1.
Introduction
The massive and rapid development of global wearable electronics is following the trend of miniaturization, portability, and functionalization, which promotes the rapid transformation of power supply and demand mode. On the one hand, the excessive consumption of fossil fuels with the consequent of resources shortage and environment pollution have brought great challenges to global energy supply, which makes it an unprecedented urgency to explore a new way of carbon neutrality with the possibilities of developing renewable, sustainable, and green energy[1, 2]. On the other hand, the conventional power generation from power plants with the fixed, concentrated, ordered and high-quality features has been centralized and then transmitted over long distances via electricity networks to local areas, which is inconsistent with the portable, distributed, disordered and low power energy supply requirements of the next-generation wearable electronics in the new era of internet of things (IoTs) and artificial intelligences (AIs)[3, 4]. Therefore, a new energy supply strategy is urgently needed for future wearable electronics.
To tackle the above issues, researchers are committed to assembling flexible, uninterruptible, and wearable self-charging power systems by integrating various energy-harvesting and energy storage devices through the circuit management system, which can make environmental energy simultaneously scavenged and stored for self-sustainable power supply[5], as illustrated in Fig. 1. In other words, wearable electronics with the ability of energy-autonomy is expected to be self-powered by conformable energy storage devices. For energy harvesting, the idea is to utilize whatever is available in the environment at which the device is deployed, at or near where it will be used[6]. With considering the working status of each sensor, it is feasible to use the energy harvested from the environment, such as solar energy, body motion energy, vibration energy, thermal energy, and chemical energy. Over the past decades, various energy harvesting strategies have emerged, such as the photovoltaic effect for harvesting solar energy[7-9], the thermoelectric and pyroelectric effects for harvesting thermal energy[10, 11], the piezoelectric and triboelectric effects for harvesting mechanical energy[12, 13], the biocatalytic effect for harvesting human swear energy[14, 15], and the water molecules ionized effect for harvesting atmospheric moisture energy[16, 17], which have been receiving great attention in the field of research into renewable and sustainable self-powered systems[18]. In particular, triboelectric nanogenerator (TENG) is a new energy harvester that can convert small scale mechanical energies including human respiration[19], ultrasound energy[20], mechanical stress[21], heart beat energy[22], and so on, into electrical energy by a combination effect of triboelectrification and electrostatic induction[23, 24]. The periodic contact-separation and/or sliding movement between two tribo-materials with different abilities of gaining or losing electrical charges contributes to electricity generation[25]. With the advantages of low cost, easy process, environmentally friendly, and diverse choice of materials, TENG has broad range of applications ranging from wearable power sources[26], physiological monitoring[27], human-machine interfaces[28], to artificial intelligences[2]. However, because of the naturally uncontrollable and unstable features of environmental mechanical energy, the converted electrical energy from energy harvesters is unstable and difficult to use as a direct power source for electronic devices. The most conventional technology is using electrochemical energy storage elements such as either lithium-ion batteries (LIBs) or supercapacitors (SCs) or microsupercapacitors (MSCs), which is able to stabilize and control the power output for direct applications. On the contrary, rechargeable LIBs or SCs also require continuous and stable power supply to achieve sustainable operation. Therefore, sustainable self-charging power systems that can synchronously harvest and store energy are able to provide a maintenance-free secondary power supply for futuristic next-generation wearable applications.

class="figure_img" id="Figure1"/>
Download
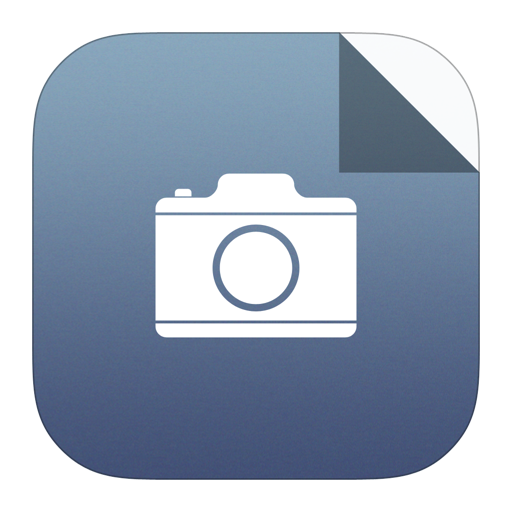
Larger image
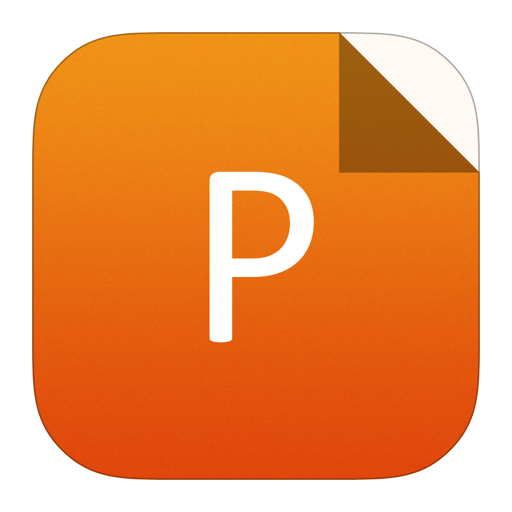
PowerPoint slide
Figure1.
(Color online) Schematic illustration of self-charging power textiles, mainly including fiber/fabric-based energy harvesting units, fiber/fabric-based energy storage unit, and power management circuits.
At present, a variety of combinations of energy harvesting units and energy storage units have been reported to design self-charging power systems, including solar cell-driven photo-rechargeable power cell (Fig. 2(a))[9, 29-31], thermoelectric generator coupled MSCs[32], triboelectric-driven self-charging SC power system (Fig. 2(b))[33], piezoelectric-driven electrochemical self-charging SC power cell (Fig. 2(c))[34], hybrid biofuel cells (BFCs) and SCs power system (Fig. 2(d))[35], and chemically self-charging aqueous ZIBs[36]. Compared with other energy harvesting methods that depend on external conditions, such as sunlight, temperature, and auxiliary catalyst, TENGs can run all day without extra burden or discomfort, which have more possibilities for application in wearable power supply and multifunctional self-powered sensing. However, conventional power systems with bulk and rigid configuration are not practical for use in wearable applications that require flexibility and comfortability[37]. Textiles with the form of fibers and fabrics can withstand versatile complex mechanical deformations, which exhibit outstanding ability of structural retention and fatigue resistance during wearing and washing. Textile TENGs with diverse structural designability and excellent performance represent an attractive interactive medium for human-oriented electronic integration[38]. Textile-based self-charging power systems with the form of fibers[39] and fabrics[27, 40] are an important part of smart textiles in the future. Although the review or perspective articles on self-charging power systems have been widely reported[41-45], there are few summaries on TENG-based self-charging power textile, which greatly limits its further research and application development.

class="figure_img" id="Figure2"/>
Download
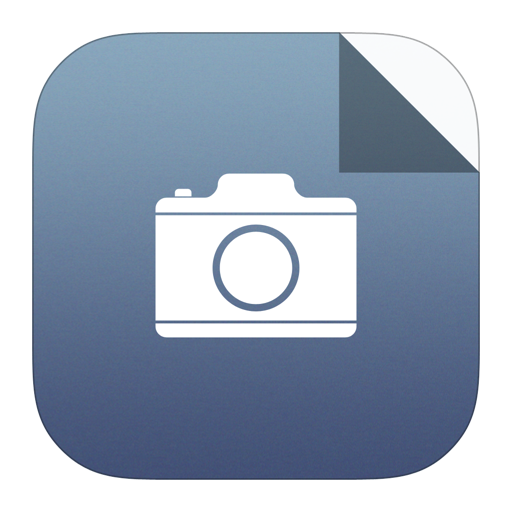
Larger image
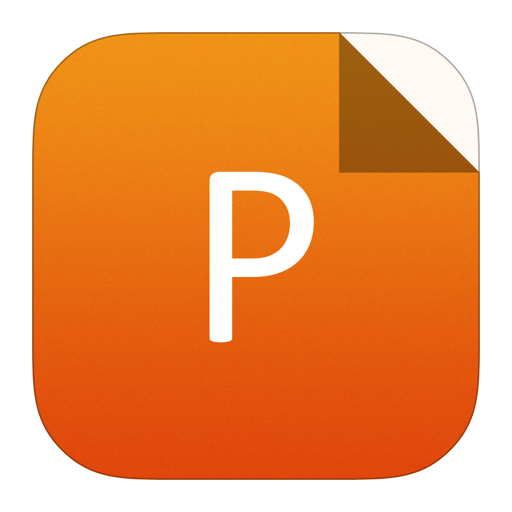
PowerPoint slide
Figure2.
(Color online) All-in-one integrated self-charging power systems based on different hybridizing modes, including (a) photorechargeable energy storage system. Reproduced with the permission from Ref. [9]. Copyright 2019, Elsevier. (b) Triboelectric coupled with microsupercapacitor self-charging system. Reproduced with the permission from Ref. [33]. Copyright 2020, Elsevier. (c) Piezoelectric-driven electrochemical self-charging SC power cell. Reproduced with the permission from Ref. [34]. Copyright 2020, Springer Nature Group. (d) Biofuel cell and SC hybrid self-charging system. Reproduced with the permission from Ref. [35]. Copyright 2018, The Royal Society of Chemistry.
Here, the latest development of self-charging power textiles based on the combination of fiber/fabric energy harvesting TENGs with fiber/fabric energy storing units are comprehensively summarized from the aspect of textile structure design, which includes fabricating in single fibers, interweaving with TENG fabrics, interweaving with fiber TENGs and fiber SCs, developing from fabric substrates, preparing with fabric-related membranous structures, and designing with multi-module combination. The potential challenges and brighter prospects towards the further research and practical application of self-charging power textiles are briefly discussed at the end of this review. We firmly believe that self-charging power textiles will become an important part of the future wearable energy supply.
2.
Self-charging power textiles
2.1
All-in-one self-charging power fibers
1D fiber-shaped electronic devices with light-weight, flexibility, and small diameters from tens to hundreds of micrometers have attracted broad interests in wearable electronic fields[46]. Recently, self-charging power systems have also been designed with 1D fiber construction. Early studies often integrate the two independent systems of TENGs and SCs by an external wire connection. For instance, a novel flexible self-charging power system prototype consisting of fiber SCs and fiber TENGs is reported for the first time by integrating three SCs with a TENG via a rectifier for harvesting mechanical energy from human motion[47]. However, fiber TENGs and fiber SCs are relatively independent and fixed on membranous substrates, which greatly limit the structural integrity and wearable application ability. With the help of novel all-in-one fiber structural design, such as coaxial, twisting, and braiding, the self-charging power systems can be integrated into one fiber system, which endows them with excellent wearability and mechanical stability to adapt to a variety of deformations, such as bending, stretching, and distortion. Several works have already designed self-charging power fibers with a coaxial configuration. For example, a flexible coaxial fiber by fabricating a 1D TENG outside and a SC inside, which can not only harvest mechanical energy but also store energy[48]. The all-in-one self-charging fiber utilizes carbon fiber bundles as the electrode material for the TENG and the active material for the SC, silicone rubber elastomer as the separator, triboelectric and encapsulation material (Fig. 3(a)). The proposed coaxial fiber with high flexibility and working stability can be further knitted as cloth for sustainably powering wearable electronics. Similarly, a multifunctional coaxial energy-autonomy fiber composed of a fiber-shaped TENG outside and SC inside is developed towards synchronous energy harvesting, energy storage, and energy utilization[49]. As shown in Fig. 3(b), through a bridge rectifier as an AC-to-DC converter, the coaxial self-charging power fiber can directly light up LEDs and drive a temperature-humidity meter. The fiber TENGs and fiber SCs are usually separated by a certain gap between them, which can make them relatively independent without interaction. However, the inserted gap usually increases the diameter of functional fiber devices, which also leads to an undesirable decrease in mechanical stability due to the weak interface. There are also studies devoted to the close integration of fiber TENGs and fiber SCs. A spontaneous hybrid smart fiber with asymmetry coaxial structure that not only scavenges surrounding mechanical energy, but also synchronously stores the harvested energy by charging a fiber SC without any external electric circuit is introduced[50]. As shown in Fig. 3(c), two carbon fiber bundle electrodes with the phosphoric acid (H3PO4)-polyvinyl alcohol (PVA) electrolyte are twisted together, which is further encapsulated by polymer polyvinylidenefluoride-trifluoroethylene (PVDF-TrFE) solution as the triboelectric material using the brush printing method. The spontaneous self-charging mechanism of the hybrid fiber can be briefly described as the coupling effect between triboelectric and electrochemical effects.

class="figure_img" id="Figure3"/>
Download
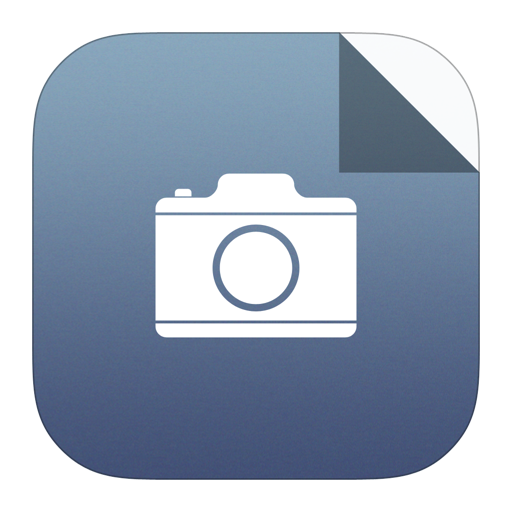
Larger image
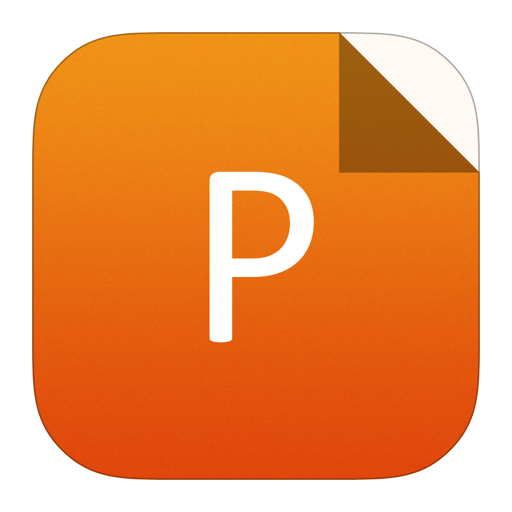
PowerPoint slide
Figure3.
(Color online) All-in-one self-charging power fibers. (a) A flexible coaxial self-charging fiber with a fiber-shaped TENG outside and a fiber-shaped SC inside. Adapted with permission from Ref. [48]. Copyright 2018, American Chemical Society. (b) Multifunctional coaxial energy-autonomy fiber composed of an all fiber-shaped TENG, SC, and pressure sensor. Reproduced with permission from Ref. [49]. Copyright 2021, American Chemical Society. (c) A hybrid smart self-charging fiber with asymmetry coaxial structure by a spontaneous energy generation and storage. Reproduced with permission from Ref. [50]. Copyright 2020, Wiley.
2.2
Self-charging power fabrics interwoven with TENG fabrics
Considering the limited power output of single fiber TENGs, some research works have been carried out to improve the power output density of the energy harvesting units by preparing fabric-based TENGs through a variety of fabric interweaving techniques, such as weaving, knitting, braiding, and sewing. In such cases, self-charging power textiles with interwoven fabric TENGs and fiber LIBs or SCs are developed. For example, a self-charging power unit is realized by integration of a textile TENG cloth and a flexible LIB belt[51]. As shown in Fig. 4(a), a heartbeat meter strap worn at the chest is powered by a LIB belt, which is further charged or compensated by a TENG-cloth worn under the arm. The TENG cloth is woven by two kinds of fabric strips, one of which is the polyester fabric coated with nickel, the other of which is the nickel-coated polyester fabric further covered by parylene layer. Considering that the LIB belts are difficult to be integrated into fabric systems, fiber-shaped SCs are designed to develop self-charging power textiles. Fig. 4(b) shows a proposed self-charging power textile that in one individual cloth integrates three functional units, i.e., fabric TENG for energy harvesting, fabric SC for energy storage, and wearable electronics or sensors for application[52]. With the self-powered charging fabric, the potential deficiencies of unstable output of TENGs and the short operation time of SCs can be simultaneously overcome. The fabric TENG is woven using Ni-coated polyester straps and parylene-Ni-coated polyester straps as the warp and weft yarns, respectively. Under the contact and separation mode motion at 5 Hz, the fabric TENG can achieve 40 V open-circuit voltage and 5 μA short-circuit current. The symmetric fiber SC is designed utilizing Ni-coated polyester fibers as the electrodes, rGO as the active materials, and PVA/ H3PO4 gel as the solid electrolyte as well as the separator. With the help of the bridge rectifiers, three-series connected fiber SCs can be continuously charged by the fabric TENG. However, although the fabric TENGs designed in the form of cloth strips can increase the effective contact area and enhance the electrical output, they cannot meet with further application and industrial scale-up manufacture. Therefore, it is more desirable to design and prepare fabric TENGs through traditional and mature textile forming technologies, such as weaving, knitting, braiding, and etc. For example, a fully stretchable fabric TENG with knitted fabric structures and scale preparation ability is designed for harvesting human biomechanical energy in cloth and garments[53]. The large-scale fabrication technology of fabric TENGs is further extended to self-charging power textiles. By utilizing traditional flying-shuttle woven craft, a one-piece self-charging power textile consisting of a fabric TENG and a woven SC for simultaneously harvesting and storing human motion energy is fabricated[54]. As shown in Fig. 4(c), the fabric TENG with the contact-separation mode is composed of a PTFE fabric and a carbon fabric, the latter of which is connected through the external load. The fabric SC is fabricated with carbon fibers as the electrode, RuO2 coated on carbon fibers as the active material, cotton fibers as the separator, and PVA/ H3PO4 gel as the electrolyte. Under the frequency of 1.5 Hz, the self-charging power fabric exhibits excellent charging performance with low charge leakage under a continuous charging process, demonstrating its potential ability to drive low-power wearable electronics.

class="figure_img" id="Figure4"/>
Download
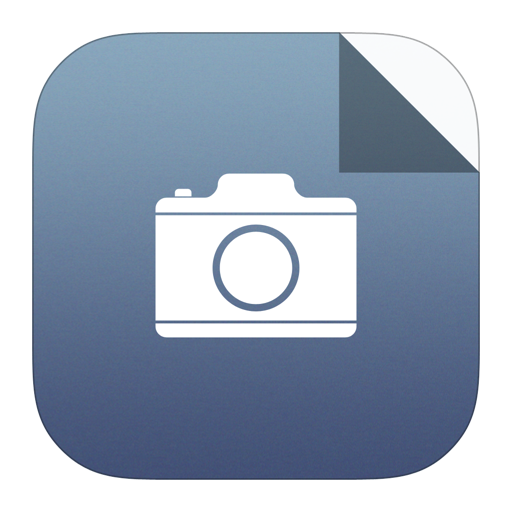
Larger image
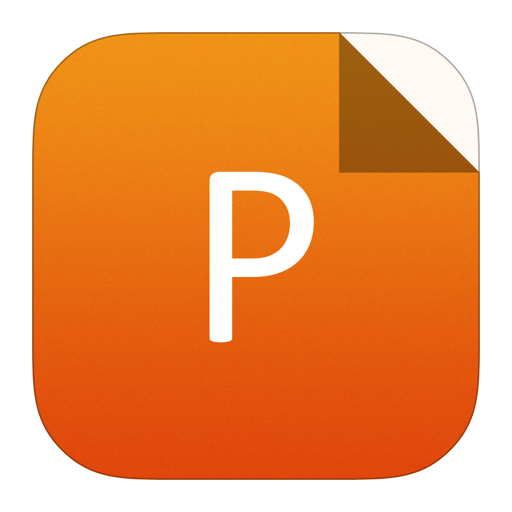
PowerPoint slide
Figure4.
(Color online) Self-charging power textiles developed with interwoven TENG fabrics. (a) A novel integrated self-charging power unit consisting of a flexible energy harvesting TENG cloth and a flexible LIB belt. Reproduced with permission from Ref. [51]. Copyright 2015, Wiley. (b) A textile self-charging power system designed by charging a fiber SC with a TENG cloth. Reproduced with permission from Ref. [52]. Copyright 2016, Wiley. (c) A one-piece self-charging power textile integrating a fabric TENG and woven SC for simultaneously harvesting and storing body motion energy to sustainably drive wearable electronics. Reproduced with permission from Ref. [54]. Copyright 2020, Elsevier. (d) Self-charging power fabric integrated with direct current TENG and fiber SCs. Reproduced with permission from Ref. [56]. Copyright 2020, American Chemical Society.
The contradiction between the alternating current (AC) electrical output form of TENGs and the direct current (DC) power demand severely limits their further research development and potential application[55]. Fabric-based DC TENGs with high power output are gradually developed based on triboelectrification and air breakdown effects[56, 57]. A self-charging power textile with DC fabric TENG and symmetrical fiber SCs is designed[56]. As presented in Fig. 4(d), by taking advantage of the electrostatic breakdown phenomenon, the DC TENG with PA nonconductive fibers as the warp yarns, and PA nonconductive fiber separated with PA conductive fibers as the weft yarns is fabricated with the plain woven structure. With the size of 6.8 × 7 cm2, the fabric DC TENG can achieve VOC of 4500 V, ISC of 40 μA, and QSC of 4.47 μC. The solid-state symmetrical fiber SC is fabricated with carbon fibers as the electrode, PEDOT:PSS as the active material, and PVA/H3PO4 gel as the electrolyte. With a simple and efficient circuit design, the self-charging power textile can power the hydrothermograph and calculator.
2.3
Self-charging power fabrics interwoven with fiber TENGs and fiber SCs
It seems to be a more efficient way to integrate fiber TENGs and fiber SCs directly into a fabric system, which greatly improves the integration of self-charging power systems. In previous studies, we have reported a highly stretchable and washable all-yarn-based self-charging knitting power textile that enables both biomechanical energy harvesting and simultaneously energy storing by hybridizing TENG and SC into one fabric[58]. As shown in Fig. 5(a), the highly stretchable and shape-adaptive self-charging power textile is obtained by integrating energy harvesting fiber TENGs with a flexible energy storing fiber SCs via weft knitting technique. The fiber TENG is fabricated by coating silicone rubber on the surface of the conductive fiber. The all-solid-state symmetric fiber SC is fabricated by dip-coating carbon nanofiber and PEDOT:PSS successively on a carbon fiber bundle. With the addition of a full-wave rectifier, the self-charging power textile can continuously drive a temperature-humidity meter or a calculator by hand tapping it. Similarly, a flexible and easy large-scale production of single-electrode mode TENG and SC yarn-based self-charging power fabric for simultaneously converting and storing biomechanical energy is also presented[59], indicating that the proposed design is a promising sustainable power source for wearable electronic devices.

class="figure_img" id="Figure5"/>
Download
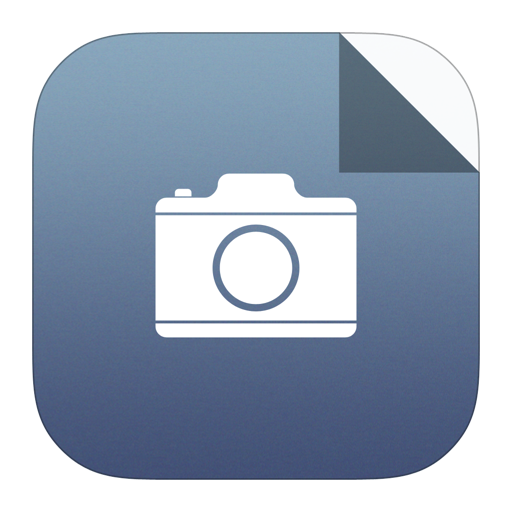
Larger image
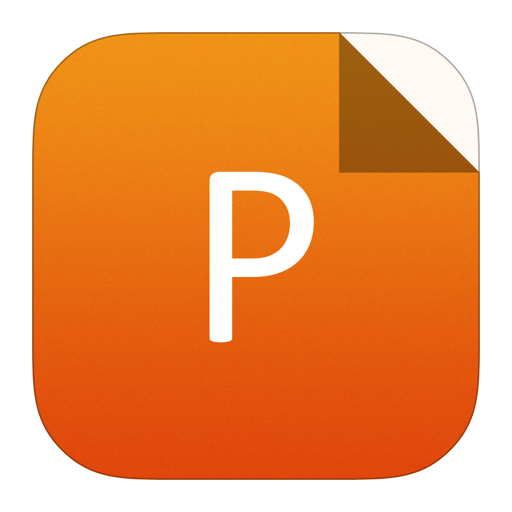
PowerPoint slide
Figure5.
(Color online) Self-charging power textiles fabricated with fiber-based TENGs and fiber-based SCs. (a) A highly stretchable and washable all-yarn-based self-charging knitting power textile composed of fiber TENG and fiber SC. Reproduced with permission from Ref. [58]. Copyright 2017, American Chemical Society. (b) Self-charging power textile interwoven by all-yarn-based energy harvesting TENG and energy storing yarn-type asymmetric SC. Reproduced with permission from Ref. [60]. Copyright 2019, Wiley. (c) All-in-one self-charging power textile developed by integrating fiber TENG with all-solid-state fiber-based asymmetric SC. Reproduced with permission from Ref. [63]. Copyright 2021, Elsevier.
In addition to the knitting technique, weaving is also an effective method to integrate fiber TENGs and fiber SCs into the self-charging power fabric systems. For example, a self-charging power textile enabled by yarn-based TENGs as the energy-harvesting devices and yarn asymmetric SCs as the energy storing unit is reported by Pu et al.[60]. As exhibited in Fig. 5(b), the energy-harvesting fibers are fabricated by encapsulating PDMS on the surface of the Cu-coated polyester fiber, and the energy-storing fibers consist of a negative fiber electrode with hydrothermally self-assembled rGO/CNT coating and a positive electrode of electroplated Ni-Co bimetallic oxyhydroxide coating. The serially connected fiber SCs can be charged by the woven TENG to 2.4 V in 104 min at about 3 Hz, which can power an electronic watch. However, due to the large impedance mismatch between TENGs and SCs, the charging efficiency is estimated to be only 2.6%. With proper power management, the energy utilization efficiency and charging rate can be further improved[61, 62]. Similar research works can also be found in other literatures. For instance, an all yarn-type self-charging power system coupling with TENGs and SCs is woven into cloth to continuously harvest and store bio-motion energy[63]. As demonstrated in Fig. 5(c), the fiber TENG is fabricated by wrapping PTFE fiber on the carbon fibers@PDMS/MnO2NW elastic fibers. The all-solid-state asymmetric fiber SC is designed with carbon fibers @ MnO2 as the positive electrode, carbon fibers @ activated carbon as the negative electrode, and Na2SO4/PVA simultaneously playing as the solid electrolyte and separator. The fiber SC can be charged by the fiber TENG under different motions, which can power a red LED.
2.4
Self-charging power fabrics developed from fabric substrates
In order to achieve more efficient fabrication of self-charging power fabrics, common fabrics are often used as the substrates, on which a variety of conductive and active materials are applied. The simplest way of developing self-charging power fabrics with fabric substrates is to directly stack the fabric TENG with single-electrode mode and fabric SCs together. Moreover, the structure design can be further simplified by sharing electrodes. For example, Zhang et al. presented an all-fabric-based self-charging power cloth by integrating a wearable single-electrode TENG and a flexible SC with a general carbon nanotube/cotton fabric electrode[64]. However, due to the stacking structure, the power generation of TENG induced from the mechanical loading will affect or even weaken the performance of the SC, which will reduce the stability of the self-charging device. Therefore, the design and preparation of self-charging power fabrics based on common fabric substrates require better structural design.
The earlier research work of self-charging power fabrics on fabric substrates is reported in 2014[65], which developed a wearable fabric-based integrated power-supply system that generates human motion energy using TENG and stores the generated energy in an integrated SC on a conductive carbon fabric, as shown in Fig. 6(a). The fabric TENG consists of two kinds of fabrics, one of which is fabricated by alternatively coating polyurethane and polyimide on a carbon fabric, the other of which is created by patterning PDMS and Al on a carbon fabric. The symmetric fabric SC is developed with sandwiched structure composed of CF/CNT/RuO2 electrode, PVA/H3PO4 gel electrolyte, and CF/CNT/RuO2 electrode. With the help of the rectifier circuit, the wearable energy supply system can be utilized either as self-powered activity monitors or as a power supply for external wearable sensors. As exhibited in Fig. 6(a), by stitching the fabric-based TENGs and SCs into commercial clothing, the self-charging power fabric can monitor and record the rectified output current and the charge accumulation from a subject simulating normal jogging procedures of stretching, walking, running, sprinting, and a cool-down walking. Based on the similar method, Pu et al. developed a stretchable coplanar self-charging power textile with TENGs and microsupercapacitors both fabricated through a resist-dyeing-analogous method[66]. As shown in Fig. 6(b), the stretchable coplanar self-charging power textile is demonstrated for powering small electronics intermittently without extra recharging. We can also use a variety of textile structures to achieve the design and fabrication of self-charging power fabrics. For example, Zhi et al. proposed an integrated flexible and wearable TENG and rechargeable ZIB system based on a flexible 3D spacer fabric as a promising wearable power unit for powering personal electronic devices[67]. As shown in Fig. 6(c), the electrical output from the fabric TENG can be effectively stored in the fabric SC via the rectifying circuit, which can then power electronic watches. Based on the above analysis, it can be found that the versatile fabric structures provide an effective and scalable design carrier and implementation platform for self-charging power textiles.

class="figure_img" id="Figure6"/>
Download
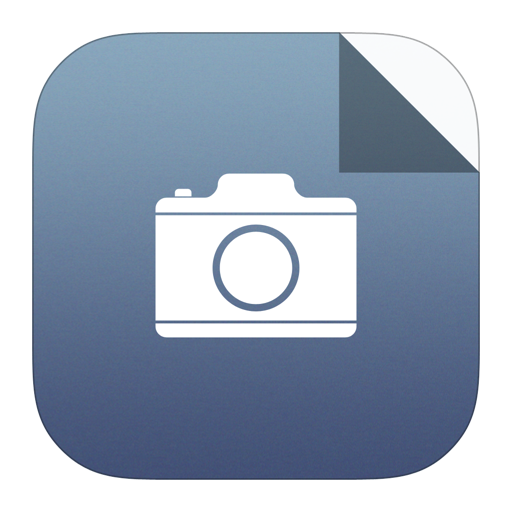
Larger image
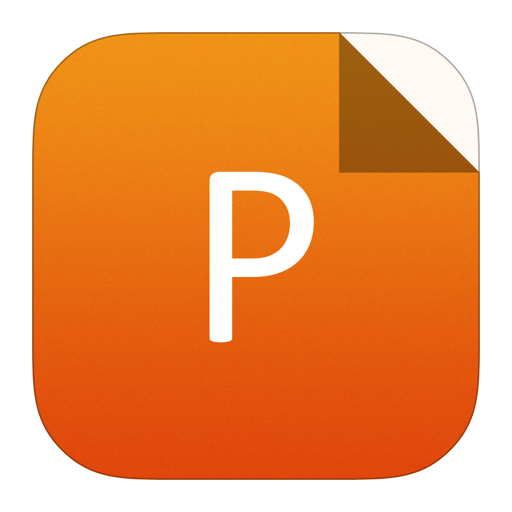
PowerPoint slide
Figure6.
(Color online) Self-charging power textiles developed from fabric substrates. (a) Wearable fabric-based integrated self-charging power supply system developed by storing triboelectric energy harvesting energy in an integrated SC. Reproduced with permission from Ref. [65]. Copyright 2014, Wiley. (b) Stretchable coplanar self-charging power textile with resist-dyeing TENG and microsupercapacitors. Reproduced with permission from Ref. [66]. Copyright 2020, American Chemical Society. (c) Integrating a TENG with a zinc-ion battery with a 3D spacer fabric structure. Reproduced with permission from Ref. [67]. Copyright 2018, Wiley.
2.5
Self-charging power textiles with fabric-related membranous configurations
Paper is often utilized as the substrate for building functionalized electronics, especially for self-charging power units[68, 69], due to its light weight, low cost, environmental friendliness, and ease of fabrication[70]. Inspired by paper-based electronic devices, textile related membrane structures are gradually used to design wearable and flexible electronics. Moreover, textile related membrane structures can also expand the design space and application scope of functional wearable electronics, including self-charging power fabrics, which have attracted great attentions. Nanofiber-based membranes are firstly used to design self-charging power systems. For example, an ultralight and flexible self-charging power system via all electrospun paper based TENGs as energy harvesters and all electrospun paper based SCs as a storage device is proposed[71]. As illustrated in Fig. 7(a), the nanofiber-based TENG made into arch shape is derived from one nonconductive polyacrylonitrile (PAN) nanofiber membrane as a triboelectric layer and conductive carbon nanofiber paper as electrodes. The nanofiber-based TENG is fabricated with conductive carbon nanofiber paper as capacitive materials and a nonconductive PAN nanofiber membrane as the separator. Similarly, a portable, flexible, and low-cost self-charging power system consisting of a paper-based TENG with high output density as the energy harvester and a paper-based SC with a great areal capacitance as the energy storage device is developed to meet the energy demand for flexible and miniaturized green electronics[72]. As shown in Fig. 7(b), the paper-based TENG is fabricated with the cellulose paper/ polypyrrole (PPy) composite as both electrode and the positive friction layer, and a nitrocellulose membrane as the negative friction layer. The paper SC is made of cellulose paper/PPy composite active material and H3PO4/PVA gel electrolyte. The all paper-based self-charging power system can drive a segmented LED display and a temperature/humidity indicator. In addition to nanofiber membranes, self-charging power units can be directly fabricated on common cloth. As shown in Fig. 7(c), a woven carbon fabric-based multifunctional TENG integrate with P-doped Cu–Mn selenide nanowire-based SC is developed for meeting future energy demands of self-charging automobiles, electronics, and various outdoor applications[73]. In addition to the stacking structure, TENGs and SCs can also be connected in the horizon direction. For instance, a highly compact self-charging power unit by integrating TENG with MXene-based MSCs in a wearable and flexible harvester-storage module[74]. Fig. 7(d) shows that the MSCs are prepared with MXene as the active and conductive material, and PVA/H3PO4 gel as the electrolyte by employing a spray-coating method followed by a direct laser cutting process. A single-electrode mode TENG based on carbon fiber embedded silicone is designed for integration with the silicone-encapsulated MXene-based MSC, which can successfully power up a thermo-hygrometer. It can be found that textile-related membranous structure is also a broad carrier and combination form to design all-in-one self-charging power textiles, which can realize integrated package.

class="figure_img" id="Figure7"/>
Download
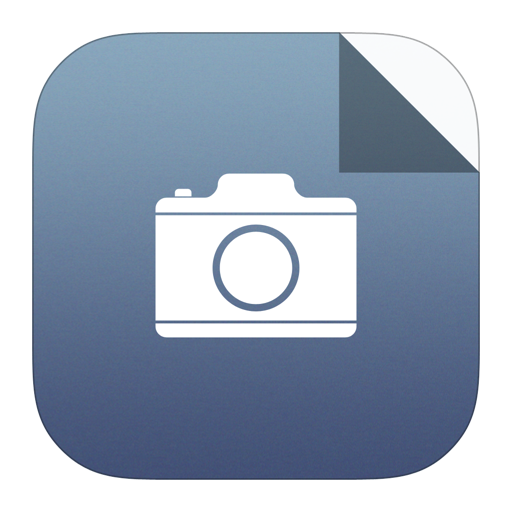
Larger image
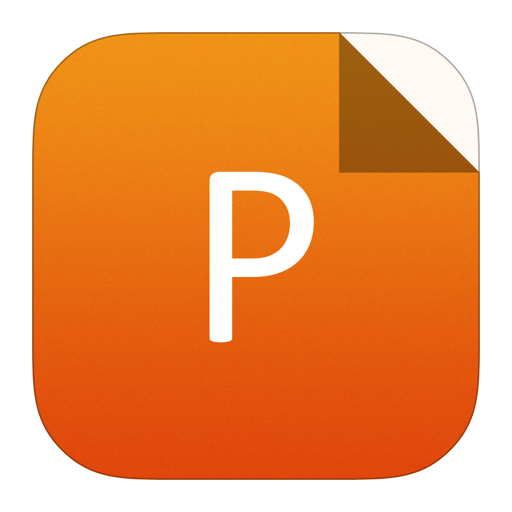
PowerPoint slide
Figure7.
(Color online) Fabric-based self-charging power systems with membranous constructions. (a) An ultralight and flexible self-charging power system via all electrospun paper based on TENGs as energy harvester and all electrospun paper based SCs as storage device. Reproduced with permission from Ref. [71]. Copyright 2017, Elsevier. (b) Paper-based self-charging power system consisting of a paper-based TENG and a paper-based SC. Reproduced with permission from Ref. [72]. Copyright 2019, American Chemical Society. (c) An integrated energy harvesting and storage system with TENG-integrated SC structure. Reproduced with permission from Ref. [73]. Copyright 2020, Elsevier. (d) A self-charging power unit by integrating MXene-based MSCs with TENG. Reproduced with permission from Ref. [74]. Copyright 2018, Elsevier.
3.
Multi-module combined self-charging power textiles
In many cases, the output power from individual energy harvester cannot completely fulfill the power requirements of the wireless sensor networks, partly because the energy source may not always be stable or continuously available in reality[75]. However, a variety of energy sources coexist in most situations. Single-source energy harvesters cannot harvest all of the energy sources and will waste part of the harvestable energy, thus hindering the maximization of their energy harvesting capability. Therefore, hybrid energy harvester that is able to harvest multiple energy sources is developed. The number of harvestable energy sources for the hybrid energy harvesters is increased and the output power is significantly improved when the harvesters suffer instability of a particular energy source, compared to the case for previous single-source energy harvesters[76]. Hybrid energy harvesters based on TENGs provide a promising method to effectively use the environmental conditions for energy harvesting by combining two or more working mechanisms, generating high currents by harvesting all types of available energy to meet the needs of distributed energy units[77].
Based on the hybrid energy harvesting strategies, multi-module combined self-charging power systems with complementary and synergistic energy harvesters and commensurate energy storage modules for maximized efficiency and performance are also developed. Photovoltaic solar cells can be hybridized with TENGs, which can collect solar energy and mechanical energy at the same time. For example, a textile-based self-charging power system is realized by integrating fabric TENGs and fiber-shaped dye-sensitized solar cells (DSSCs) to scavenge the energy of human motion and solar energy, which are further stored in a lithium-ion battery for sustainable power supply application[78]. The self-charging systems coupling with photovoltaic and mechanical energy harvesting can also be designed in all fiber forms. An all-fiber-based hybridized self-charging power textile is proposed with the aim of simultaneously collecting outdoor sunshine and random body motion energies and then storing them in an energy storage unit[79]. As illustrated in Fig. 8(a), a single fiber TENG is formed by connecting each fiber DSSCs and fiber SCs unit to one another. According to the charging curve, it can be found that the charging efficiencies of the self-charging power textile can be greatly improved. Similarly, a highly stretchable, wearable, and comfortable self-charging power system is fabricated to individually and simultaneously harvest solar energy and human motion energy, which are then stored in SCs to sustainably power electronics[80]. As exhibited in Fig. 8(b), two generators including three fiber DSSCs in series and a TENG and two SCs in series can be effectively integrated into a flexible and stretchable bracelet, which can power several portable electronic devices such as LED lights, electronic watches, and temperature sensors. In addition to the combination of solar cells and TENGs, other energy harvesting combination methods have also been gradually explored and studied. For instance, a self-sustainable wearable multi-modular e-textile bioenergy system is developed by harvesting biochemical and biomechanical energy using sweat-based BFCs and TENGs, and regulating the harvested energy via SCs for high-power output[81]. As shown in Fig. 8(c), an integrated microgrid system into one e-textile platform that unites BFCs and TENGs with distinct and complementary energy conversion mechanisms based on human activities, along with SC modules for regulating the powering of wearable applications with both low and high power demand. The complementary relationship between the two bioenergy harvesters thus compensates for the limitations of the BFCs due to delayed perspiration and of the TENGs due to the lack of motion. The SC modules regulate low-current, high voltage inputs from the TENG modules and high-current, low-voltage inputs from the BFC modules, with the optimal capacity to deliver sufficient power for designated applications while maintaining fast booting.

class="figure_img" id="Figure8"/>
Download
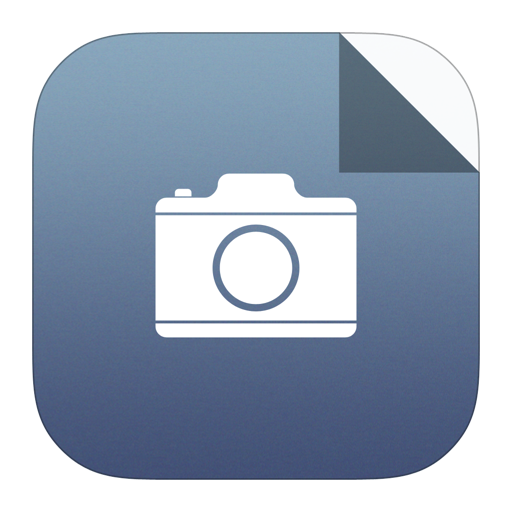
Larger image
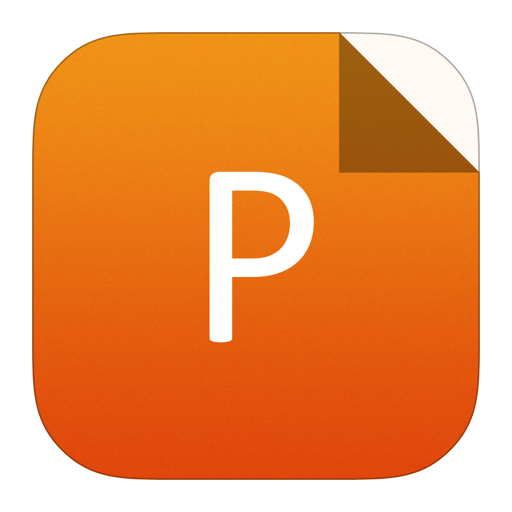
PowerPoint slide
Figure8.
(Color online) Self-charging power textiles with multi-modular energy harvesting methods. (a) Self-powered textiles for wearable electronics by hybridizing fiber-shaped TENGs, solar cells, and SCs. Reproduced from permission from Ref. [79]. Copyright 2016, AAAS. (b) Highly elastic self-charging power bracelet consisting of two energy harvesting devices, i.e., TENG and FDSSC, and an energy storage device. Reproduced from permission from Ref. [80]. Copyright 2019, Elsevier. (c) Self-sustainable wearable multi-modular E-textile by harvesting biochemical and biomechanical energy using sweat-based BFCs and TENGs and regulating the harvested energy via SCs. Adapted from permission from Ref. [81]. Copyright 2021, Springer Nature Group.
4.
Summary and discussions
In summary, the recent process of self-charging power textiles that integrate fiber/fabric energy harvesting TENGs with fiber/fabric-shaped energy storage LIBs/SCs are comprehensively summarized, which provides a promising energy-autonomy strategy to the next-generation wearable electronics. According to the textile structure design, the TENG-based self-charging power textiles can be divided into fabrication in single fibers, interweaving with TENG fabrics, interweaving with fiber TENGs and fiber SCs, development from fabric substrates, preparation with fabric-related membranous structures, and design with multi-module combination, each of which has been introduced and discussed with representative examples. However, although significant improvements have been achieved in the research development of self-charging power textiles, some critical problems or huge challenges regarding their further study and potential application are needed to be addressed, such as:
(1) Working performance. The relatively low output power of fiber/fabric TENGs is one of the key bottlenecks for self-charging power textiles. For energy storing devices, the self-discharge behaviors of SCs and the high threshold voltage of LIBs also greatly reduce the overall energy conversion efficiency. In addition, the pulsed electrical output mode may affect ion diffusion and transport across the isolating membrane. Tremendous efforts need to be made to improve the output performance of fiber/fabric TENGs as well as their combining ability with energy storage devices. Moreover, the performance stability of self-charging power textiles in the long-term working cycles is also very important. Reasonable packaging techniques are necessary to prevent the internal electrode and triboelectric charge from being interfered with by the external environment. Some polymer materials with excellent hydrophobicity and strong electrification ability are often adopted as packaging materials.
(2) Capacities/impedances mismatch. The energy harvesting TENGs with the electrical output characteristics of high voltage and low current do not match with the stored form of electrical energy of energy storing units, which is low voltage and high current. Many efforts should be done in order to improve energy conversion efficiency.
(3) Power management systems/circuits. The power management circuits including hard modules are hard to integrate into cloth, which requires certain flexibility and stretchability. In addition, although various kinds of power management circuits, including full-wave rectification[82], half-wave rectification[83], inductance-capacitor (LC) buck conversion with mechanical/electronic switch[61, 84], LC oscillation[85], switched capacitor converter based on fractal design[86, 87], have been designed to match the impedance between them, the development of more effective management circuits is still significant and urgent.
(4) Self-charging mechanism. The working principles of energy harvesting TENGs and energy storing devices have been widely studied and reported, which has basically reached a consensus. However, the potential working mechanisms of TENG-based self-charging power textiles are still not fully understood, which makes it difficult to explore new or higher performance self-charging devices. In most cases, self-charging mainly goes through three processes. Firstly, the mechanical energy is harvested and simultaneously converted into electric energy through the coupling effect of contact electrification and electrostatic induction. Secondly, the triboelectric signals with the characteristics of pulse, high voltage, low current, and alternating current (AC) require energy management circuits to transform into the electrical signals with stable, low voltage, steady current, and direct current (DC) mode. Finally, the stable DC output is stored in SCs or LIBs to power external electronic devices. More detailed explanations of the self-charging mechanism need the help of more advanced equipment and technology.
(5) Performance evaluation criteria. At present, the performance metrics of electrochemical energy storage (EES) devices have been widely reported, which mainly include energy density and power density[88]. However, challenges still remain daunting for establishing more sophisticated standard criteria for the evaluation EES systems. In addition, as a new technology, the properties of TENGs are evaluated based on different opinions without a unified standard, which makes it difficult to compare the performance of TENG-based self-charging power systems. Although some potential standards for evaluating the overall performance of TENGs, such as Q–V curves[89], figure-of-merits (FOMs) including structural FOM and material FOM[90], maximized effective output energy density[91, 92], and etc., their coverage and effectiveness still need a lot of verification as well as a further improvement. Therefore, the evaluation criterion of the overall performance of self-charging power systems, including charging efficiency should be unified in the future.
(6) Integration into one cloth system. For terminal wearable use, the components of self-charging power textiles should be highly integrated into one clothing system, and should have certain shape adaptability to human daily motions. This is not only related to the textile-related design of each unit, but also the connection and packaging of the circuit between the constituent units.
(7) Wearability and comfortability. The wearability and comfortability of self-charging power textiles are also particularly important since their service occasion is always for wearable usage. In general, the addition of functional attributes will lose part of the comfort, due to excessive chemical treatments and the occurrence of skin sensitive materials. In addition, external loads are required to stimulate the electricity generation of fiber/fabric TENGs, which will also cause discomfort to the human body. Therefore, more attention should be paid to wearability and comfortability on the basis of satisfying good working performance.
(8) Cost-effectiveness analysis. Most of the reported self-charging power textiles are still proof-of-concept prototypes, which are fabricated with expensive raw materials and complicated preparation processes. It is the primary concern and the only way to realize the commercial application of self-charging power textiles by reducing the cost of raw materials, simplifying the preparation process, and improving manufacturing efficiency.
As a new research direction with great application prospects, self-charging power textiles provide a unique solution for future energy autonomy energy supply and distributed self-powered sensing. Although it is full of difficulties and challenges in the road of practical application, we believe that the TENG-based self-charging power textiles will be extensively applied in our daily life in the near future owing to the unremitting efforts by a large number of researchers around the world, especially for wearable electronic devices and self-powered systems.
Acknowledgments
The authors are grateful for the support received from National Natural Science Foundation of China (Grant No. 22109012), the Beijing Municipal Natural Science Foundation (Grant No. 2212052), and the Fundamental Research Funds for the Central Universities (Grant No. E1E46805).