1.
Introduction
High-efficiency semiconductor light-emitting diodes (LEDs) are now widely used in the field of display, lighting, etc. to replace the traditional incandescent or fluorescent lamp for energy saving[1, 2]. Recently, the wavelength of LEDs has been investigated to extend from visible light to ultraviolet (UV) and deep UV by increasing the Al component to raise the energy bandgap of AlGaN[3]. Besides this, the dimension of LEDs is shrinking from the order of a millimeter or hundreds of microns to several microns to improve the resolution in display applications[4, 5]. However, due to the rigid substrate of such LEDs, the current structure could not be adapted to flexible applications, such as lighting on wearable device, display on google glasses, robotics, biomedicine, etc.[6, 7].
Organic light-emitting diodes (OLED) are naturally flexible[8-10]. However, the efficiency and especially the reliability still cannot compete with solid-state LEDs, which limits their real applications[11]. Recently, 2D materials have attracted much attention. Due to their atomic-layer thickness, they are also naturally a type of flexible material. 2D material-based flexible photodetectors are reported to achieve ultrahigh sensitivity by several groups[12-15]. However, rare reports can be found in the field of lighting with high efficiency. Right now, inorganic LEDs are still the workhorse for solid-state lighting and many other applications. Hence, inorganic rigid semiconductors as lighting material are still the best choice for flexible LEDs, which is realized by flexible electrical connection.
The wavelength of quaternary material AlGaInP LED ranges from 560 to 650 nm at room temperature, which is widely applied in the fields of display, lighting, etc. For the flexible type, Rogers’ group developed a type of waterproof AlGaInP LED on a flexible substrate for biomedical and robotics applications[16]. After that, Gao’ group also developed a flexible AlGaInP LED in China[17]. Both of them utilized a mesa structure in order to avoid additional transfer process. However, mesa structure will cause current crowding at the corner of the mesa where heat generates. AlGaInP is very sensitive to temperature, which decreases the efficiency of the LEDs. Furthermore, due to the transfer process, the yield is relatively low because the wafer is very thin (~25 μm) after releasing from the GaAs substrate. Any strain on it may make the wafer break. Also, such transfer process is not applicable for GaN LEDs which use sapphire as substrate.
In the large-scale LED manufacture process, LED chips will be put on a piece of blue PVC film after dicing. The space between the adjacent chips can be controlled by uniform pulling force applied on the heated PVC film for both AlGaInP and GaN LEDs. In this paper, a 4 × 4 flexible AlGaInP LED array is fabricated by transferring the LED chips from the blue PVC films to flexible polyimide substrate directly. Such a fabrication process is much easier, mature and high-yield compared with the previously reported methods.
2.
Experiment
Figs. 1(a)–1(f) illustrate the fabrication process of the flexible AlGaInP LEDs. Firstly, a Ti/Au (15 nm/150 nm) layer is deposited on the polyimide substrate (DuPont, Kapton 500HN), which has excellent physical, chemical and electrical performance. After photolithography, the bottom n-electrodes are patterned on the polyimide substrate. The square bottom n-electrodes are connected by straight metal lines with a width of 200 μm and length of 430 μm. After conductive silver paste is applied on the center of the Ti/Au electrodes, the center of the LED chips stuck on the PVC film are aligned to the center of the bottom n-electrodes on the polyimide film with pressure under the microscope. Then it is put into an oven at 150 °C for 1 h for curing. The PVC film can be peeled easily from the chips because the viscosity of PVC film decreases a lot due to the high temperature. The LED chips finish by being transferred to the polyimide substrate and electrical connection. Then BCB (benzocyclobutene) is coated on the top for electronic isolation between the p- and n-electrodes. After heating for around 1 h with gradient rising temperature, the liquid BCB becomes transparent, which indicates little absorption. After exposing the top electrode of LEDs by photolithography, Ti/Au (15 nm/150 nm) layers are deposited on the BCB layer. Finally, the p-electrodes are connected by metal lines after photolithography and wet etching. The 4 × 4 array is formed, and the schematic cross section is illustrated in Fig. 1(g).

class="figure_img" id="Figure1"/>
Download
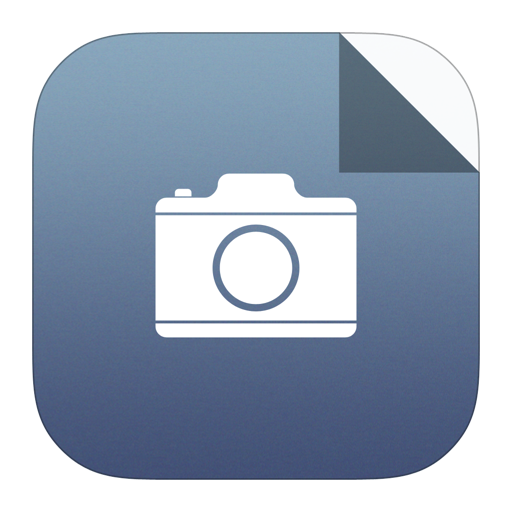
Larger image
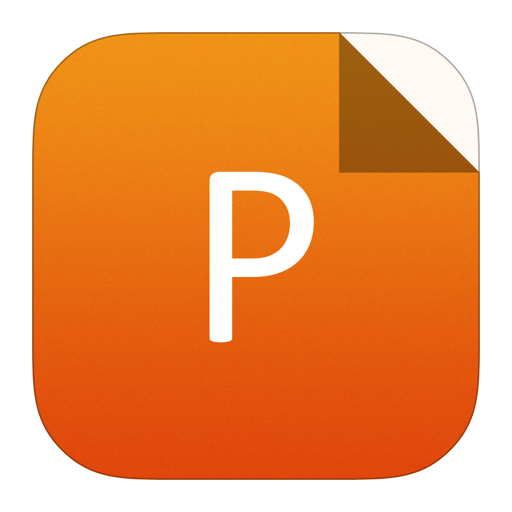
PowerPoint slide
Figure1.
(Color online) Illustration of fabrication process of flexible LEDs. (a) After Ti/Au layer deposition and photolithography, the bottom n-electrodes are patterned on the polyimide substrate. (b) Application of conductive silver paste. (c) Transfer of LED chips from PVC film to polyimide substrate. (d) BCB coating and then photolithography to expose the top electrodes of LEDs. (e) Top metal layer deposition. (f) Patterned top p-electrodes after photolithography. (g) Schematic cross section of flexible LEDs.
3.
Results and discussion
Fig. 2(a) shows the emission image of 4 × 4 arrays under the current injection of 1 mA taken by the metallurgical microscope. All the LED chips are illuminating uniformly. Figs. 2(b) and 2(c) demonstrate the side-view images of the LED array with concave and convex bending. The emission intensity is constant and is also not influenced by the bending process.

class="figure_img" id="Figure2"/>
Download
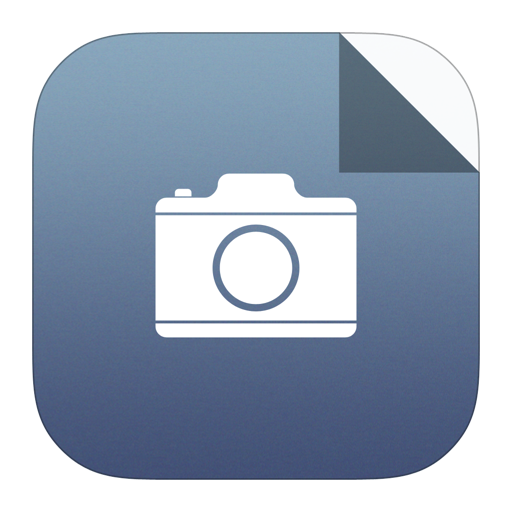
Larger image
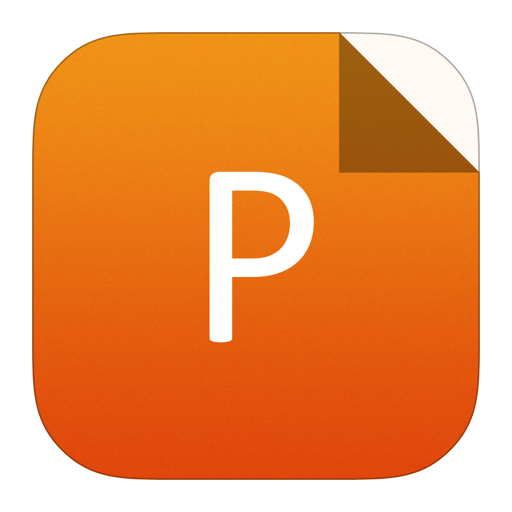
PowerPoint slide
Figure2.
(Color online) (a) Metallurgical microscope image of the transferred LED arrays under the current injection of 1 mA. (b) and (c) Side-view images of concave and convex bent LED arrays, respectively.
Fig. 3(a) compares the forward current–voltage (I–V) curves of transferred LEDs when it is flat (before bending) and bent with curvature of 5, 8, and 11 mm, respectively. At 20 mA, the voltage drop is around 2 V. And the ideality factor is 2.10, 2.12, 2.09, and 2.11, respectively, with the corresponding series resistance of 7.3, 6.7, 7.1, and 7.1 Ω. However, it can be seen that the I–V curves can almost coincide together, which indicates the bending process has little effect on the electrical performance due to the better ductibility of Au. Fig. 3(b) compares the electroluminescence (EL) spectra of transferred LEDs before and after bending, measured by integrating sphere from Ocean optics. The bending curvature is 11 mm. The peak of the EL spectra is 635.7 and 637.6 nm, respectively. The red-shift of the EL spectra is due to the heat during the continuous measurement. Bending only occurs in the flexible metal electrodes, which will not affect the characteristics of the LED chips. However, in the case of bending LED chips, it will introduce mechanical damage to the AlGaInP film, which will damage its performance. In the case of GaN-based LEDs, bending affects the internal quantum efficiency and emission wavelength, which adds up additional functionality of flexible LEDs[18, 19].

class="figure_img" id="Figure3"/>
Download
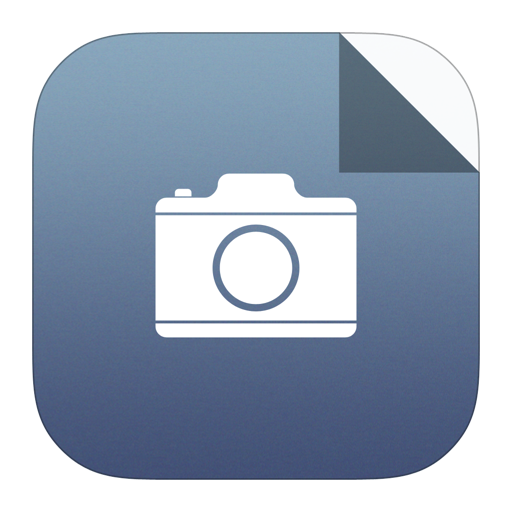
Larger image
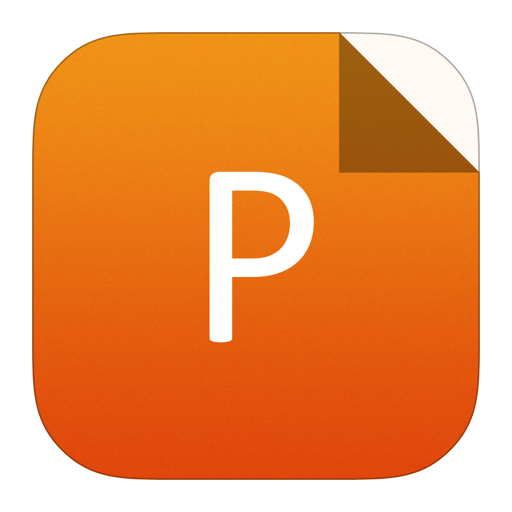
PowerPoint slide
Figure3.
(Color online) (a) Comparison of forward I–V characteristics of transferred LED array before and after bending with the curvature of 5, 8, and 11 mm, respectively. The voltage drop is 2 V under the current injection of 20 mA. (b) EL spectra comparison of the transferred LED under the current injection of 5 mA before and after bending. The red-shift of peak wavelength is due to heat.
4.
Conclusion
In this paper, we proposed a fabrication process of flexible LED array by utilizing a mature LED manufacture technique. The key point is transferring the LED chips on the blue PVC film to the polyimide film directly. The electrical and optical performances keep well after transfer process even in the bending state. Such method keeps good yield and good optoelectronic performance at the same time.
Acknowledgments
This work was supported by the National Key R&D Program of China (2016YFB0400603).