Why need precision photonic integration? Of the issues indicated above, another critical issue is how to increase the integration density at a very low cost. For dense WDM (DWDM), the standard wavelength spacing is 100 GHz or ~ 0.8 nm around 1550 nm. In order to produce the 0.8 nm wavelength difference, the structural variation in grating pitch or ring radius etc. could be as small as ~ 0.13 nm, which is near the size of a hydrogen atom. In future LS-PICs, there may be many wavelength sensitive components and their adjustment is realized by the change of the temperature. Because the maximum wavelength deviation may be as large as several nanometers, the maximum temperature change may be several tens of degrees, which will be leading a very low yield in LS-PICs. Therefore, precision wavelength control will be necessary for general LS-PICs. For example, multi-wavelength laser arrays (MLAs) with a high channel count are considered to be an engine of LS-PICs, but high-volume production of MLAs with accurate wavelength control and low manufacturing cost still remains a huge challenge. Currently, the distributed feedback (DFB) lasers used in MLAs are fabricated using electron beam lithography (EBL), which offers from high resolution fabrication but low throughput because of the long writing time[3]. It is also well-known that EBL suffers from drawbacks such as blanking or deflection errors and shaping errors. Very few references have discussed the non-uniformity of the wavelength spacing of the devices fabricated using EBL. In Ref. [4], it is shown that using EBL, only 35% lasers have a wavelength variation of less than ± 0.2 nm. Ref. [5] shows that the error associated with the EBL process may be as large as 3 nm. In Ref. [6], when the difference between the two rings is only about 0.4 nm, the optical response is completely different. This shows that although the optical components are much larger than transistors in ICs, the higher process precision control is necessary for PICs. We can call this as precision photonic integrated circuits (precision PICs). Precision PICs means the resonance wavelength (channel) spacing will be controlled precisely.
What is reconstruction-equivalent-chirp (REC) technique for semiconductor laser and laser array? REC technique, which is first proposed by us, is a simple technique that only sampled Bragg grating (SBG) is used to design and fabricate lasers and laser arrays with complex grating structures. Through changing the sampling pattern, equivalent chirp (continuous change) or equivalent shift (discrete change) of the sub-gratings (Fourier components) in the SBG can be produced. The Bragg wavelengths of the sub-gratings can also be changed with the sampling period. Thus various sub-gratings with complex structures can be obtained by pre-designing the sampling pattern. Sampling period is usually several micrometers, which can be formed by using normal photolithography. Combined the reconstruction algorithm for desired optical response, the arbitrary grating profile (chirp/ shift) can be equivalently obtained by sampled grating with μm-level photolithography. That is why such a technique is called REC technique. The REC technique and its applications in semiconductor lasers have been illustrated in a number of papers[7–9] . The most important advantage of REC technique is its precision in wavelength control. Ref. [9] shows the error tolerance of the wavelength precision is largely relaxed by at least 100 times.
In Ref. [10], 60-wavelength DFB laser arrays with a π-equivalent phase shift (π-EPS) were designed and fabricated in two wafers. For all the laser arrays, the mean lasing wavelength residuals of 83.3% of the lasers are within ± 0.20 nm, and 93.5% are within ± 0.30 nm. Lasers with a wavelength deviation of > 0.50 nm are less than 1.0% of the total laser count. The single-longitudinal-mode (SLM) yield of lasers from the seven arrays are 98.3%, 100%, 100%, 100%, 98.3%, 93.3% and 100% respectively.
As shown in Fig. 1, the standarddeviationis 0.159 nm, which means 68.26% of the laser wavelengths are lied within ± 0.159 nm. The detailed lasing spectrum of one array was randomly selected. Its wavelength spacing is 0.8 nm.

class="figure_img" id="Figure1"/>
Download
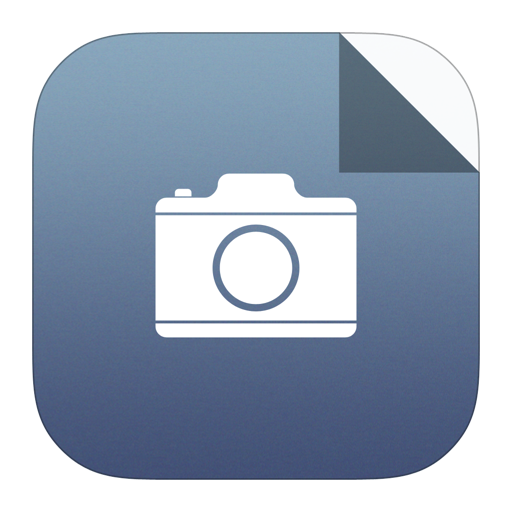
Larger image
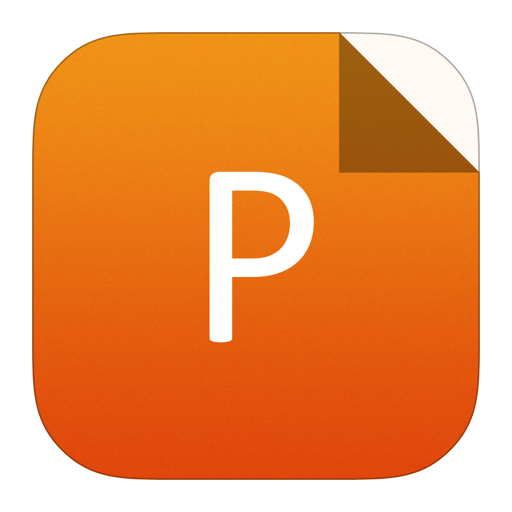
PowerPoint slide
Figure1.
(Color online) (a) Frequency count of wavelength residual (871 lasers) and (b) measured lasing spectra of one 60-channel array.
From the above experimental results, it can be seen that low-cost high-channel-count and high precision channel spacing MLAs can be obtained by REC technique. It also implies that the two great obstacles to manufacture MLAs for very-large-scale PICs, namely poor wavelength accuracy and the low yield, which have impeded progress for nearly three decades, have been essentially overcome.
Typical applications? Fast tunable lasers with switching time less than one microsecond or even tens of nanoseconds (ultra-fast) are key components in high-speed smart networks or software defined networks (SDNs). The wavelength of the Vernier effect based tunable lasers can in principle be switched from one wavelength to another by tuning the currents. However, it comes with the change in heat dissipation and the thermal relaxation takes quite some time. Moreover, the thermal settling may cause mode hoping, which implies large deviations from the target wavelength. Thus Vernier effect based tunable lasers should be very difficult to be commercialized as fast tunable lasers. The other tunable laser with fast tuning speed is demonstrated by the tunable distributed amplification distributed feedback (TDA-DFB) principle[11], which is needed to examine in actual applications. It should be mentioned that the fabrication of TDA-DFB lasers is much more complex than Vernier effect based tunable lasers and the yield may be a problem, thus making the module very expensive.
An effective and low-cost method is to integrate multi-wavelength laser array (MLA) and switch between wavelengths by turning on/off individual lasers. The DFB lasers are very stable against temperature variations and the speed of electronics is only a few nanoseconds, making it possible to stabilize the wavelength within tens of nanoseconds. The proposed method is an M × N DFB laser matrix shown in Fig. 2. It can cover wide tuning range and the wavelength can be switched quickly by turning on/off individual lasers using high speed switching driving circuit. Because for a wideband fast tunable laser, there are at least 40-wavlength array, the precision PICs will be very important.

class="figure_img" id="Figure2"/>
Download
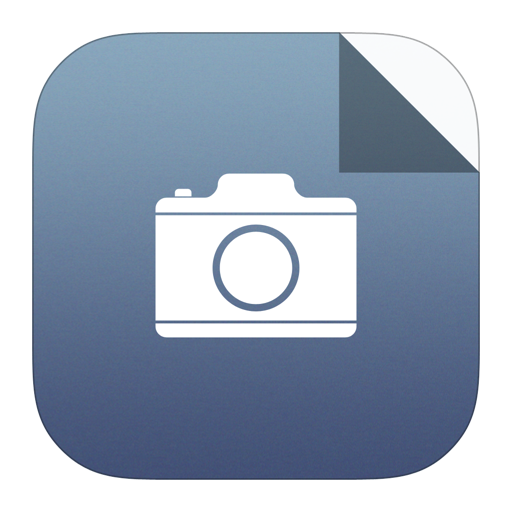
Larger image
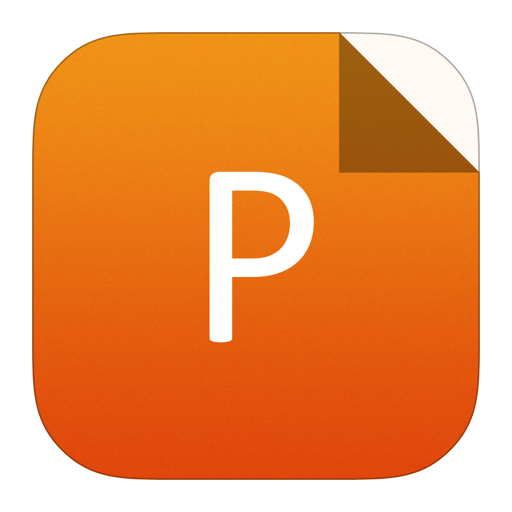
PowerPoint slide
Figure2.
(Color online) Schematic of an M × N DFB laser matrix with high speed switching driver circuit.
Possible way for LS-PICs based on precision PICs and photonic wire bonding?
Because up to now, both the semiconductor processes and the silicon lasers are very difficult for commercial LS-PICs, another possible way should be pursued, Photonic wire bonding (PWB) is a technique developed at the Karlsruhe Institute of Technology (KIT) of Germany in which photonic waveguides are written with an ultrafast laser into a photoresist material via two-photon lithography[12].
The combination of LS precision REC laser arrays and PWB may is a good way to LS-PICs, as Fig. 3 shows. The modulators and passive waveguides can be made based on silicon photonics.

class="figure_img" id="Figure3"/>
Download
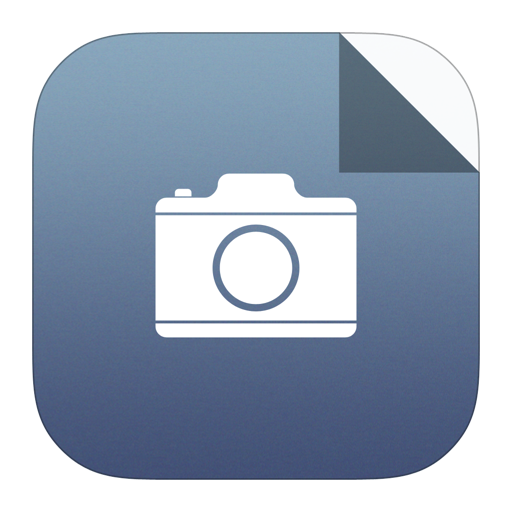
Larger image
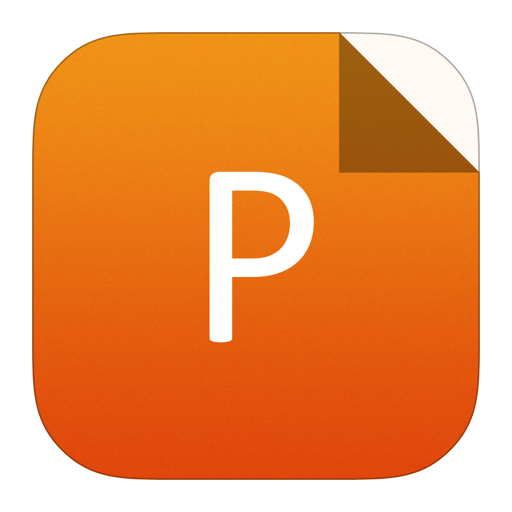
PowerPoint slide
Figure3.
(Color online) Schematic of an LS-PICs based on the combination of LS precision REC laser arrays and PWB.
Ruiqin Jin
Ruiqin Jin is a senior editor of Journal of Semiconductors.