As containing earth-abundant elements, kesterite (Cu2ZnSn(S,Se)4, CZTSSe) semiconductors have great potential to be low-cost and environmental-friendly inorganic absorbers. However, the record power conversion efficiency (PCE) for CZTSSe solar cells is only 12.6%[1, 2], much lower than that for Cu(In,Ga)Se2 (CIGS) solar cells (23.35%)[3]. The key issue for kesterite solar cells is the large open-circuit voltage deficit (Voc,def, the gap between Voc and Shockley-Queisser limit VocSQ) or small Voc gain (Voc/VocSQ). The Voc/VocSQ is higher than 85% for high-performance CIGS solar cells but only 61% for current world-record CZTSSe device[2]. Many factors may cause the Voc loss of kesterite: (1) the narrow phase stability makes it difficult to achieve highly uniform absorber composition, which can result in bandgap fluctuation and secondary phases; (2) the similar ionic size of Cu and Zn leads to high concentration of Cu–Zn antisite defects (Cu–Zn disorder), which may cause electrostatic potential fluctuation and band tailing; (3) the multi-element composition and the variable valence of Sn lead to complicated defect property, causing serious recombination in absorber bulk and interfaces[4-8]. Identify the most critical one and its origin is crucial for further improving device efficiency.
Cu–Zn disorder was once blamed to account for band tailing and limits kesterite Voc, which has now been disapproved by experiments and theoretical calculations[9, 10]. "Order–disorder" transition experiments by Rey et al. indicated that the order level of kesterite can be improved through long-time annealing at low temperature, which increases the bandgap (Fig. 1(a))[11]. Bourdais et al. reported that the Voc of kesterite was indeed improved upon long-time annealing (Figs. 1(b) and 1(c)), and indicated that Cu–Zn disorder is not directly responsible for the Voc deficit. They suggested that the defect clusters of [2CuZn+SnZn] might cause band tailing and limit the Voc[12]. Comparing the photocurrent and photoluminescence spectra for the CZTS films prepared under Sn-rich and Sn-poor conditions, Ma et al. confirmed the band tailing of kesterite indeed comes from the high concentration of [2CuZn+SnZn] defect clusters, not Cu–Zn disorder (Fig. 1(d))[13]. At the same time, their results also showed that the concentration of [2CuZn+SnZn] defect cluster as well as deep donor states SnZn and [CuZn+SnZn] formed under Sn-rich condition can be sufficiently suppressed under Sn-poor condition (Fig. 1(d)). Considering that the state-of-the-art kesterite absorber materials are made under slightly Sn-poor condition, to what extent the band tailing limits device Voc needs further investigation.

class="figure_img" id="Figure1"/>
Download
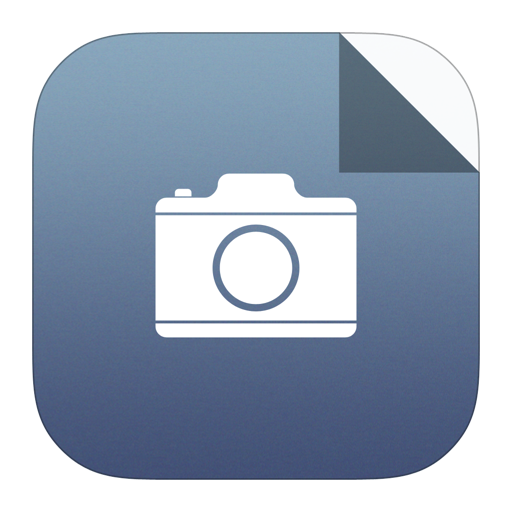
Larger image
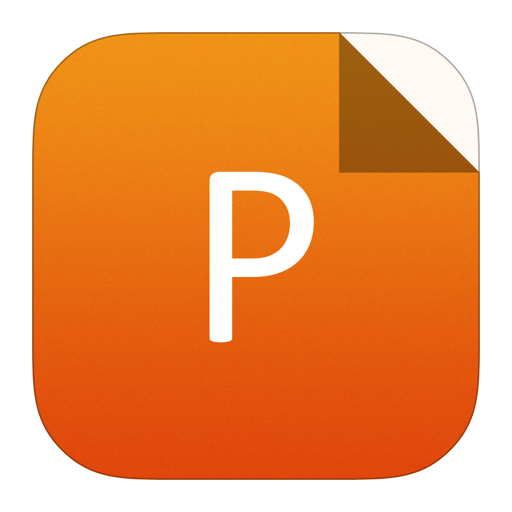
PowerPoint slide
Figure1.
(Color online) (a) “Order–disorder” transition of kesterite upon thermal annealing. Reproduced with permission[11], Copyright 2014, AIP Publishing LLC. (b) J–V curves for CZTSSe devices with PD (partially disordered) and PO (partially ordered) absorber. (c) Extraction of the bandgaps for CZTSSe absorbers in (b) from the absorption spectra. Reproduced with permission[12], Copyright 2016, Wiley-VCH. (d) Illustration of the band-tail and donor defect states for CZTS absorbers made under Sn-rich and Sn-poor conditions. Reproduced with permission[13], Copyright 2019, American Chemical Society.
Recently, Xin et al. found CZTSSe solar cells made from DMSO solution exhibited very different Voc when Sn precursor had different oxidation state (Sn2+ vs Sn4+)[14]. The device from Sn4+ solution gave much high Voc than that from Sn2+ solution, yielding a record low Voc deficit (0.297 V, Voc/VocSQ = 63.7%). Investigation on solution chemistry showed that different chemical reactions of Sn2+ and Sn4+ in precursor solution resulted in different composition in precursor films. The coordination of Sn2+ with thiourea (Tu) led to a highly crystalline precursor film containing various sulfide phases (Cu2–xS, ZnS, SnS, and CZTS), while the coordination of Sn4+ with DMSO resulted in a uniform precursor film with kesterite (CZTS) structure. The study on grain growth showed that the secondary sulfides in Sn2+ precursor film first converted to selenides (Cu2–xSe, ZnSe, SnSe2) and then took a multi-step fusion reaction to form the absorber film with secondary phase SnSe2 existing near film surface (Fig. 2(a)), giving a very defective surface, while Sn4+ film took a direct phase transformation from CZTS to CZTSSe without secondary phases (Fig. 2(b)), presenting a clean surface[15]. Characterizations showed that both absorber films presented similar bulk electronic property with comparable bandgap fluctuation (σg, Fig. 2(e)), electrostatic potential fluctuation (

class="figure_img" id="Figure2"/>
Download
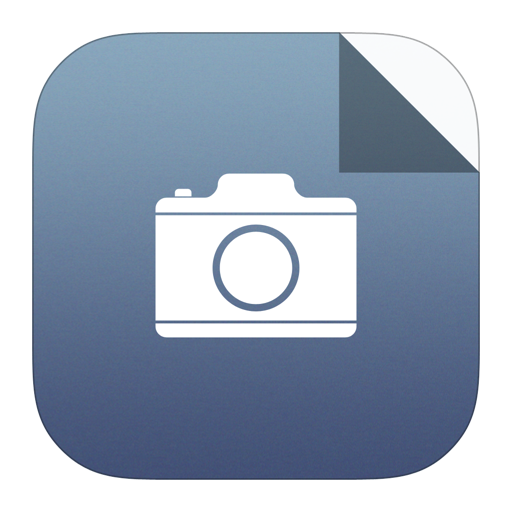
Larger image
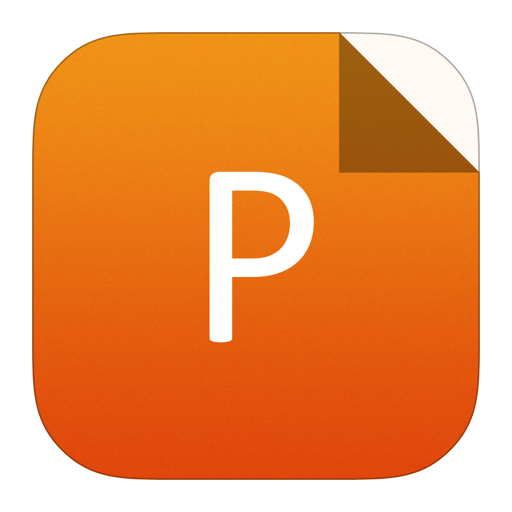
PowerPoint slide
Figure2.
(Color online) SEM images at different stage of selenization clearly show (a) grain growth of Sn2+ film with a multi-phase conversion and (b) grain growth of Sn4+ film with a uniform direct transformation. (c) J–V curves, (d) EQE spectra, (e) bandgap fluctuation (σg), (f) electrostatic potential fluctuation (γopt), and (g) Urbach energy (EU) for CZTSSe devices made from Sn2+ and Sn4+ solutions with and w/o thermal annealing. (h) Defect profiles measured by capacitance–voltage (C–V) and drive-level capacitance profiling (DLCP) for thermally-annealed Sn2+ and Sn4+ devices. Reproduced with permission[15], Copyright 2021, Royal Society of Chemistry.
The Voc deficit for kesterite solar cells was ascribed to the deep defects on/near the absorber surface due to SnSe2 involved in the grain growth. This work demonstrates that the grain growth accompanied with a direct phase transformation is crucial for obtaining high-quality kesterite films. This approach is expected to further boost the PCE for kesterite solar cells via well engineering CZTSSe/CdS interface.
Acknowledgements
This work was supported by the National Key Research and Development Program of China (2019YFE0118100) and the National Natural Science Foundation of China (U1902218, 22075150). L. Ding thanks the National Key Research and Development Program of China (2017YFA0206600) and the National Natural Science Foundation of China (51773045, 21772030, 51922032, 21961160720) for financial support.