1.
Introduction
Flexible sensors have been widely used in various types of electronic devices. Their advantage lies in their high flexibility, ability to adapt to various complex environments, and broad development prospects. Flexible sensors with nanomaterials have a larger specific surface area to further enhance sensor performance. There are several ways to make nanomaterials. Among them, electrospinning provides a low-cost, large-scale method for preparing flexible nanofibers. The morphology of the fibers can be adjusted during the electrospinning process to obtain different structures[1–4]. The electrospun fiber membrane is easily attached to the electrode material, simplifying the assembly steps of the sensor. Electrospinning enables the preparation of a variety of materials that respond to the external environment, including temperature[5], electromagnetic field[6], pressure[7], light[8, 9], humidity[10], pH[11], and drugs[12, 13]. The surface wettability of some materials can be altered by electrospinning, which expands the range of applications for related materials[14]. Moreover, the electrospun fiber membrane has high porosity and good gas permeability and can be used in the medical field[15, 16]. For these reasons electrospinning has wide application prospects in various electronic devices such as wearable devices.
In this review, we summarize the progress in the preparation of flexible sensors by electrospinning. Photodetectors, stress sensors, gas sensors and nanogenerators were introduced. The prospect of flexible sensors and electrospinning were discussed towards the end.
2.
Electrospinning
Electrospinning devices typically consist of a propulsion pump, a high voltage power supply, and a collection plate. When the voltage applied at the end of the needle exceeds a certain critical value, the charge repulsion on the surface of the solution exceeds its surface tension, and the jet formed at the end of the needle is deposited on the collecting plate under the action of an electric field to form a fiber membrane. The parameters used in the electrospinning process will affect the morphology of the fiber to some extent. An increase in the spinning voltage, a decrease in the advancement speed, and an increase in the distance between the electrodes and the collection plate can reduce the diameter of the resulting nanofiber. There are usually two methods for preparing conductive nanofibers by electrospinning, as shown in Fig. 1[17, 18]. The fibers obtained by electrospinning generally have flexibility. However, the solution used for electrospinning needs to have a certain viscosity, and a polymer solution is often used. This causes the fibers obtained by electrospinning to be subjected to subsequent processing to improve their electrical conductivity.

class="figure_img" id="Figure1"/>
Download
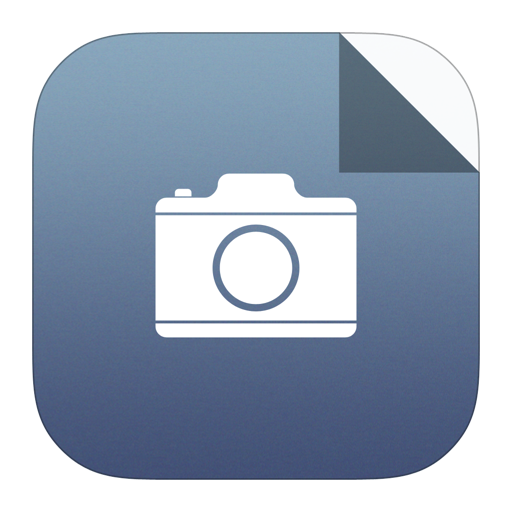
Larger image
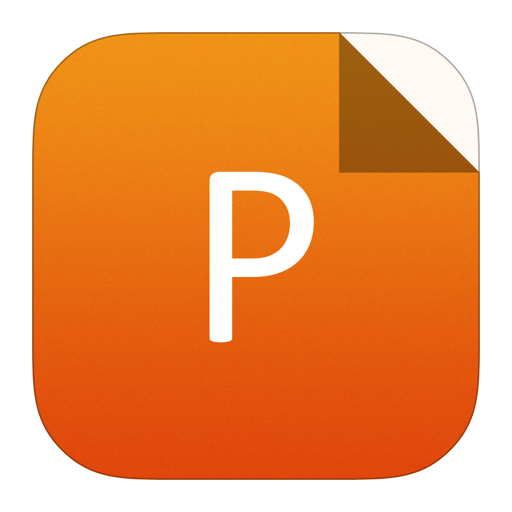
PowerPoint slide
Figure1.
(Color online) (a) Flowchart for preparing conductive nanofibers by surface modification[18]. (b) Flowchart for preparing conductive nanofibers by heat treatment[17].
3.
Strategies for preparing conductive nanofibers
3.1
Surface treatment
An electrospun fiber is used as a template, and a metal material such as gold or copper is deposited thereon to obtain a conductive fiber. The electrospinning film can also be removed by sintering, dissolution, or the like in a subsequent step. Similarly, conductive fibers can also be obtained by chemically depositing or coating a polymer conductive material, carbon nanotubes or the like onto the surface of the fiber membrane. This method of treating only the surface of the fiber causes the conductive material to form a cylindrical structure, increase the specific surface area, and have a certain conductivity. This also increases their sensitivity as a gas sensor.
3.2
Precursor heat treatment
Conductive fibers can also be obtained by heat treating a precursor of a certain material. For example, the carbon fiber is obtained by electrospinning a polyacrylonitrile (PAN) solution of N,N-dimethylformamide (DMF) and heat treatment. Mixing a precursor thereof with a polymer solution and electrospinning, then sintering in an air atmosphere to remove components such as a polymer can obtain an inorganic oxide fiber. This method can completely remove the polymer component and obtain a continuous conductive fiber.
4.
Application
4.1
Photodetector
Due to its photoelectric effect, semiconductors can be exposed to light and cause a change in conductivity. The light intensity can be characterized by changes in material current. Zhang et al.[19] prepared La-doped p-type ZnO nanofibers by adding rare earth element La to the electrospun zinc oxide precursor solution. 0.2 g of cesium acetate (C6H9O6La), 1.0 g of zinc acetate (C4H6O4Zn·2H2O) and 1.5 g of polyvinylpyrrolidone (PVP) were mixed with 0.2 g of water (H2O) and 8.5 g of ethanol (C2H6O), and then electrospun fibers were obtained by electrospinning, followed by heat treatment to obtain p-type ZnO fibers. Later, Liu et al.[20, 21] also obtained Ce-doped p-type ZnO nanofibers by doping the rare earth element Ce. As shown in Fig. 2[19, 21], these fibers all have good optoelectronic properties. Liu et al.[22] developed the printing process for printing ZnO nanofibers using near-field electrospinning. A near-field electrospinning and printer were combined to design a printing process. As shown in Fig. 3[22], The resulting ZnO fiber array has good photoelectric response and detection rate.

class="figure_img" id="Figure2"/>
Download
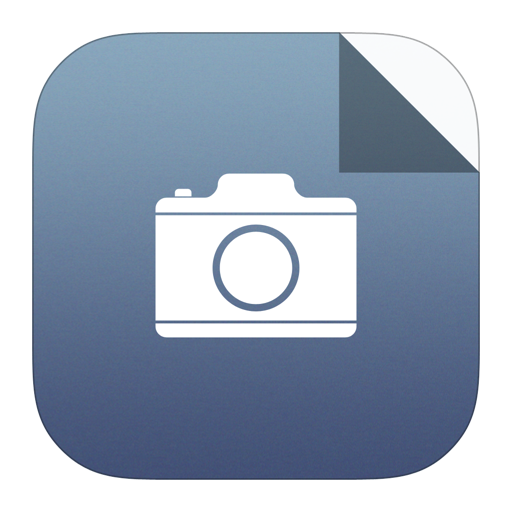
Larger image
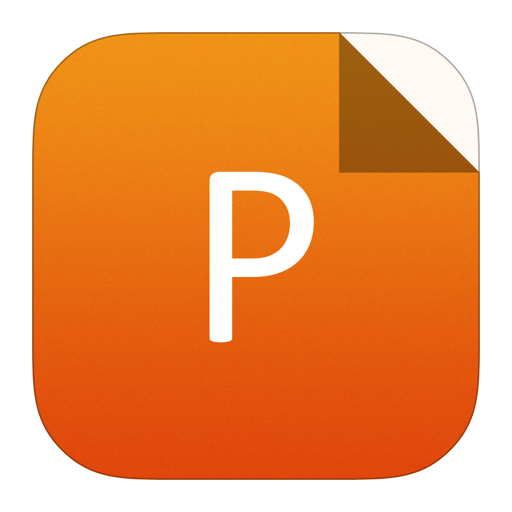
PowerPoint slide
Figure2.
(Color online) (a) Schematic diagram of field effect test device for zinc oxide fiber. (b) Field effect characteristics of Ce-doped ZnO[21]. (c) I–V curve of La-doped ZnO at different irradiation intensities. (d) Field effect characteristics of La-doped ZnO[19].

class="figure_img" id="Figure3"/>
Download
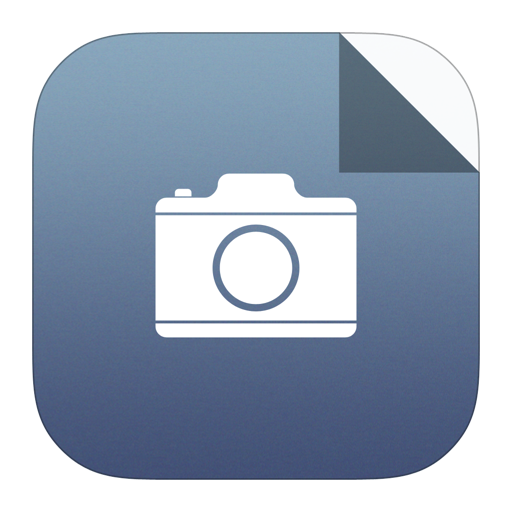
Larger image
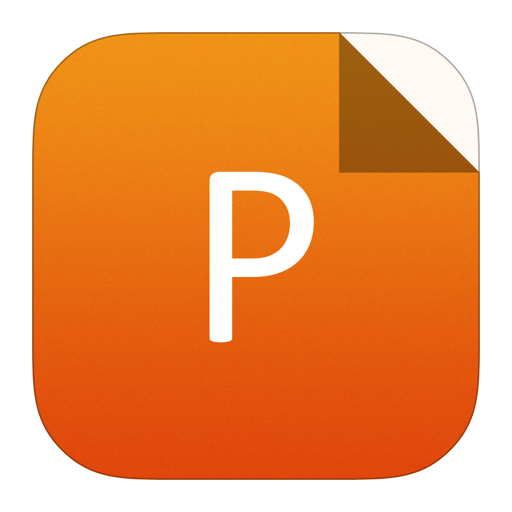
PowerPoint slide
Figure3.
(Color online) (a) Schematic of the printing unit. (b) Time-domain optical response of the ZnO fiber array. (c) I–V curve of the ZnO fiber array. (d) Flexible photodetector. (e) Optical photo of the nanowire array and silver electrode with a scale of 30 microns[22].
4.2
Stress sensor
The conductive fiber membrane prepared by electrospinning is easily changed under pressure for the variation of shape, and the electrical resistance of the fiber membrane is also changed. Therefore, the change in the current of the conductive fiber membrane can characterize the change in the corresponding stress[4, 23]. Tong et al.[24] provided a convenient method for making strain sensors. A thermoplastic polyurethane (TPU) nanofiber membrane was obtained by electrospinning, and polyaniline (PANI) was polymerized in situ on the fiber membrane to obtain a PANI/TPU sensor. The sensor has good response speed and stability and can detect small movements of the human body, as shown in Figs. 4(a) and 4(b)[24]. Yu et al.[25] prepared patterned nanofiber membranes for stress sensors, as shown in Figs. 4(c)–4(f) [25]. 2.2 g of polyvinylidene fluoride (PVDF) was dissolved in 3.9 g of DMF and 3.9 g of acetone mixed solution, and the solution was electrospun onto the patterned collector, followed by in situ polymerization on the patterned spun film. Polyaniline obtained a conductive polymer fiber membrane. The sample had a fast response time and good recovery for the press test, and the tensile properties were 2.6 times that of the corresponding nonwoven nanofiber film. The reason for this result is mainly related to the morphology of the fiber membrane.

class="figure_img" id="Figure4"/>
Download
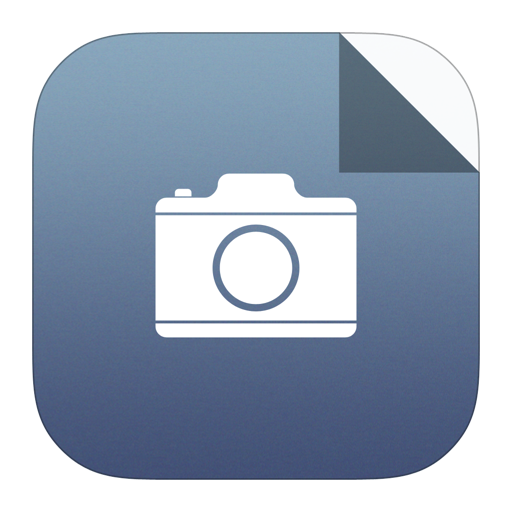
Larger image
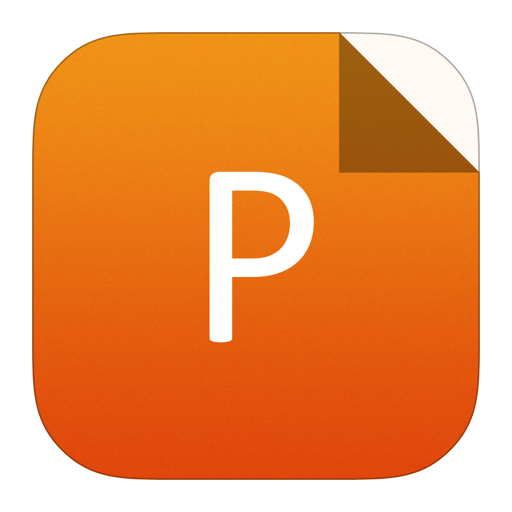
PowerPoint slide
Figure4.
(Color online) (a) I–V curves for PANI/TPU sensors under different tensions. (b) Responses when fingers are bent[24]. (c–f) Fibers with different patterned collectors[25].
A silver-loaded alginate stress sensor was prepared by Hu et al.[26]. A sodium alginate fiber membrane was obtained by electrospinning with 2 g of dimethyl sulfoxide, 36.8 g of water, 0.4 g of Triton X-100, 0.16 g of polyethylene oxide and 0.64 g of sodium alginate. The fiber membrane was completely wetted with a silver nitrate solution, and then dropped onto the fiber membrane with dimethylamine borane (DMAB). After a while, the silver-loaded alginate nanofiber membrane was obtained. The sample has certain antibacterial properties and good repeatability and durability, as shown in Fig. 5[26]. The sensor has good sensitivity to monitor human breathing and distinguish between different sounds. By creating a pressure distribution matrix, the sensor can detect the spatial pressure distribution[26].

class="figure_img" id="Figure5"/>
Download
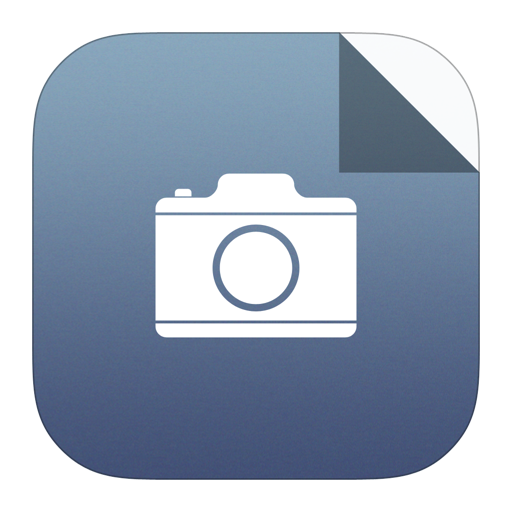
Larger image
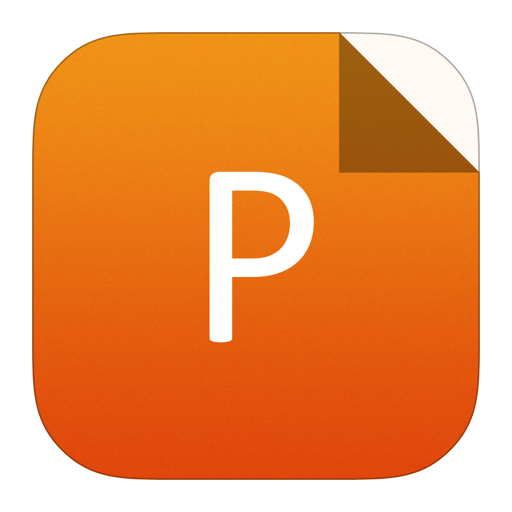
PowerPoint slide
Figure5.
(Color online) (a) Electrical performance of the sensor at different pressures. (b) Current change of the sensor at different pressures. (c) Response of the sensor to human respiration. (d) Response of the sensor to “Nano” and “Perfect”. (e) Electrode array diagram. (f) Pressure distribution[26].
4.3
Gas sensor
Due to its structural advantages, the large specific surface area and high porosity allow rapid gas detection, and the nano-conductive fiber membranes are well suited for use as gas sensors. Zinc oxide has a special shape and is widely used in various types of sensors. Zhang et al.[27] prepared comb-like ZnO nanofibers for gas sensors. Comb-shaped ZnO nanofibers were obtained by chemical vapor deposition using Au as a catalyst and zinc powder as a raw material. The sample showed a good response to CO at room temperature. Later, Zhang et al.[28] prepared a CO gas sensor again to improve the sensitivity of the sensor. PEDOT:PSS / PVP composite nanoparticles were prepared by electrospinning technique using poly(3,4-ethylenedioxythiophene): poly(styrene sulfonate) (PEDOT:PSS) and polyvinylpyrrolidone (PVP). The fiber exhibits good sensitivity to CO, as shown in Figs. 6(a) and 6(b)[28]. The ammonia gas sensor was also prepared by Zhang et al.[29]. Poly(methyl methacrylate) (PMMA) was dissolved in tetrahydrofuran (THF) to perform electrospinning to obtain a PMMA nanofiber membrane. Good ammonia gas sensitivity is obtained by in-situ polymerization of polyaniline on the fiber, as shown in Figs. 6(c) and 6(d)[29]. Humidity sensors were also prepared by electrospinning. The barium titanate (BaTiO3) fiber obtained by electrospinning and calcination of Sheng et al.[30] exhibits good humidity-sensitive properties, as shown in Figs. 6(e) and 6(f)[30].

class="figure_img" id="Figure6"/>
Download
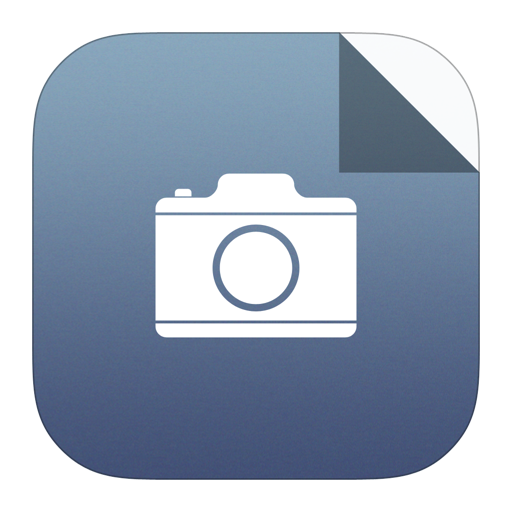
Larger image
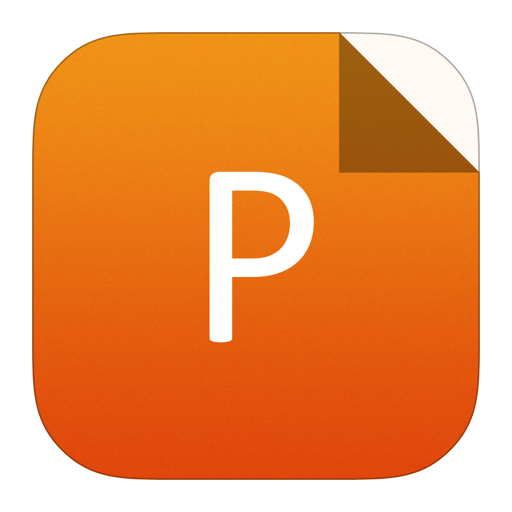
PowerPoint slide
Figure6.
(Color online) (a) PEDOT:PSS/PVP composite nanofibers response to CO. (b) PEDOT:PSS/PVP composite nanofibers response to different concentrations of CO[28]. (c) Polyaniline/PMMA nanofibers in response to different concentrations of ammonia. (d) Sensitivity of polyaniline/PMMA nanofibers[29]. (e) Humidity response of BaTiO3 fiber. (f) Recovery performance of BaTiO3 fiber[30].
The diameter of nanofibers affects their sensing properties. Zhang et al.[31] demonstrated that the decrease of nanofiber diameter can improve its sensing sensitivity by preparing ultrafine fibers. Polyvinyl alcohol (PVA), PEDOT: PSS as a precursor, a negative high-voltage power supply was added to increase the voltage of the electrospinning process, and the ultrafine fibers were successfully prepared by gas flow assistance. The fiber has an average diameter of 68 nm and is more sensitive to ammonia than conventional electrospun fibers, as shown in Fig. 7[31].

class="figure_img" id="Figure7"/>
Download
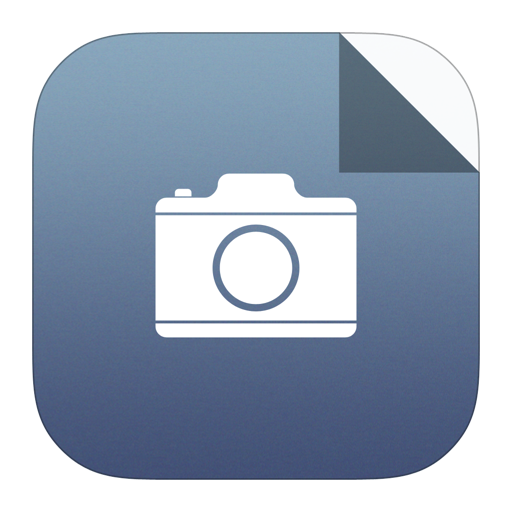
Larger image
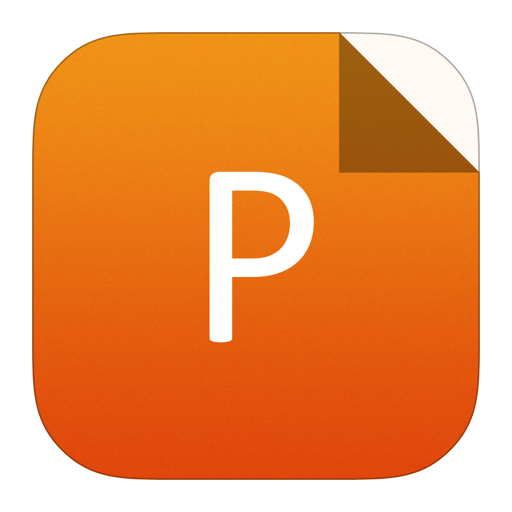
PowerPoint slide
Figure7.
(Color online) (a) SEM image of ultrafine fiber. (b) Particle size distribution of ultrafine fiber. (c, d) Ammonia response curve of traditional electrospun fiber. (e, f) Ammonia response curve of microfiber[31].
Zhang et al.[18] prepared Ag/alginate nanofibers by ion exchange between sodium ions in sodium alginate and silver ions in silver nitrate and in situ reduction. The nanofibers exhibit good humidity response characteristics at 20% ambient relative humidity to 85% ambient relative humidity. Place it on the mask to monitor the depth and frequency of breathing, and even to distinguish between breathing in joy and sadness, as shown in Figs. 8(a)–8(d)[18]. This will play a big role in the direction of the interactive system. The sensor monitors breathing during sleep and clearly distinguishes between normal (NB) and hard to breathe (HB) states, providing an alarm and timely assistance during apnea, as shown in Figs. 8(e) and 8(f)[18].

class="figure_img" id="Figure8"/>
Download
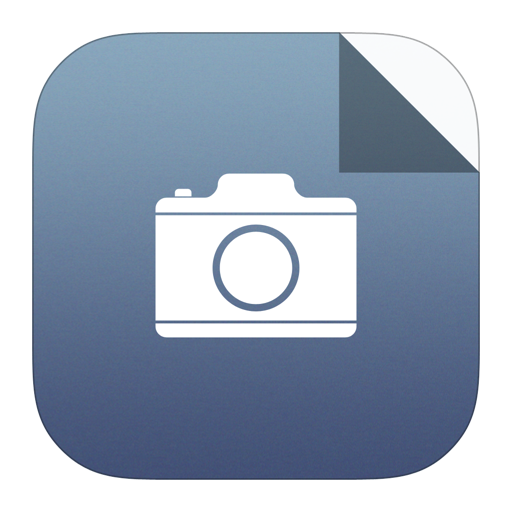
Larger image
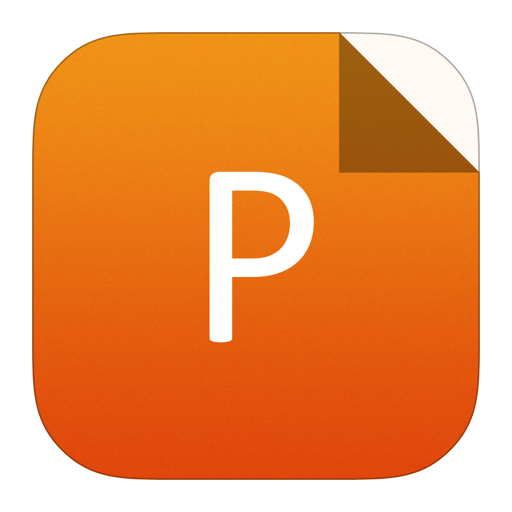
PowerPoint slide
Figure8.
(Color online) (a) Photo of the mask combined with the sensor. (b) Response in normal and running state. (c) Response in joy state. (d) Response in sad state. (e) Response in sleep state. (f) Response in normal breathing (NB) and hard to breathe (HB) conditions[18].
4.4
Nanogenerator
Nano-generators can convert small-scale environmental energy into electrical energy, and self-powered systems based on nano-generators will be a good choice for reducing the size of electronic devices. On the other hand, the strength of the output signal of the nanogenerator is related to pressure and temperature, and can be used as a self-powered sensor. Due to its portability and self-powered features, there are many applications in the field of wearable devices. You et al.[5] prepared a hybrid nano-generator based on electrospun polyvinylidene fluoride (PVDF). The nanogenerator has both piezoelectric and pyroelectric output characteristics, and outputs a corresponding signal when the temperature and pressure changes. Moreover, the piezoelectric and pyroelectric signals do not affect each other, but the superimposed output, as shown in Fig. 9[5]. Wang et al.[32] adopted a single-electrode configuration to further increase the portability of the piezoelectric system and prepared a single-electrode electronic skin based on PVDF nanogenerator. It has not only piezoelectric and pyroelectric properties but also flexibility and transparency, as shown in Fig. 10. And this electronic skin is more stable and has good short circuit resistance, as shown in Fig. 11[32].

class="figure_img" id="Figure9"/>
Download
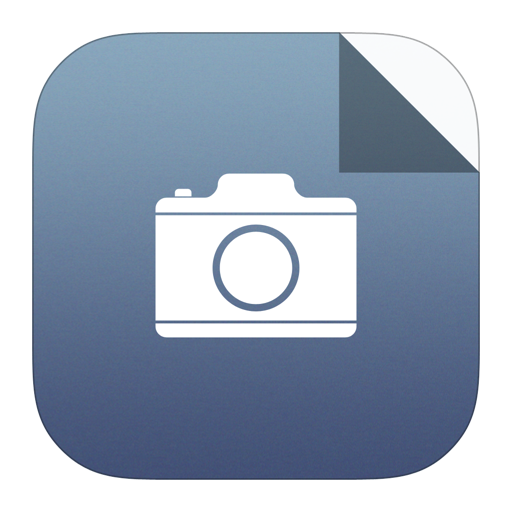
Larger image
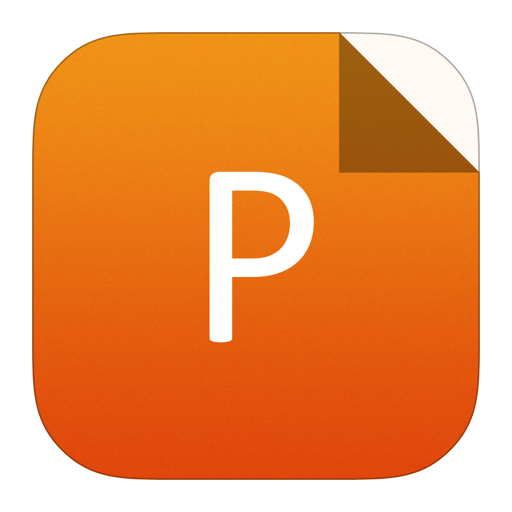
PowerPoint slide
Figure9.
(Color online) (a) Piezoelectric properties of PVDF under pressure. (b) Piezoelectric properties of PVDF during bending. (c) Pyroelectric characteristics of PVDF. (d) Mixed output of piezoelectric signals and pyroelectric signals of PVDF[5].

class="figure_img" id="Figure10"/>
Download
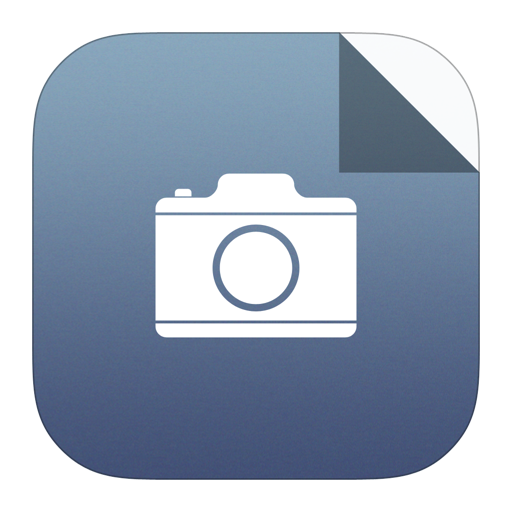
Larger image
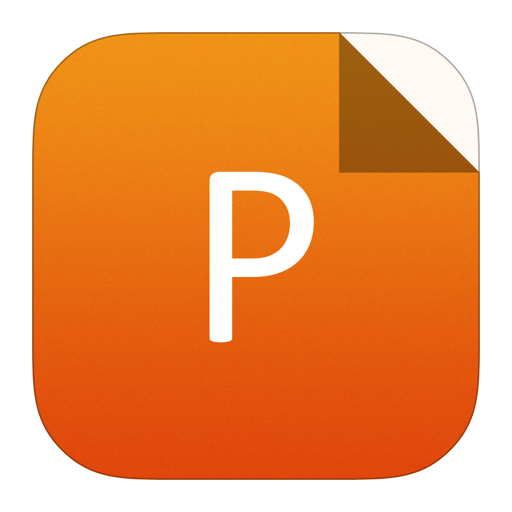
PowerPoint slide
Figure10.
(Color online) (a) Electronic skin map and wiring diagram. (b) Piezoelectric properties of electronic skin under pressure. (c) Piezoelectric properties of electronic skin during bending. (d) Pyroelectric characteristics of electronic skin[32].

class="figure_img" id="Figure11"/>
Download
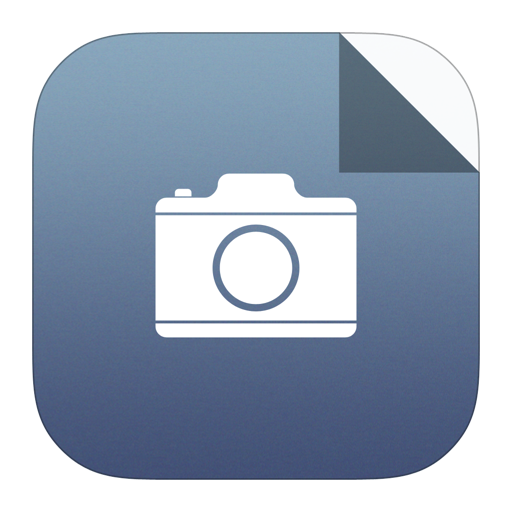
Larger image
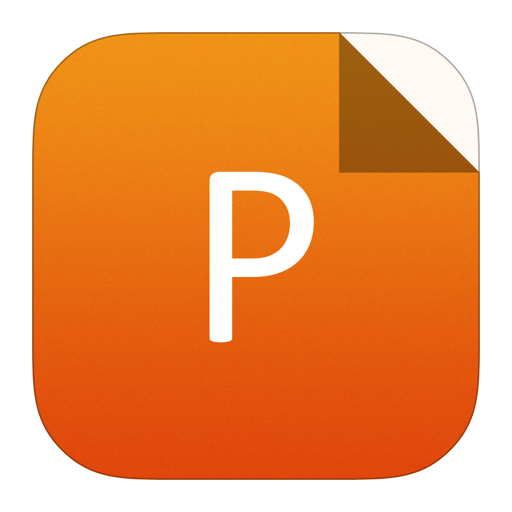
PowerPoint slide
Figure11.
(Color online) (a) Pressure profile. (b) Temperature profile. (c) Output of electronic skin after short-circuit of adjacent components. (d) Output signal of direct short-circuit of resistor unit. (e) Output of resistor unit in short-circuit condition of adjacent components[32].
Guo et al.[33] added barium titanate (BaTiO3) to PVDF to improve the piezoelectric properties of PVDF. The PVDF/BaTiO3 nanofiber membrane was obtained by electrospinning BaTiO3 into a PVDF precursor solution. A wireless piezoelectric device was fabricated by combining a fiber membrane with a Bluetooth transmission device. The device has good sensitivity and can be used for human motion monitoring, as shown in Fig. 12[33]. Triboelectric nanogenerators (TENG) are also capable of converting external energy into electrical energy. Qiu et al.[34] prepared a highly sensitive triboelectric nanogenerator using PVDF and PA6 as functional layers to form an all-fabric TENG. It has an open circuit voltage of up to 1000 V and is not subject to interference from additional resistors. It monitors the patient's breathing and can transmit information through a finger click, as shown in Fig. 13[34].

class="figure_img" id="Figure12"/>
Download
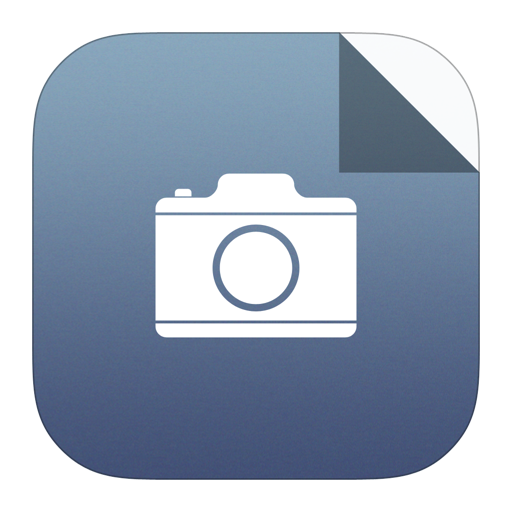
Larger image
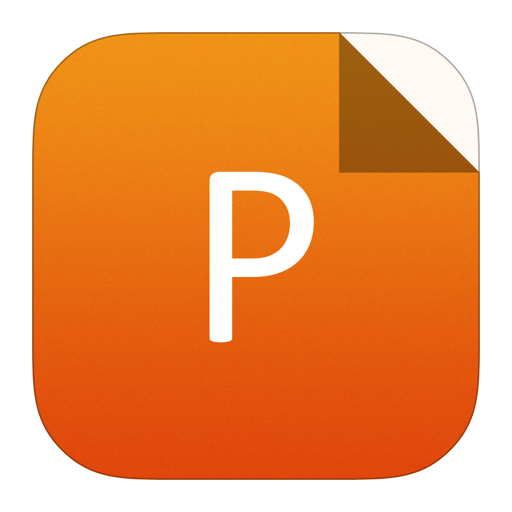
PowerPoint slide
Figure12.
(Color online) (a) Bluetooth device schematic. (b) Software interface diagram. (c) Wireless signal when squatting. (d) Wireless signal while walking. (e) Wireless signal during running. (f) Effect of BaTiO3 content on piezoelectricity. (g) 3% BaTiO3 The piezoelectricity of the PVDF fiber membrane at different frequency impacts[33].

class="figure_img" id="Figure13"/>
Download
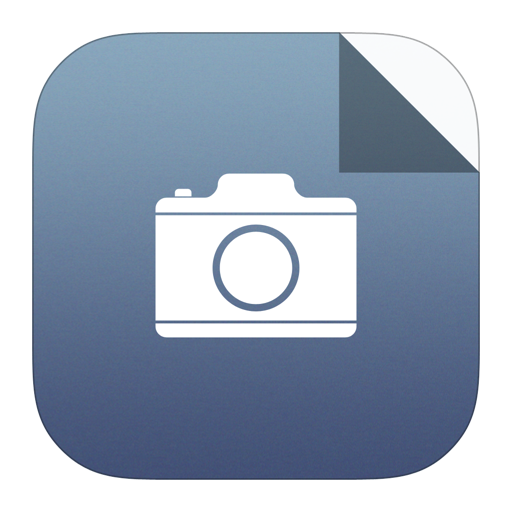
Larger image
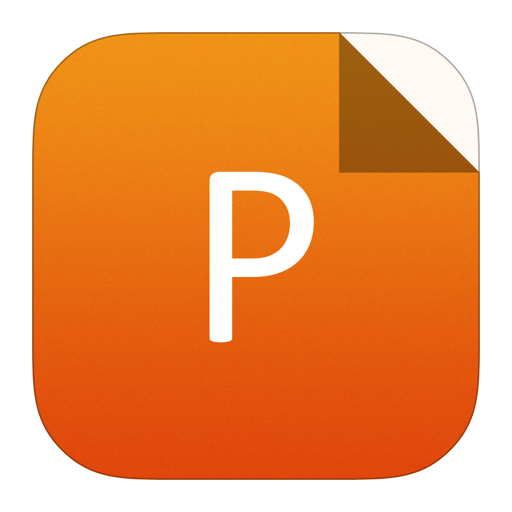
PowerPoint slide
Figure13.
(Color online) (a) Triboelectric nanogenerator structure diagram. (b) Open circuit voltage output. (c) Breathing output signal. (d) Finger click output signal[34].
5.
Summary
Flexible sensors composed of conductive nanofibers exhibit outstanding performance. The large-scale and low-cost development of high-performance conductive nanomaterials will greatly promote the development of flexible sensors. Of course, electrospinning technology is an alternative solution. Electrospinning technology can control the fiber diameter and even produce fibers with a diameter of less than 1 nm[35–37]. However, fine control of fiber diameter and preparation of fine nanofibers having a uniform diameter are still challenging. In order to further improve the electrical conductivity of the electrospun fiber, the combination of the electrospinning technology and other conductor preparation methods will have a better development prospect. Flexible sensors have been applied to many areas of electronic devices, with the health care field being the most compelling. This wearable sensor for health and motion monitoring will improve people's lifestyles. In addition, with the development of Internet of Things technology, the smart home field has also shown good development prospects.
Acknowledgements
This work was supported by the National Natural Science Foundation of China (Nos. 51673103 and 51973100).