With great achievements in efficiency, stability, and large-scale preparation of perovskite solar cells (PSCs), the commercialization of PSC is ongoing, but there is still an issue on lead toxicity. Although lead content in the device is low, the water solubility of lead salts leads to potential environmental pollution. At present, the non-lead perovskites studied include: divalent metal perovskite (e.g., Sn2+, Ge2+, Cu2+), trivalent metal perovskite (e.g., Bi3+, Sb3+, In3+), tetravalent metal double perovskite (e.g., Sn4+, Pd4+, Ti4+, Pt4+) and mono-trivalent mixed double perovskite (e.g., Ag+ and Bi3+, Ag+ and In3+, Ag+ and Sb3+). Their properties are summarized in Table 1. Since the first report on non-lead double perovskite Cs2AgBiBr6 in 2016[1], this material has caused extensive research in optoelectronic devices because of its long carrier lifetime[1] and good stability[2]. However, owing to its wide and indirect bandgap (Eg) (~2.0 eV), its light absorption range is narrow, which limits its application in photovoltaics. At present, the power conversion efficiency (PCE) of Cs2AgBiBr6 device is ~3%[3]. To enhance PCE, the Eg needs to be narrowed. The efforts in this area focus on adjusting chemical composition and physical structure (Fig. 1) to tune Eg.
Structure formula | Representative | Advantage | Disadvantage |
AB(II)X3 | FASnI3 | High absorption, high mobility | Unstable |
A2B(IV)X6 | Cs2SnI6 | Stable, suitable Eg | Defect |
A3B(III)2X9 | Cs3Bi2I9 | Stable | Wide Eg, defect |
A2B(I)B(III)X6 | Cs2AgBiBr6 | Stable | Wide Eg |
AaB(III)bXa+3b | Ag3BiI6 | High absorption | Phase separation, defect |
Table1.
Properties of lead-free perovskites.
Table options
-->

Download as CSV
Structure formula | Representative | Advantage | Disadvantage |
AB(II)X3 | FASnI3 | High absorption, high mobility | Unstable |
A2B(IV)X6 | Cs2SnI6 | Stable, suitable Eg | Defect |
A3B(III)2X9 | Cs3Bi2I9 | Stable | Wide Eg, defect |
A2B(I)B(III)X6 | Cs2AgBiBr6 | Stable | Wide Eg |
AaB(III)bXa+3b | Ag3BiI6 | High absorption | Phase separation, defect |

class="figure_img" id="Figure1"/>
Download
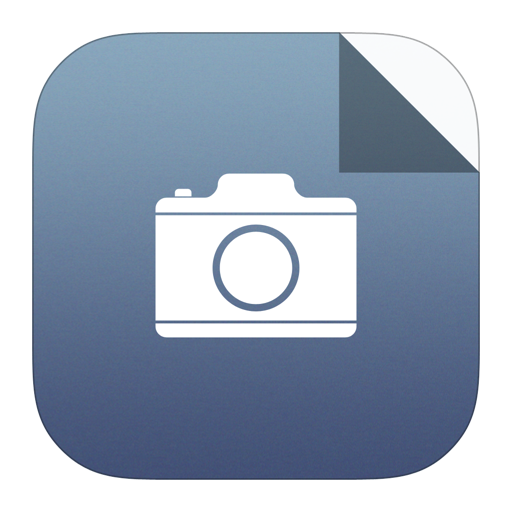
Larger image
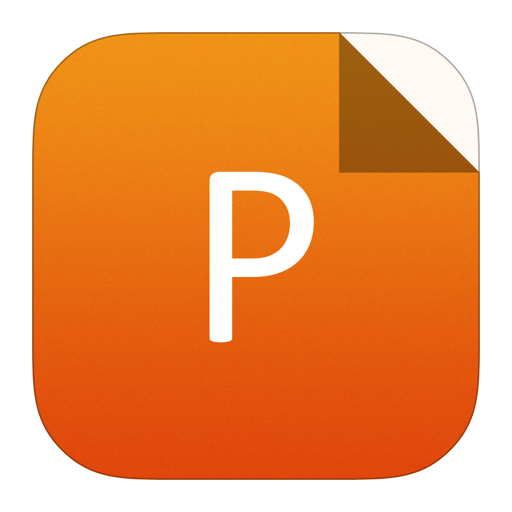
PowerPoint slide
Figure1.
(Color online) Tuning Eg of double perovskites.
A common attempt to tune the Eg of double perovskite is to adjust the chemical composition of compound A2B(I)B(III)X6.
A-site. Doping alkali metal ions like Rb+, K+, and Na+ made little effect on Eg[4]. Chen et al.[5] introduced Rb+ into Cs2AgBiBr6 to form (Cs1–xRbx)2AgBiBr6 and effectively passivated the defects of double perovskite, thus increasing PCE to 1.5%. But this doping could not significantly change the Eg. When doping A-site to change the dimension of perovskite from 3D to 2D, the Eg and electronic structure can be tuned. In 2019, Mitzi et al.[6] reported that a stable 2D double perovskite [AE2T]2AgBiI8 was formed by using multifunctional organic molecules with a direct Eg of 2.01 eV.
X-site. For lead-based perovskites, the Eg decreases with the increasing of halogen atom radius, and similar results are also observed in double perovskites. The introduction of Cl– can greatly increase Eg, while I– doping can significantly reduce Eg, which is 2.77 eV (X = Cl), 1.95 eV (X = Br), and 1.75 eV (X = I) for Cs2AgBiX6[7]. When X site is I, the stability for double perovskite decreases. It is difficult to prepare Cs2AgBiI6 experimentally, because the formation energy of Cs3Bi2I9 is lower. As reported by Ma et al.[8], the Eg of Cs2NaBiI6 was relatively narrow (1.66 eV), but it can easily decompose into Cs3Bi2I9, yielding a 0.42% PCE.
B-site. The structure of double perovskite consists of B(I)X6 and B(III)X6 with alternating octahedrons connected by vertices. Substituting B-site elements can tune Eg. Karunadasa et al.[9] found that doping low content of toxic Tl (Tl+, Tl3+) into Cs2AgBiBr6 to form Cs2(Ag1–aBi1–b)TlxBr6 (0.003 < x = a + b < 0.075) can significantly reduce its Eg to 1.40 eV for Cs2(Ag1–aBi1–b)Tl0.075Br6. Replacing Ag+ and Bi3+ by Tl+/Tl3+ yielded defects, leading to band-edge reconstruction. Using unstable Sn2+ (can be oxidized to Sn4+) to replace both Ag+ and Bi3+ can also reduce Eg to 1.71 eV (indirect) and 1.48 eV (direct)[10]. These results indicate that the band-edge reconstruction caused by the lattice distortion due to B-site ion doping can tune the Eg of double perovskite. Mitzi et al.[11] reported that the use of trivalent metal In3+ or Sb3+ can also significantly tune Eg, which could be increased to 2.4 eV by replacing 75% of Bi3+ with In3+. While replacing 37.5% Bi3+ with Sb3+ could reduce Eg to 1.86 eV. When using In+ to replace Ag+, it was predicted that the Eg of Cs2InBiCl6 (1.02 eV) and Cs2InSbCl6 (0.91 eV) would be very suitable for photovoltaics[12]. However, In+ is extremely unstable and can be easily oxidized into In3+[13].
When using Cu+/Cu2+ to replace part of Ag+ in Cs2AgBiBr6, there is no obvious effect on Eg[14]. Cu+ (ionic radius 77 pm, hereafter omit) and Cu2+ (73 pm) are much smaller than Ag+ (115 pm). They can cause lattice defects, and the absorption tail extends from 610 to 860 nm. This was due to the absorption of the defect intermediate state. Similarly, Fe3+ (65 pm) was used to replace part of Bi3+ (103 pm)[15]. Since the size of Fe3+ is quite different from Bi3+, it can easily cause lattice distortion and defects. The lattice constant was reduced from 11.27 to 11.25 ?, and Cs2AgBi0.886Fe0.114Br6 was obtained as a black crystal. Though Eg did not change obviously, the light absorption was greatly increased by defects. Recently, Gao et al.[16] demonstrated that Fe3+ could replace diamagnetic In3+ to yield Cs2AgIn1–xFexCl6 and form [FeCl6]3–·[AgCl6]5– domains, and the connection of larger [FeCl6]3–·[AgCl6]5– domains leads to segregated Fe3+-rich phases. The Eg of Cs2AgInCl6 was tuned from 2.8 to 1.6 eV via Fe3+-alloying.
The second approach to tune Eg of double perovskite is to adjust the physical structure. First-principles calculations indicated that changing the order of Ag+ and Bi3+ in space can significantly reduce Eg. When the arrangement of Ag+ and Bi3+ was completely disordered, the Eg could be reduced to 0.44 eV[17]. Gao et al.[18] reported that through thermally-induced defects, as the temperature increased, the color of Cs2AgBiBr6 single crystal and film can change from red to black, the Eg decreased, but the original color of the film recovered after cooling. The finite-temperature molecular dynamics simulations indicated the synergistic effect of the incongruous bond lengths (RAg–Br and RBi–Br) fluctuations and the related electron-phonon coupling, as well as the special spin-orbit coupling effect, were the cause for the thermochromism. Gao et al.[19] increased the disorder degree of [AgBr6] and [BiBr6] octahedral by adjusting the crystallization speed of Cs2AgBiBr6. As the temperature increases, the disorder degree gradually increases, the lattice shrinks, initially leading to the formation of isolated defect states in the forbidden band, and finally a series of defect states, and reduced Eg (60 °C, 1.98 eV; 150 °C, 1.72 eV). And this reduced Eg can keep stable at room temperature. Zou et al.[20] found that high pressure can also significantly reduce Eg of Cs2AgBiBr6 single crystal. Under 15 GPa pressure, the Eg was reduced from 2.2 to 1.7 eV. When the pressure was released, the Eg was relatively lower than that at atmospheric pressure.
In summary, for double perovskite Cs2AgBiBr6, the effect of A-site doping on Eg is not obvious, the stability of X-site doping is intractable, and only B-site doping can significantly tune Eg. Changing the order of Ag+ and Bi3+ in space is much effective for reducing Eg (Table 2). We need further study Eg-narrowing mechanism of double perovskites and find effective methods to realize it.
Precursor | Method | Product | Eg (eV) | Feature | Ref. |
Cs2AgBiBr6 | A | Cs2(Ag1–aBi1–b)TlxBr6 (x = a+b) | 1.40–1.95 | Toxicity of Tl | [9] |
Cs2AgBiBr6 | A | Cs2(Ag1–(2a+b)Sna(II))(Bi1–bSnb(IV))Br6 | 1.48 (i) and 1.71 (d) | Unstable Sn2+ | [10] |
Cs2AgBiBr6 | A | Cs2Ag(Bi1–xMx)Br6(M:In,Sb) | 1.86 (Sb0.375) –2.28 (In0.75) | Sb3+ decreases while In3+ increases Eg | [11] |
Cs2AgBiBr6 | A | Cs2(Ag:Cu+/Cu2+)BiBr6 | Tailing to 860 nm | Defect absorption | [14] |
Cs2AgBiBr6 | A | Cs2Ag(BiFe)Br6 | Tailing to 800 nm | Defect absorption | [15] |
Cs2AgInCl6 | A | Cs2AgIn1–xFexCl6 | 1.6–2.8 | For single crystal | [16] |
Cs2AgBiBr6 | B (temperature) | Cs2AgBiBr6 | Reversible | [18] | |
Cs2AgBiBr6 | B (temperature) | Cs2AgBiBr6 | 1.72–1.98 | For single crystal | [19] |
Cs2AgBiBr6 | B (pressure) | Cs2AgBiBr6 | 1.70 @15 GPa | Partially retainable | [20] |
A: Chemical composition. B: Physical structure. |
Table2.
Tuning Eg of double perovskites.
Table options
-->

Download as CSV
Precursor | Method | Product | Eg (eV) | Feature | Ref. |
Cs2AgBiBr6 | A | Cs2(Ag1–aBi1–b)TlxBr6 (x = a+b) | 1.40–1.95 | Toxicity of Tl | [9] |
Cs2AgBiBr6 | A | Cs2(Ag1–(2a+b)Sna(II))(Bi1–bSnb(IV))Br6 | 1.48 (i) and 1.71 (d) | Unstable Sn2+ | [10] |
Cs2AgBiBr6 | A | Cs2Ag(Bi1–xMx)Br6(M:In,Sb) | 1.86 (Sb0.375) –2.28 (In0.75) | Sb3+ decreases while In3+ increases Eg | [11] |
Cs2AgBiBr6 | A | Cs2(Ag:Cu+/Cu2+)BiBr6 | Tailing to 860 nm | Defect absorption | [14] |
Cs2AgBiBr6 | A | Cs2Ag(BiFe)Br6 | Tailing to 800 nm | Defect absorption | [15] |
Cs2AgInCl6 | A | Cs2AgIn1–xFexCl6 | 1.6–2.8 | For single crystal | [16] |
Cs2AgBiBr6 | B (temperature) | Cs2AgBiBr6 | Reversible | [18] | |
Cs2AgBiBr6 | B (temperature) | Cs2AgBiBr6 | 1.72–1.98 | For single crystal | [19] |
Cs2AgBiBr6 | B (pressure) | Cs2AgBiBr6 | 1.70 @15 GPa | Partially retainable | [20] |
A: Chemical composition. B: Physical structure. |
Acknowledgments
This work was supported by the National Natural Science Foundation of China (61775004, 61935016). L. Ding thanks the National Key Research and Development Program of China (2017YFA0206600) and the National Natural Science Foundation of China (51773045, 21772030, 51922032, 21961160720) for financial support.