1.
Introduction
Flexible and stretchable sensors can be uniformly attached to the surface of skin or organs due to exceptional features such as light weight, stretchability, low modulus, high flexibility and ultrathinness, thus enhancing the sensing properties of personal healthcare and human activity detection[1-4]. Due to these advantages, the wearable device market is expected to continue to grow in the next decade[5]. In order to promote the development of this new direction, a variety of flexible and stretchable sensors have been further developed by integrating new nanomaterials.
Nanofibers/nanowires, as a typical one-dimensional (1D) nanostructured material, has been widely concerned in recent years due to their good mechanical flexibility, with remarkable tolerance against mechanical bending and exceptionally low flexural rigidities, which make them a promising active material for flexible and stretchable sensors[6-12]. Apart from excellent mechanical flexibility and toughness, nanofibers/nanowires also have the characteristics of high possibility of surface functionalization, large surface area, good optical transmittances, a wide range of material selection and tunable porosity, overcoming the inherent property limitations of conventional active materials and makes them have good piezoresistive sensitivity, high carrier mobility and unique electrical properties[13-15]. In addition, nanofiber/nanowire have the 1D wire-shape features, so they can quantitatively identify some important parameters of wearable sensors such as possible crack characteristics, deformation degree, deformation mode and bending stiffness. Due to its large length–diameter ratio, the mechanical relaxation of 1D nanofiber/nanowire materials in the natural growth directions is much higher[16]. Under a given bending radius, the linear structure has a strong stress recovery ability. Compared with other nanostructures, the diameter of the linear structure is very small, and the deformation process is difficult to lead to the transverse formation of cracks. In view of the above advantages, nanofibers/nanowires have been extensively researched as building blocks in all kinds of flexible electronics, including flexible pressure sensor, flexible strain sensor, flexible gas sensor and flexible optical sensor and flexible biosensor[17-21].
Here, we focus on latest developments in the nanofibers/nanowires-based flexible and stretchable sensors with enhanced mechanical performance. First, we summarize the main advantages of using various types of 1D nanofibers/nanowires in flexible and stretchable sensors (Fig. 1). Then, we also comprehensively introduce some typical works of flexible and stretchable sensors applications of nanofiber/nanowires, involving pressure sensor and strain sensor. Finally, the future perspectives of flexible and stretchable sensors and the challenges and opportunities for future research directions are briefly discussed.

class="figure_img" id="Figure1"/>
Download
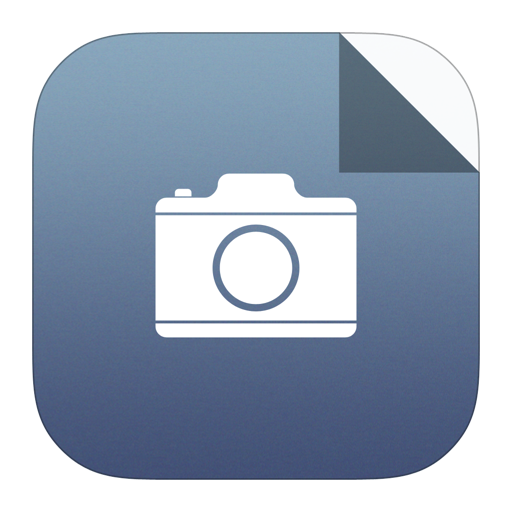
Larger image
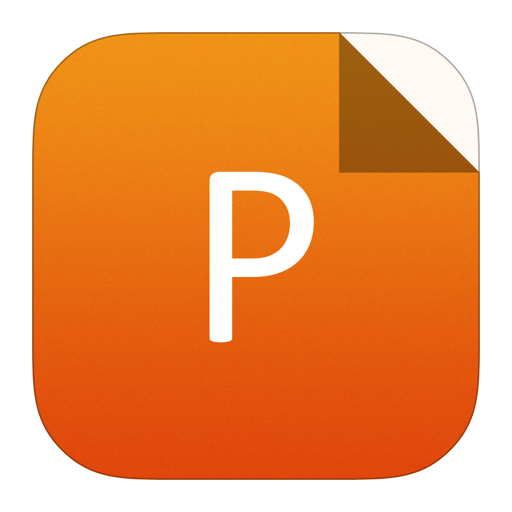
PowerPoint slide
Figure1.
(Color online) Schematic of nanofibers/nanowires-based flexible and stretchable sensors.
2.
1D nanostructure nanofiber/nanowire and their advantages
Nanofibers/nanowires materials as a typical one-dimensional nanostructured material, have two kinds of external dimensions: 1) nanoscale (less than or equal to 100 nm) and 2) larger three-dimensional dimensions[22]. Comparing with bulk materials, fibers have good thermal properties, high aspect ratio, excellent electrical conductivity, large specific surface area, optical properties, good mechanical flexibility and anisotropic physical properties, which make nanofiber/nanowire into a good active material for many special fields, such as flexible electronic, tissue engineering, sensor, oil/water separation, energy storage and intelligent actuator[23-27]. Nanofibers are attractive in flexible and stretchable sensors field for several reasons (Fig. 2).

class="figure_img" id="Figure2"/>
Download
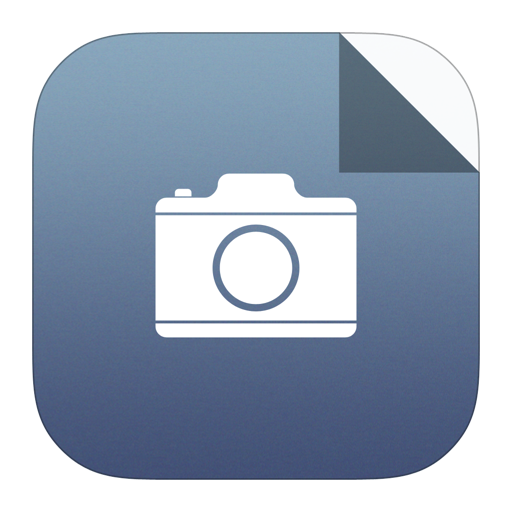
Larger image
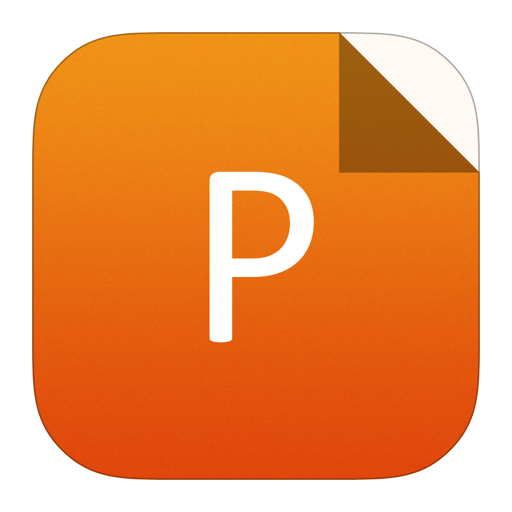
PowerPoint slide
Figure2.
(Color online) Graphical summaries of advantages of possible flexible and stretchable sensors application of 1D nanofibers/nanowires materials.
1) Relatively higher carrier mobility and size-dependent physical properties: because of the above properties, 1D nanofibers/nanowires have a wide range of potential applications in high-performance flexible devices. For example, the 1D single-walled carbon nanotube (SWCNT)-based sensor has aroused researcher’s enthusiasm. As a result, SWCNT is expected to become a candidate for high-performance electronic devices because of its good mechanical flexibility, high mobility (> 100 000 cm2 V–1s–1) and excellent optical transparency[28].
2) Large specific surface area: Compared with bulk materials, nanofibers show a larger specific surface area, which makes the adhesion of target objects, gases and external stimuli enhanced. For example, the surface area of the fiber with direct 5–500 nm is about 10 000–100 000 m2/kg[29]. About half of the molecules are on the surface of nanofibers with a diameter of 3 nm. Because most of the atoms are located on the surface of nanofibers, the inherent features of nanofibers/nanowire are different from those of traditional fibers, and most of the atoms of the latter are embedded in the fibers. The high specific surface area of nanofibers/nanowires offers significant ability for the release and attachment of functional groups, adsorbed molecules, ions, catalytic parts and nanoparticles[30].
3) 1D wire-shape structure, nanofiber or nanowire flexible electron reliable and effective conductive channel, can provide 1D path carrier. In addition, the effective mobility of flexible electronic devices is greatly improved because the carriers do not have to cross many boundaries between adjacent nanocrystals.
4) Large length–diameter ratio, 1D nanofibers and nanowires are more mechanically relaxed in their natural growth direction. Under a given bending radius, the linear structure has a strong stress recovery ability. Compared with other nanostructures, the diameter of the linear structure is very small, and the deformation process is difficult to lead to the transverse formation of cracks.
5) Small size, the size of the nanofiber/nanowire has a key influence on the response time of the system to external stimuli (e.g. gas, humidity, light, temperature, force and electricity). Based on NaBEt[31], we can expect to obtain higher rectifying performance and better electron transport in the nanowire at the same time via decreasing the size of the conducting wire[32]. This result is mainly due to the geometric surface or inherent conductivity effect and bulk density effect of the nanofiber/nanowire, or both are the result of the decrease of the nanofiber/nanowire diameter. Similar principle can also be applied to the size effect of nanofibers sensitive to other types of stimuli.
3.
Flexible and stretchable sensor applications of 1D nanofibers/nanowires
The response of flexible and stretchable electronic devices to different external stimuli mainly depends on the active materials[33-35]. For example, one-dimensional nanofibers and nanowires with large aspect ratio and good mechanical properties are widely used as sensitive materials for high-performance flexible and stretchable sensors. For flexible and stretchable sensors, the noble metal nanowires, polymer nanofibers and oxide nanowires are selected as sensing layers. In this section, the latest development of one-dimensional nanofiber/nanowire-based flexible stretchable devices is summarized (Table 1). Two types of mechanical devices, flexible pressure sensor and flexible stretchable sensor, are mainly introduced.
Material | Structure | Application | Reference |
Poly(VDF-TrFE) | Nanofiber | Pressure sensor | [36] |
Carbon nanofiber | Nanofiber | Strain sensor | [37] |
Silk | Nanofiber | Pressure sensor | [38] |
Poly(l-lactic acid) | Nanofiber | Pressure sensor | [39] |
Poly(3-hexylthiophene) | Nanofiber | Pressure sensor | [40] |
Cu/Ni | Nanofiber | Pressure sensor | [41] |
GO/PVDF | Nanofiber | Pressure sensor | [42] |
Polyacrylonitrile | Nanofiber | Pressure sensor | [43] |
GO/PU | Nanofiber | Pressure sensor | [44] |
Strain sensor | |||
Polyvinylidene fluoride/Ag | Nanofiber | Pressure sensor | [45] |
PDMS/Carbon | Nanofiber | Strain sensor | [46] |
PVDF/Graphene | Nanofiber | Pressure sensor | [47] |
ZnO/PVDF | Nanofiber | Pressure sensor | [48] |
SiO2/Graphene | Nanofiber | Strain sensor | [49] |
Carbon/Graphene | Nanofiber | Strain sensor | [50] |
PDMS ion gel /PVDF-HFP | Nanofiber | Pressure sensor | [51] |
Ag | Nanowire | Strain sensor | [52] |
ZnO | Nanowire | Pressure sensor | [53] |
ZnO/Graphene | Nanowire | Pressure sensor | [54] |
ZnO | Nanowire | Strain sensor | [55] |
GaN | Nanowire | Strain sensor | [56] |
Ag | Nanowire | Pressure sensor | [57] |
Gan/ZnO | Nanowire | Pressure sensor | [58] |
Cu | Nanowire | Pressure sensor | [59] |
Ag | Nanowire | Strain sensor | [60] |
PVA/PPy | Nanowire | Pressure sensor | [61] |
PMN-PT | Nanowire | Pressure sensor | [62] |
Table1.
Summary of various 1D nanostructure materials based flexible and stretchable sensors.
Table options
-->

Download as CSV
Material | Structure | Application | Reference |
Poly(VDF-TrFE) | Nanofiber | Pressure sensor | [36] |
Carbon nanofiber | Nanofiber | Strain sensor | [37] |
Silk | Nanofiber | Pressure sensor | [38] |
Poly(l-lactic acid) | Nanofiber | Pressure sensor | [39] |
Poly(3-hexylthiophene) | Nanofiber | Pressure sensor | [40] |
Cu/Ni | Nanofiber | Pressure sensor | [41] |
GO/PVDF | Nanofiber | Pressure sensor | [42] |
Polyacrylonitrile | Nanofiber | Pressure sensor | [43] |
GO/PU | Nanofiber | Pressure sensor | [44] |
Strain sensor | |||
Polyvinylidene fluoride/Ag | Nanofiber | Pressure sensor | [45] |
PDMS/Carbon | Nanofiber | Strain sensor | [46] |
PVDF/Graphene | Nanofiber | Pressure sensor | [47] |
ZnO/PVDF | Nanofiber | Pressure sensor | [48] |
SiO2/Graphene | Nanofiber | Strain sensor | [49] |
Carbon/Graphene | Nanofiber | Strain sensor | [50] |
PDMS ion gel /PVDF-HFP | Nanofiber | Pressure sensor | [51] |
Ag | Nanowire | Strain sensor | [52] |
ZnO | Nanowire | Pressure sensor | [53] |
ZnO/Graphene | Nanowire | Pressure sensor | [54] |
ZnO | Nanowire | Strain sensor | [55] |
GaN | Nanowire | Strain sensor | [56] |
Ag | Nanowire | Pressure sensor | [57] |
Gan/ZnO | Nanowire | Pressure sensor | [58] |
Cu | Nanowire | Pressure sensor | [59] |
Ag | Nanowire | Strain sensor | [60] |
PVA/PPy | Nanowire | Pressure sensor | [61] |
PMN-PT | Nanowire | Pressure sensor | [62] |
3.1
1D nanofibers/nanowires for flexible pressure sensor
The application of next generation pressure sensor is gradually expanding to intelligent artificial limb, humanoid robot, wearable devices and electronic skin (e-skin)[63-65]. Flexible and stretchable sensor can monitor small changes in pressure or strain caused by vital signs (e.g. respiration, muscle contraction or pulse), and can also be designed as multi-functional medical monitoring equipment[66]. For most practical applications, excellent sensitive ability is the basic characteristic of high-performance flexible pressure sensor. Recently, 1D nanostructures, including metal nanowires, polymer nanofibers, carbon nanotubes, and oxide nanowires, have been widely used in constructing advanced wearable pressure sensor due to their excellent mechanically flexible yet robust and good electronic performance[67-69]. For instance, Au nanowires-based flexible pressure sensors were fabricated by Cheng and his group (Fig. 3(a))[70]. Generally, the whole manufacturing process of the device is scalable, which can be easily integrated in a large area and graphically drawn to draw the spatial pressure distribution (Fig. 3(b)). Furthermore, Au nanowire (NW) based sensors can respond to pressure changes at a frequency of up to 5.5 Hz in 0.05 s. These results show that the superior sensing characteristics combined with good mechanical properties (such as mechanical flexibility and robustness) enable real-time detection of pulse changes and monitor of small vibration force from music (Figs. 3(c)–3(f)). In 2019, their team also demonstrated a vertically arranged gold nanowires (v-Au NWs)-based high-performance wearable pressure sensor (Figs. 3(g) and 3(h))[71]. Due to its wide electrochemical window, good chemical inertness, biocompatibility and tunable conductivity, the pressure sensor has low working voltage of 0.1 V, high durability of signal stability of 10 000 cycles test, high sensitivity in low voltage region and fast response time of less than 10 ms (Fig. 3(i)). The sensor can detect the pulse signal of radial artery seamlessly, which provides a non-invasive solution for real-time wireless health detection. In addition, the integration of ink-jet printing electrode array and v-Au nanowire-based pressure-sensitive layer can realize spatial pressure mapping. Sun et al.[59] also successfully synthesized the Cu nanowire aerogel as a flexible pressure sensor. The as-fabricated flexible pressure sensor exhibits high pressure-sensing response (0.7 kPa?1). More importantly, the flexible pressure sensor based on Cu nanowire aerogel has the advantages of light weight, high sensitivity and convenient fabrication. It is very suitable for potential applications as flexible electronic devices, e-skin, and other devices.

class="figure_img" id="Figure3"/>
Download
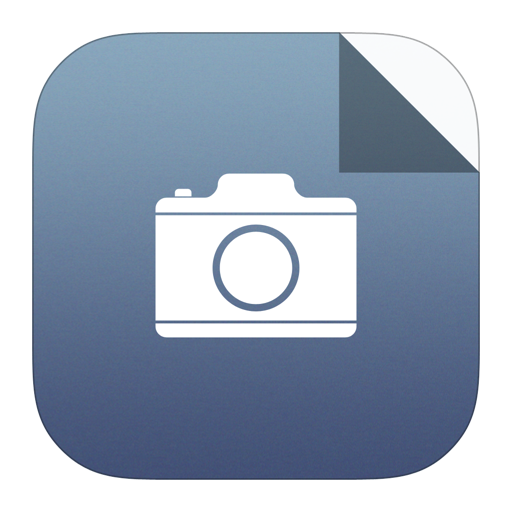
Larger image
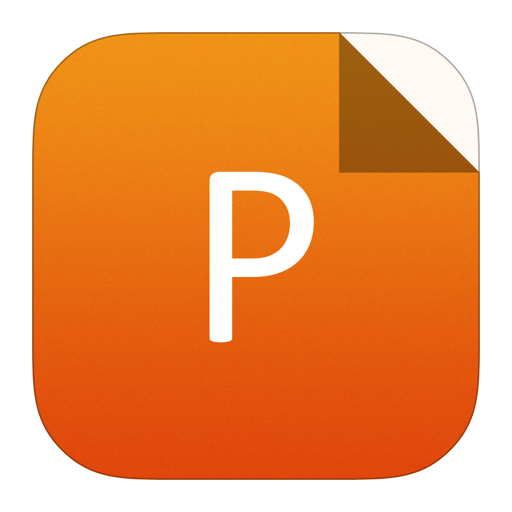
PowerPoint slide
Figure3.
(Color online) (a) Illustration of the preparation and structure of the Au nanowires-based flexible pressure sensor. (b) Optical image of the Au nanowires-based flexible pressure sensor. Inset show the SEM image of Au nanowires (scale bar, 100 mm). (c) Optical image of device attached on the wrist. (d) pulse change records of device attached on the wrist. (e) Schematic illustration of the setup for acoustic vibration sensing. (f) The current responses to the acoustic vibrations from a piece of music. Reproduced from Ref. [70] with permission, Copyright 2014, Macmillan Publishers Limited. (g) Illustration of the preparation process of vertically arranged gold nanowires on microstructured PDMS films. (h) Schematic showing the sensor structure. (i) Response time and recovery time of vertically arranged gold nanowires-based flexible pressure sensor under both loading and unloading conditions. Reproduced from Ref. [71] with permission, Copyright 2019, American Chemical Society.
In addition to the metal NWs, polymer nanofibers have also been used as the sensing materials for flexible pressure sensor. Recently, Lou et al.[72] introduced a simple and effective method of making p(VDF-TrFE) nanofiber wrapped by rGO nanoflakes, forming a good 3D network conductive film, which can be easily used to make flexible piezoresistive pressure sensor, as shown in Figs. 4(a)-4(c). Due to the viscoelastic property of PVDF nanofibers and good pressure-sensing performances of rGO, the flexible pressure sensor shows a rapid response speed (5 ms), low detection limit (1.2 Pa) and high sensitivity (15.6 kPa–1) (Figs. 4(d) and 4(e)). The device can also be used as a e-skin to test different forces, such as bending and twisting, and to monitor physiological signals, including blood pressure pulses and muscle movements (Figs. 4(f) and 4(g)). Looking forward to the future, the research methods of low-cost materials and devices will be of great value to the application of wearable electronic technology in artificial skin, disease prevention and human-computer interface. Recently, Xia and his colleagues[73] prepared a PVDF-PVDF/Ag nanofiber-based flexible pressure sensor by using PET/ITO electrodes. In addition, the sensor also realizes non-contact pressure sensor, which widens the future development of flexible sensors. This study offers a cheap, simple and novel approach for the preparation of flexible pressure sensor, and a novel method for the real-time motion monitoring of wearable electronic devices in the future.

class="figure_img" id="Figure4"/>
Download
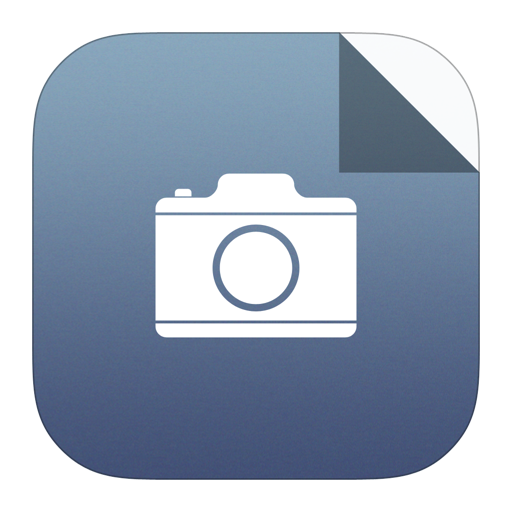
Larger image
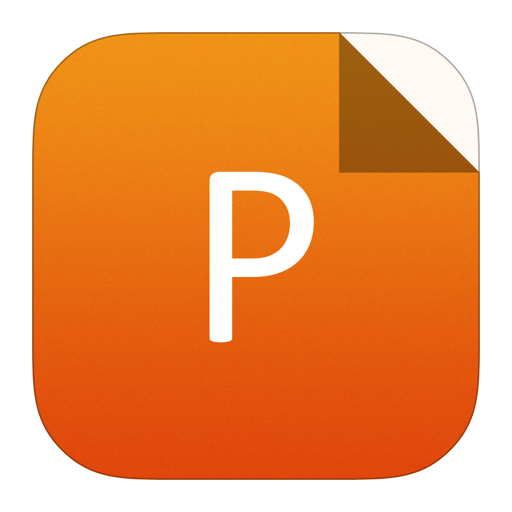
PowerPoint slide
Figure4.
(Color online) (a) Illustration of fabrication process of rGO/PVDF nanofibers-based flexible pressure sensor. (b) SEM image of rGO/PVDF nanofibers. (c) Illustration of a flexible pressure sensor structure. (d) Sensitivity of rGO/PVDF nanofibers-based flexible pressure sensor under different force conditions. (e) dynamic response curves of rGO/PVDF nanofibers-based flexible pressure sensor for different objects. (f, g) Response curves of rGO/PVDF nanofibers-based flexible pressure sensor attached on the wrist under different condition. Reproduced from Ref. [72] with permission, Copyright 2016, Elsevier B.V. (h, i) Structure and fabrication process of the PVR system. SEM image of the as-obtain (j) ZnO nanowire and (k) WO3 film array. Enlarged SEM images of (j1, j2) the ZnO nanowire before and after spin-coating the photoresist layer and (k1, k2) The WO3 film deposited on the ITO electrode. Reproduced from Ref. [74] with permission, Copyright 2017, WILEY-VCH Verlag GmbH & Co. KGaA, Weinheim. (l) Pressure response of the PTNWs/G-based sensors. (m) dynamic pressure-sensing curves of the PTNWs/G-based sensors. Reproduced from Ref. [75] with permission, Copyright 2017, American Chemical Society.
Oxide NWs with piezoelectric properties have also been used in flexile self-powered pressure sensors. A pressure display and recording (PVR) system is developed by Han et al. as shown in Figs. 4(h)–4(k) [74]. By combining the WO3 film electrochromic device (ECD) array and ZnO nanowire matrix pressure sensor (ZPSs), the applied pressure can be directly detected, displayed and recorded. In PVR system, each pixel of ECD array is connected to a group of ZnO NWs by connecting electrodes. Due to the electrochromic characteristics of WO3, the pressure exerted on the ZnO nanowires will produce piezoelectric charges, which change the color of the corresponding pixels in the ECD array. Therefore, the change of color can distinguish the distribution of different pressure. Chen et al.[75] reported PbTiO3 nanowire/graphene heterostructure-based a static flexible pressure device. When an external force is applied to the sensor surface, the induced polaron in the piezoelectric PbTiO3 NW acts as a charged impurity, which affects the mobility of the carrier in graphene. the device has higher static pressure-sensing response (9.4 × 10–3 kPa–1) and faster response time (5–7 ms) compared with the traditional piezoelectric pressure sensor, as displayed in Figs. 4(l) and 4(m).
3.2
1D nanofibers/nanowires for flexible strain sensor
Highly sensitive and stretchable flexible strain sensor can test the whole range of human motion, which is an important part of many specific fields, such as soft robot and human–machine, real-time record of human daily physical activity and personalized health care monitoring[76-80]. Highly sensitive flexible strain devices must be able to integrate consistently with any soft skin or clothing that is bent and moving to precisely test external force stimuli for health monitoring and disease diagnosis[81-84]. The use of sensing materials based on 1D nanostructure, such as nanofiber, CNT, AuNWs, and AgNWs coated on ?exible substrates, provides good gauge factor ranges in working strain ranges. By employing different structures, complex strains are produced in the elastic matrix during the tensile process, which leads to different density distribution of cracks, so as to achieve the purpose of controlling the strain-sensing response. For instance, Chen et al.[85] developed a high tensile conductive P(VDF-TrFE) polymer nanofiber/Ag nanowire-based strain sensor, as shown in Figs. 5(a) and 5(b). The P(VDF-TrFE) enhanced fiber sensor has a high range coefficient of 5.3, over 100% super tensile property, excellent durability after 10000 strain cycles and 20 ms fast response time. Superior performance enables us to accurately and timely monitor vigorous exercise (finger bending or stretching) and subtle physiological signals (pulse and voice) (Figs. 5(c) and 5(d)). Due to the superior performance of detecting finger joint movement, an intelligent data glove is made to detect gesture language, which proves the practicability of optical P(VDF-TrFE) nanofiber-based strain device for flexible electronic products as shown in Fig. 5(e). Similarly, Park and his team[60] demonstrated an Ag nanowire networks and PDMS elastomer sandwich structures-based flexible and stretchable sensor (Figs. 5(f)–5(i)). The strain sensor displays excellent tensile properties for both dynamic and static forces, even at the large strain level (ε > 40%), with high linearity and negligible hysteresis, and has the strong piezoresistance of tunable gauge factors (GFs) in the range of 2–14, which depends on the maximum tensile strength of Ag nanowires up to 70% density (Figs. 5(j) and 5(k)). 3D resistance network model is used to calculate the response of the sensor. The orientation and position evolution of PDMS encapsulated Ag nanowires to various strain were calculated. This research will open new applications in flexible and stretchable electronic products, especially in human motion detection applications, strain sensors should accommodate very large strain.

class="figure_img" id="Figure5"/>
Download
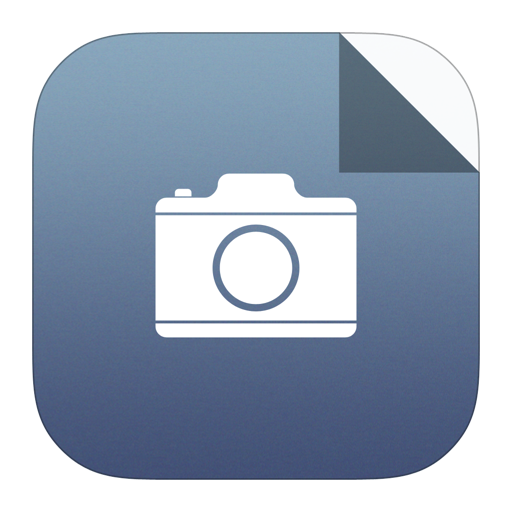
Larger image
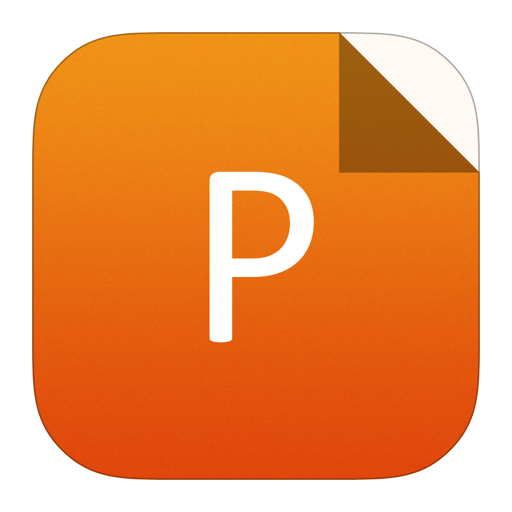
PowerPoint slide
Figure5.
(Color online) (a) Schematic structure and (b) high-magnification SEM image of the P(VDF-TrFE)-based conductive fiber. (c) Pulse and (d) spoke response curves of the P(VDF-TrFE)-based fibrous sensor. (e) A data glove fixed with ten-fiber strain sensors. Reproduced from Ref. [85] with permission, Copyright 2016, WILEY-VCH Verlag GmbH & Co. KGaA, Weinheim. (f) Illustration of preparation process and (g) optical image of Ag nanowires/PDMS-based sandwich-structured strain sensors. (h) Optical image of the Ag nanowires/PDMS-based strain sensor under bending and twisting. (i) optical images on top and cross-section of Ag nanowires/PDMS-based strain sensor. (j) Finger motion detection and (k) smart glove system by Ag nanowires/PDMS-based sandwich-structured strain sensors. Reproduced from Ref. [60] with permission, Copyright 2014, American Chemical Society.
CNT represents the basis of most traditional tensile strain sensors because its performance far exceeds that of known organic semiconductor and metal/metal oxide-based flexible sensors[86]. Notably, SWCNTs-based strain sensor exhibits high internal piezoresistivity. For example, Hata et al.[87] reported a class of flexible and stretchable sensors made of aligned SWCNT films, as shown in Figs. 6(a)–6(c). By this method, a new strain sensor has been developed, which can detect and endure strain up to 280%, fast response (14 ms delay time), low creep (3.0% at 100% strain), and high durability (10 000 cycles at 150% strain). Because of the structure of the strain sensor, the bandage and SWCNT membrane sensor are both single and stretchable objects, so SWCNT membrane can directly and accurately monitor skin deformation (Fig. 6(d)). Moreover, the incorporation of SWCNT-based strain sensors creates a platform for examining human body structure, as illustrated via data gloves made of five paralleled SWCNT-based devices fitted to one glove (Fig. 6(e)). Data glove is an interactive sensor, similar to gloves usually worn on hands, which is helpful for fine motion control of virtual reality and robots. Very recently, Lee and his group[88] have effectively fabricated a directional response an asterisk shaped CNT sensing-based flexible strain sensor to identify the direction of applied stress, thus overcoming some shortcomings of existing sensors (Figs. 6(f)–6(i)). The design overcomes the limitation that traditional flexible strain sensor can only detect strain in a specific direction. With the increase of the external stress applied on the carbon nanotube star strain sensor, the resistance also increases. According to the strain, the resistance increases in the linear, threshold or saturation response range. They also proved that the sensor can be used to identify the moving direction of the brush used and the tilt amplitude and direction of the self-made control lever installed on the sensor equipment (Figs. 6(j) and 6(k)). This study confirms that carbon nanotube-based strain sensors are expected to be used in cost-effective and easy to manufacture wearable electronic products to prevent potential risks from the external environment.

class="figure_img" id="Figure6"/>
Download
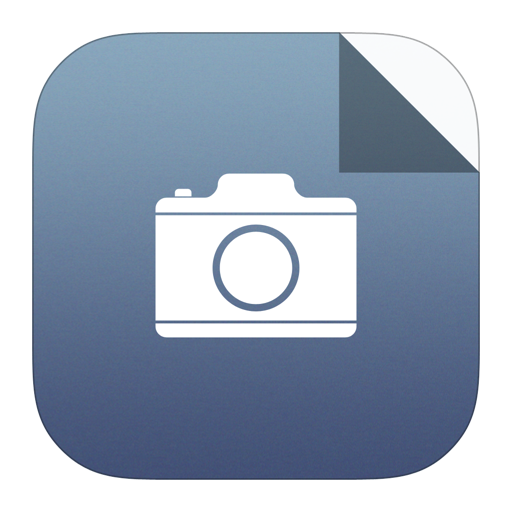
Larger image
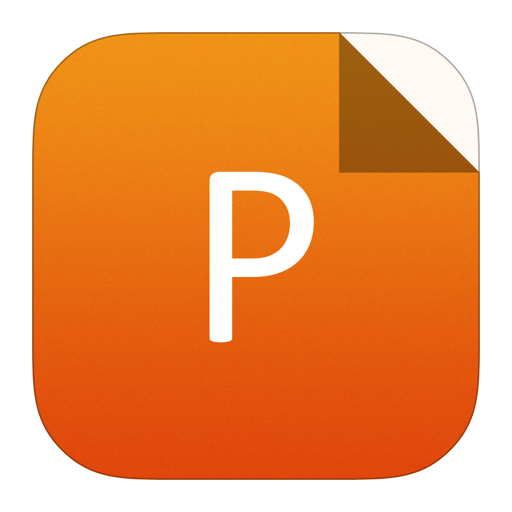
PowerPoint slide
Figure6.
(Color online) (a) Illustration of fabrication process of SWCNT-based strain sensor. (b) Optical image of the SWCNT-based strain sensor under strain. (c) SEM image of SWCNT. (d) Stretchable wearable sensors on the human body. (e) Finger motion detection of stretchable wearable sensors. Reproduced from Ref. [87] with permission, Copyright 2011, Macmillan Publishers Limited. (f) Illustration of fabrication process of CNT-based strain sensor. (g) Optical images of the CNT-based strain sensor. (h) Top-view and (i) cross-section view SEM image of CNT materials. (j) Brushing test and (k) joystick movements of CNT-based strain sensors. Reproduced from Ref. [88] with permission, Copyright 2019, The Royal Society of Chemistry.
4.
Conclusion
In this review, we summarized the latest research work of 1D nanofiber/nanowire in this field of flexible and stretchable sensors (such as flexible pressure sensor and strain sensor). The exciting results highlighted in this paper confirm that their unique one-dimensional structure and good mechanical toughness and flexibility have strong advantages in the field of flexible and stretchable sensors. We also summarize the application of one-dimensional nanofibers and nanowires in flexible sensors and the performance of advanced flexible and stretchable sensors made of this kind of nanomaterials. The exciting results obtained so far can arouse researchers' interest in the research of new high performance flexible electronic devices.
To further improve the performance of flexible and stretchable sensors, the rationalization and design of one-dimensional nanofibers and nanowires should be paid more attention. Although one-dimensional nano materials have been widely used in the field of flexible sensors, there are still some challenges in improving their performance. 1) The effects of structure graphing and controllability on device performance are obvious. Therefore, it is urgent to adjust the assembly and orientation device of one-dimensional structure more precisely, so that it has high deformation performance. 2) In order to manufacture and integrate flexible and stretchable sensors, the yield of one-dimensional nanostructures is still significantly insufficient. At present, one-dimensional nanostructure assembly technology has advantages and disadvantages. In this case, printing one-dimensional nanostructures on flexible substrates will bring some problems. Therefore, a more suitable one-dimensional nanostructure assembly method is needed to provide high-quality one-dimensional nanofibers and nanowires for flexible and stretchable sensors.
Acknowledgements
The authors sincerely acknowledge financial support from the National Natural Science Foundation of China (NSFC Grant No. 61625404), the Science and Technology Development Plan of Jilin Province (20190103135JH) and Young Elite Scientists Sponsorship Program by CAST (2018QNRC001).