Silicon photonics has attracted a great deal of interest for integrated photonics systems due to its large-scale electronics–photonics integration on a chip by leveraging the fabrication process of the complementary-metal-oxide semiconductor (CMOS) foundries[1-5]. Germanium-on-silicon (Ge-on-Si) waveguide photodetector (PD) is an indispensable building block of silicon photonics technology which requires high sensitivity, large bandwidth, and low dark current. However, there is a tradeoff between the high sensitivity and large bandwidth. The inductive gain peaking technique in silicon photonics processes which is firstly proposed by Hochberg and co-authors demonstrated that the bandwidth of Ge-on-Si PD can be improved significantly without sacrificing responsivity and undesired effects[6-9]. Although the 3-dB bandwidth has achieved 60 GHz, the demonstrated electrical eye diagram rate is only up to 45 Gbit/s. The limited eye diagram bit rate may be attributed to the large signal phase delay with different frequency. In this work, by employing inductive gain peaking technique with suitable inductance for conventional vertical Ge-on-Si PD, the 100 Gbit/s non-return-to-zero (NRZ) on-off-keying (OOK) and 64 Gbaud four-level pulse amplitude modulation (PAM-4) clear open eye diagrams are firstly realized with 3-dB bandwidth of 62 GHz.
It has been proved that by utilizing an inductor to peaking the opto-electrical (OE) response of a PD, the 3-dB bandwidth can be increased[6, 7]. Fig. 1(a) shows the cross-sectional view of designed peaking Ge-on-Si PD. It is fabricated on a commercial standard silicon-on-insulator (SOI) platform, with 220 nm Si and 8 μm buried oxide (BOX) layers. A 500 nm height Ge film is grown on the 220 nm Si-layer, with its top 100 nm ion implanted by N+ for ohmic contact. The bottom 220-nm-thick Si waveguide is implanted by P and P+ for ohmic contact. The width and length of Ge are about 4 and 25 μm, respectively. Fig. 1(b) shows the current–voltage (I–V) characteristics of peaking PD in dark illuminated state. The dark current are about 8 and 46 nA at –1 and –3 V bias voltages. To further simulate how the PD equivalent circuit respond to the added inductor, a precisely circuit model is introduced, as shown in the inset of Fig. 1(c). The CjPD, RPD, Cpad, Rload, Rpk, Cpk, Lpk represent capacitance of PD junction, resistance of PD junction, capacitance of RF pad, resistance of load, resistance of inductor, capacitance of inductor and inductance, respectively. Here, the typical internal responsivity of this vertical Ge-on-Si PD is evaluated to be 0.8 A/W at 1550 nm. Fig. 1(c) shows normalized RF response of vertical Ge-on-Si PD with small peaking, large peaking and without peaking. The designed vertical Ge-on-Si PD with the large inductance shows an obviously over-peaking near 34 GHz followed by a sharp drop and a 3-dB bandwidth of 57 GHz. On the other hand, the vertical Ge-on-Si PD with small inductance possesses smoothing peak curve, but has 3-dB bandwidth of large than 60 GHz. The over-peaking PD would result in seriously signal distortion, which is because of the non-uniform time delay for different frequencies in the transient response[6]. Here, the inductance is elaborately designed to be 300 pH to decease the phase shift. By using a commercial software, the full 3-dimensional electromagnetic simulation of the inductor was performed to design the physical parameters. The simulation model of our inductor has also considered the processes of commercial standard SOI platform. After fabricated by the silicon photonics Fab, the PD device will be characterized with small signal and large signal measurements. The calibration of the high-speed RF trail was implemented to reduce the contributions from GSG probes and coaxial cables. The 3-dB bandwidth test experiments were achieved by collecting the response of the S21 transmission parameter in the Lightwave Component Analyzer (LCA, Keysight N4373D) tool versus frequency. Fig. 1(d) shows the experimental and fitted result of normalized RF response of vertical Ge-on-Si PD. The inset in Fig. 1(d) is the optical micrograph of the designed vertical Ge-on-Si PD with small inductance. By fitting the experimental result of S21 transmission parameter under 0.45 mA photocurrent, the 3-dB bandwidth of 62 GHz is realized with –3 V bias voltage. In order to obtain the inductance of fabricated PD, the S11 parameters are measured from 100 MHz to 60 GHz using LCA under –3 V bias voltage. Figs. 1(e) and 1(f) shows experimental and fitted magnitude/phase part of the small signal S11 reflection parameters. The series resistance, total capacitance, and inductance of Ge-on-Si PD are extracted by fitting S11 scattering parameter data to the small signal RC-model. The fitting result exhibits good agreement with the measured scattering parameter data curve, which results in a junction capacitance CjPD of 35 fF, junction resistance RPD of 24 ?, pad capacitance Cpad of 12 fF, inductor resistance Rpk of 0, inductor capacitance Cpk of 3 fF and inductance Lpk of 276 pH, respectively. The load resistance Rload is 50 ?. As the aforementioned, the optimum inductance of the designed device is 300 pH. However, the fitting inductor is 276 pH, which is smaller than the optimum value.

class="figure_img" id="Figure1"/>
Download
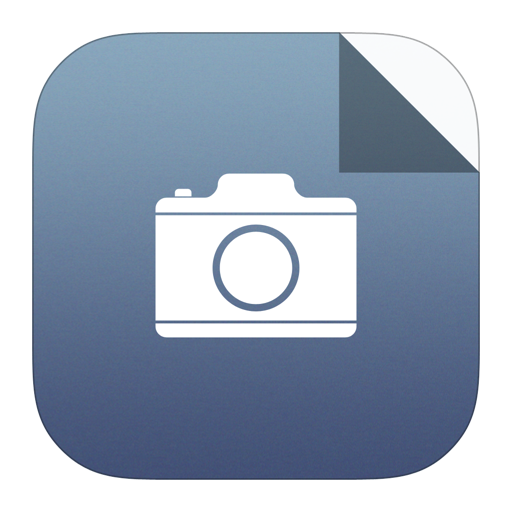
Larger image
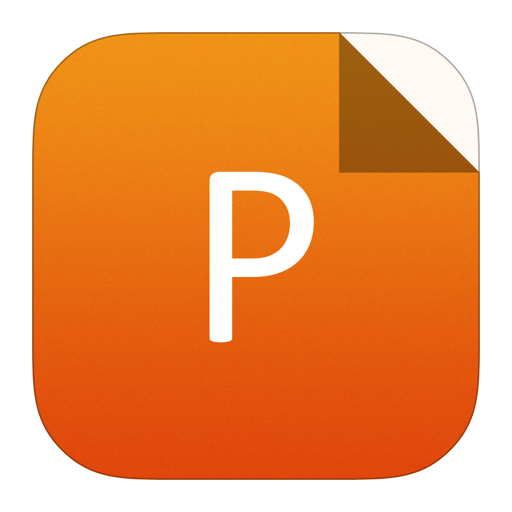
PowerPoint slide
Figure1.
(Color online) (a) Cross-sectional view of designed peaking Ge-on-Si PD. (b) I–V characteristics of designed peaking Ge-on-Si PD in dark illuminated state. (c) The simulated normalized S21 response of vertical Ge-on-Si PD with small peaking, large peaking and without peaking. (d) The experimental and fitted result of normalized RF response of vertical Ge-on-Si PD with small inductance, the inset is the optical micrograph of peaking PD. (e–f) The experimental and fitted magnitude/phase part of the small signal S11 reflection parameters from 100 MHz to 60 GHz at –3 V bias voltage, the blue and red line represent experimental and fitted result, respectively.
The feasibility of this Ge-on-Si PD with small inductance was further checked by measuring the eye-diagram large-signal acquisitions with different operation speed. The data were transmitted in NRZ and PAM-4 optical modulation formats. A 215 – 1 long optical NRZ pseudo random binary sequence (PRBS) data pattern at 70, 80, 90, 100 Gbit/s NRZ, and 40, 50, 60, 64 Gbaud PAM-4 generated by a commercial LiNbO3 modulator at 1550 nm was amplified by using a C-band erbium-doped fiber amplifier (EDFA), and followed by a filter (wavelength selective switch-WSS) to reduce spontaneous emission noise. As shown in Fig. 2, the clear open electrical eye diagrams up to 70, 80, 90, 100 Gbit/s NRZ, and 40, 50, 60, 64 Gbaud PAM-4 are experimentally attained. The achieved large-signal eye diagram measurements in Fig. 2 indicate a favorable performance of Ge-on-Si PD with inductive gain peaking technique. We can believe that the proposed Ge-on-Si PD device possesses the great potential to achieve low-cost >100 Gbit/s data reception per lane for future 800 GbE transceiver.

class="figure_img" id="Figure2"/>
Download
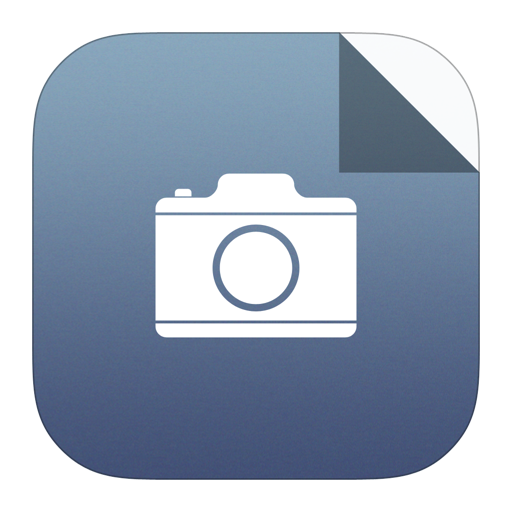
Larger image
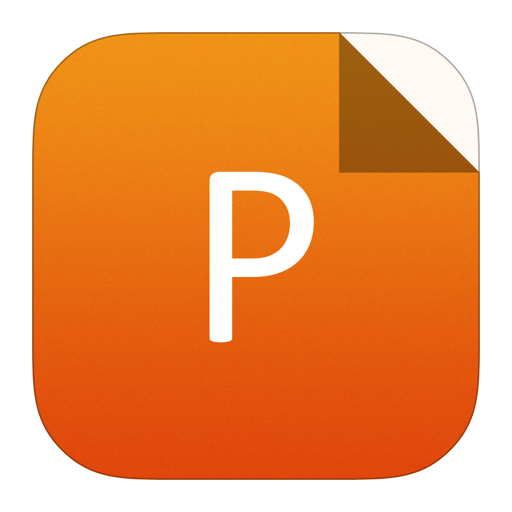
PowerPoint slide
Figure2.
(Color online) (a) Measured 70, 80, 90, and 100 Gbit/s NRZ eye diagrams under 3 V reverse-bias voltage. (b) Measured 40, 50, 60, and 64 Gbaud PAM-4 eye diagrams under 3 V reverse-bias voltage.
In summary, by employing inductive gain peaking technique for conventional vertical Ge-on-Si PD, the 3-dB bandwidth of 62 GHz are realized with 276 pH inductors. Benefitting from the suitable inductance, for the first time, the clear open electrical eye diagrams up to 70, 80, 90, 100 Gbit/s NRZ, and 40, 50, 60, 64 Gbaud PAM-4 are experimentally attained. It proves the efficiency of inductive gain peaking technique for high-speed integrated silicon photonics systems.
Acknowledgements
This work was supported by National Key Research and Development Program of China (2019YFB2205201, 2019YFB2205203) and Hubei Technological Innovation Project (2019AAA054).