 - {c_{{\rm{out}}}}} \right)}} \times 100\% $ (10)
where Qin is bubble column inlet gas flow rate, L/min; Qout is bubble column outlet gas flow, L/min; cin is concentration of CO2 of bubble column inlet, %; cout is concentration of CO2 of bubble column outlet, %.
Removal efficiency enhancement factor E is defined as the ratio of the removal efficiency of nanoparticles experiment and the blank experiment.
$E = \frac{{{\eta _{\rm{n}}}}}{{{\eta _0}}} \times 100\% $ (11)
where ηn is removal efficiency of CO2 by adding nanoparticles; η0 is removal efficiency of CO2 by not adding nanoparticles. E>1, illustrating that particles addition has the effect of strengthening the absorption. E=1, illustrating that particles addition has no effect on the absorption. E < 1, illustrating that particles addition can inhibit the absorption. In order to eliminate the impact of ammonia concentration on E, ammonia is formulated in a sealed container and measuring the pH value before the reaction to ensure the same conditions for the same ammonia concentration.
4 Experimental Results and Analysis4.1 Effect of Nanoparticle Types and Solid Loading on EExperiments on the bubble absorption of nanofluid that ammonia mass fraction is 1.0%, CuO, TiO2, SiO2 particle solid loading are 1.0, 2.0, 3.0, 4.0, 5.0 g/L respectively. The enhancement factors in different conditions are shown in Fig. 4.
Figure 4
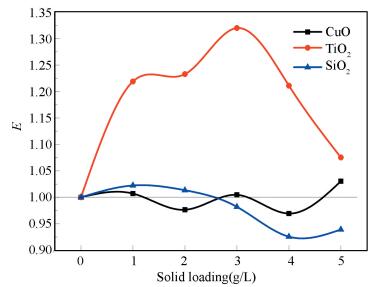
Fig. 4 shows that when the fluid is SiO2 nanofluid, the enhancement factor is slightly more than 1 only in 1.0, 2.0 g/L, less than 1 for the others. Feng[18]got the similar result. When the fluid is CuO nanofluid, the enhancement factor is always hovering around 1, and there is no obvious enhancement or inhibition effect, getting the similar result with Kim[19-20]. When the fluid is TiO2 nanofluid, its enhancement factor has been greater than 1. The result is similar with Dagaonkar[10] and LU Su-min[21].
Due to the polarity of SiO2 with hydrophilic, it would interact with water and form the nanofluid with a certain viscosity, so the resistance of CO2 through the liquid membrane is increased, and the number of CO2 molecules in liquid phase through the liquid membrane is reduced in the same time, which leads to the decrease of removal efficiency. The viscosity of fluid increases with the increase of particle size, and the removal efficiency decreases with the increase of particle size. Nano CuO is easy to combine with ammonia to form a copper ammonia complex ion, which leads to the loss of effect of nanoparticles on the reaction, at the same time, the formation of copper ammonia complex ion has no effect on the viscosity of solution, so nano CuO does not show obvious enhancement or inhibition on the reaction.
Studies indicate that TiO2 particles have a high adsorption affinity for certain gases (e.g. H2, CO, CO2, NH3, etc.)[22]. Based on Refs.[23-27], CO2 concentration gradient of the gas-liquid interface is significantly increased, the gas-liquid two-phase interface in thermodynamic non-equilibrium state. This imbalance state will push to restore the gas-liquid mass transfer equilibrium of the entire system interface direction, TiO2 particles that adsorbing CO2 get into the liquid body, a new CO2 molecules will complementary to the gas-liquid interface, the absorption of ammonia to CO2 is strengthened, TiO2 particles in the process adsorption of CO2 reach saturation easily, TiO2 particles have no ability to adsorb CO2. With the addition of TiO2 particles increasing, solution viscosity increases, a flat will be formed between the particles, the resistance is increasing, therefore the effect of strengthening gradually decreases.
4.2 Effect of Ammonia Concentration on EExperimental conditions: ammonia mass fraction is 0.5%, 0.8% and 1.0% respectively.TiO2 particles are solid loading 1.0-8.0 g/L respectively and a blank absorption experiment.
As shown in Fig. 5, the trend of enhancement factors of different ammonia concentrations increases first and then decreases, but the optimum solid content is different, it decreases with the increase of ammonia concentration, and the final enhancement factor is more than 1.
Figure 5
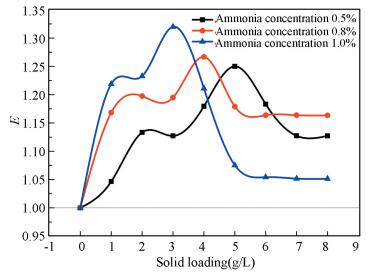
The reasons of different optimum solid loading may be: the number of ammonia molecules in the liquid phase increases with the increase of ammonia concentration. Under the same flue gas quantity, CO2 in flue gas through liquid phase is more likely to be captured by ammonia, and TiO2 particles are reduced when it reaches the maximum removal efficiency.
4.3 Effect of Nano Particle Type on Removal RateExperiments on the bubble absorption of nanofluid that ammonia mass fraction is 1%, CuO, TiO2, SiO2 particles are 1.0, 5.0 g/L respectively. Fig. 6 is the curve of CO2 absorption rate changing with time in the first 140 s of the reaction.
Figure 6
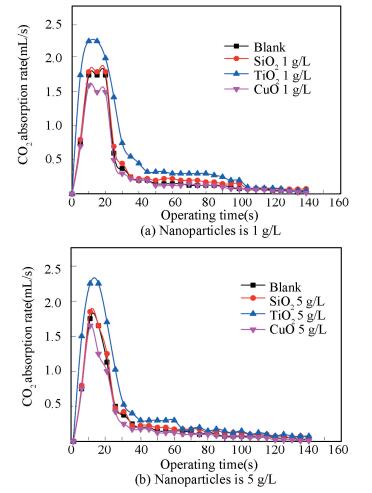
From Fig. 6, the absorption rate of TiO2 nanofluid is larger than that of the blank one, the absorption rate of CuO nanofluid is the same as that of the blank and the absorption rate of SiO2 nanofluid is less than that of the blank. Lee[28] thought absorption was strengthened because of Marangoni convection caused by the nanoparticles. The reason for Marangoni is: due to the difference of viscosity between the fluid and interfacial fluid at the junction of two phase mass transfer, tension gradient emerges at the interface, and then the stress of the interface is created. The relationship between the stress and the shear stress of interface is shown as follows.
$\left| {\frac{{\partial \sigma }}{{\partial x}}} \right| = \mu \frac{{\partial {\sigma _{{\rm{surf}}}}}}{{\partial y}} + {\mu _G}\frac{{ - \partial {\sigma _{{\rm{surf, G}}}}}}{{\partial y}}$
where μ is liquid viscosity, Pa·s; μG is gas viscosity, Pa·s.
The gas viscosity is far less than that of the liquid viscosity, so the gas phase can be neglected relative to the above equilibrium. Thus, the stress of interface balances no longer as long as the absolute value of surface tension gradient is more than a critical value, which will lead to flow. The convection produced by the hydrodynamics instability that is induced by the surface tension gradient is called Marangoni convection, as shown in Fig. 7.The phase transfer process can be enhanced by the interfacial convection.
Figure 7

In this experiment, due to the difference of viscosity between ammonia and ammonium bicarbonate solution, the Marangoni flow will be emerging. According to the solute permeation theory, the actual mass transfer coefficient kc is shown as follows.
${k_{\rm{c}}} = 2\sqrt {\frac{D}{{\pi t}}} $
where kc is the mass transfer coefficient, m/s; D is the diffusivity coefficient, m/s2; t is the time, s.
Based on Refs.[29-30], combining with the analysis of the experimental results, it can obtain that:
The reaction between ammonia and CuO is easily carried out, which produces blue flocculent precipitate, is unable to obtain satisfactory results; the polar SiO2 is hydrophilic, will interact with water to form a nanofluid that has a certain viscosity, the effects of Brownian movement and Marangoni can be ignored, but for TiO2:
1) The micro-convection is induced by Brownian movement, which is generated by TiO2 nanofluid will speed up the update on interface, shuttle mechanism of TiO2 particles is accelerated, so the diffusion coefficient increases, the time CO2 stays at surface will be shorter, so that the mass transfer rate increases.
2) Due to the adsorption and shuttle mechanism of TiO2 nanoparticles, interfacial force is no longer balanced, and the state is instability, which will lead to Marangoni flow. Its performance in this absorption process is a positive effect, which promotes interfaces update, and the absorption rate increases.
3) The micro-convection is induced by Brownian movement and Marangoni flow generated by TiO2 will prevent accumulation of bubbles, increase gas holdup and the effective area of mass transfer, so the mass transfer rate increases.
4.4 Effect of Nanoparticle Addition on Removal RateExperimental conditions: ammonia mass fraction is 1%, TiO2 particles are 1.0, 2.0, 3.0, 4.0, 5.0 g/L respectively and a blank absorption experiment.
As shown in Fig. 8, the removal rate increases first and then decreases with the increase of nanoparticle additions, it is always greater than the blank solution experiment. The removal rate reaches the maximum when the additive amount is 3.0 g/L. Reasons are possible as follows.
Figure 8
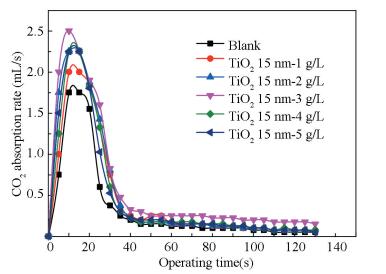
1) With the increase of nanoparticles additions, the degree of agglomeration of nanoparticles will be exacerbated, thereby weaken the Brownian movement, decrease the molecular diffusion coefficient and mass transfer rate.
2) Gas-liquid contact interface becomes unstable, easy to be pulled broken, resulting in reducing or even disappearing the Marangoni effect of the contact surface, declining the surface renewal rate and dropping the removal rate.
3) The increase of the content of TiO2 nanoparticles and the viscosity of the solution result in TiO2 which adsorbs CO2 difficult to move out from the contact surface quickly, the particles lost adsorbing capacity of CO2 to form a flat in the gas-liquid contact surface which obstacles CO2 to get into the liquid, therefore surface renewal rate decreases and removal rate drops.
5 Conclusions1) The addition of nanoparticles has a strengthening effect on the gas-liquid mass transfer process with chemical reactions, which is related to nanoparticles and the properties of solvents.
2) The nanoparticles with selective adsorption and hydrophobic solvent can enhance the gas-liquid mass transfer process with chemical reactions. The strengthening effect increases first and then decreases with the increase addition of nanoparticles, that is, the content of nano TiO2 has an optimum solid content for the CO2 capture.
3) The nanoparticles, which have no adsorption to the gas solute but have affinity solvent, can inhabit the gas-liquid mass transfer process with chemical reactions.
4) Mechanism nanoparticles reinforced liquid mass transfer process can be summarized as follows: Brownian movement and Marangoni convection generated by TiO2 nanofluid make the gas-liquid two-phase interface updated rate faster; the adsorption of TiO2 nanoparticles affects thermodynamic equilibrium of gas-liquid interface and this imbalance strengthens the absorption process; the convection effect on the gas-liquid interface strengthens shuttle mechanism of TiO2 particles, thereby strengthens mass transfer process.
References
[1]Ma Shuangchen, Wang Mengxuan, Sun Yunxue, et al. Efficiency comparison of CO2 removal respectively with ammonia solution and MEA.Journal of Chinese Society of Power Engineering, 2012, 32(1): 52-58.(

[2]Shi Xiaomei, Liao Zuwei, Wang Jingdai, et al. Graphical optimization approach to carbon-constrained energy sector planning.Journal of Chemical Industry and Engineering, 2009, 60(5): 1237-1244.(

[3] U.S. Energy Information Administration. http://www.eia.Gov/cfapps/ipdbproject/. (

[4]Ma Shuangchen, Lv Yukun, Lu Tongchang, et al. Experimental research on CO2 capture from simulated flues gas using ammonia solution in packed towers.Proceedings of the CSEE, 2013, 33(26): 27-32.(

[5]Ma Shuangchen, Sun Yunxue, Zhao Yi, et al. Experimental and mechanism research on CO2 capture from simulating flue gas using ammonia solution.Journal of Chemical Industry and Engineering, 2011, 69(12): 1469-1474.(

[6]Bai H, Yeh A C. Removal of CO2 greenhouse gas by ammonia scrubbing.Industrial and Engineering Chemistry Research, 1997, 36(6): 2490-2493.DOI:10.1021/ie960748j(

[7] Wolf M, Braustch A, Gernert J, et al. Outlook on post combustion CO2 capture in ALSTOM gas turbine plants.Proceedings of Power Gen Europe.Cologne, Germany, 2008. (

[8]Jiang Jiazong, Zhao Bo, Cao Meng, et al. Overview of studies on gas-liquid mass transfer enhancement in the presence of fine particles.Proceedings of the CSEE, 2014, 34(5): 786-790.(

[9] Choi S U S.Enhancing thermal conductivity of fluids with Nanoparticles. Proceedings of the 1995 ASME International Mechanical Engineering Congress and Exposition. San Francisco, CA, 1995.99-105. (

[10]Dagaonkar M V, Heere H J, Beenackers A A C M. The application of fine TiO2particles for enhanced gas absorption.Chemical Engineering Journal, 2003, 92(1/2/3): 151-159.(

[11]Kim J K, Jung J Y, Kang Y T. The effect of nanoparticles on the bubble absorption performance in a binary nanofluids.International Journal of Refrigeration, 2006, 29(1): 22-29.DOI:10.1016/j.ijrefrig.2005.08.006(

[12]Kim J K, Jung J Y, Kang Y T. Absorption performance enhancement by nano-particles and chemical surfactants in binary nanofluids.International Journal of Refrigeration, 2007, 30(1): 50-57.DOI:10.1016/j.ijrefrig.2006.04.006(

[13]Pineda I T, Choi C K, Kang Y T. CO2 gas absorption by CH3OH based nanofluids in an annular contactor at low rotational speeds.International Journal of Greenhouse Gas Control, 2014(23): 105-112.(

[14]Lu Sumin, Ma Youguang, Shen Shuhua, et al. Effect of fine solid particles on gas absorption rate of CO2.Journal of Chemical Engineering of Chinese Universities, 2008, 2(2): 357-360.(

[15] Ma Lian. Influence of Adding Solid Particles on Absorption of CO2 in Aqueous Monoethanolamine (mea) Solution.Harbin:Harbin Institute of Technology, 2008. (

[16]Tang Zhongli, Peng Linming, Zhang Shuyang. Experiment on enhancement of bubble absorption of gaseous CO2 with nanofluids.Journal of Tianjin University, 2012, 45(6): 534-539.(

[17]Li Shuhong, Ding Yi, Du Kai. CO2 bubble absorption performance enhancement by TiO2 nanoparticles in MDEA solution.Jurnal of Southeast University (Natural Science Edition), 2013, 43(4): 830-834.(

[18]Feng Xuemei, Johnson D W. Mass transfer in SiO2 nanofluids: A case against purported nanoparticle convection effects.International Journal of Heat and Mass Transfer, 2012(55): 3447-3453.(

[19]Kim J K, Jung J Y, Kang Y T. The effect of nano-particles on the bubble absorption performance in a binary nanofluid.International Journal of Refrigeration, 2006, 29(1): 22-29.DOI:10.1016/j.ijrefrig.2005.08.006(

[20]Kim J K, Jung J Y, Kang Y T. Absorption performance enhancement by nano-particles and chemical surfactants in binary nanofluids.International Journal of Refrigeration, 2007, 30(1): 50-57.DOI:10.1016/j.ijrefrig.2006.04.006(

[21]Lu Sumin, Ma Youguang, Shen Shuhua. Enhancement of gas absorption in slurries of TiO2 in water.Journal of Tianjin Polytechnic University, 2007, 26(1): 42-45.(

[22]Linsebigler A L, Guangquan L, Yates Y T. Photocatalysis on TiO2 surfaces: Principles, mechanisms and selected results.Chem Rev, 1995(95): 735.(

[23]Jiang Jiazong, Zhao Bo, Cao Meng, et al. Fine particle enhanced oxygen absorption.Journal of Chemical Industry and Engineering, 2013, 64(4): 1306-1311.(

[24]Karve S, Juvekar V A. Gas absorption into slurries containing fine catalyst particles.Chem Eng Sci, 1990, 45(3): 587-594.DOI:10.1016/0009-2509(90)87003-B(

[25]Lu Sumin, Ma Youguang, Shen Shuhua, et al. Effect of fine solid particles on gas absorption rate of CO2.Journal of Chemical Engineering of Chinese Universities, 2008, 22(2): 356-360.(

[26]Cheng Hong, Zhou Ming, Zhang Ying, et al. Enhancement of fine adsorbent particles gas-liquid mass transfer.Journal of Chemical Industry and Engineering, 1999, 50(6): 772-777.(

[27]Li Xin, Xu Chunjian, Zhou Ming. A study of gas-liquid mass transfer enhancement of adsorbent particle in slurry bubble columns.Transactions of Beijing Institute of Technology, 2008, 28(3): 263-266.(

[28] Lee J K, Jeong M Y. Absorption heat and mass transfer enhancement in binary (NH3/H2O) nanofluids. Cryogenics and Refrigeration, Proceeding of ICCR2008. Beijing: Science Press, 2008.580. (

[29]Sheng Wei, Wu Weidong, Zhang Hua, et al. Enhancing influence of Al2O3 nano-particles on ammonia bubble absorption process.Journal of Chemical Industry and Engineering (China), 2008, 59(11): 2762-2767.(

[30]Sheng Wei, Wu Weidong, Zhang Hua, et al. Mechanism and influence factors of nano-particles enhancing ammonia bubble absorption performance.Journal of Chemical Engineering of Chinese Universities, 2011, 25(1): 30-36.(
