1.
Introduction
Electrically-pumped lasers on silicon are in great demand for the recent developments of silicon-based photonics integrations[1, 2]. However, silicon-based materials with an indirect band-gap are not suitable for realizing low-threshold light sources because of their ultralow light-emission efficiency[3–5]. Thus, heterogeneous integrations of direct-band-gap III–V semiconductor materials on silicon or germanium substrates through heteroepitaxy techniques[6, 7] and bonding techniques[8, 9] have been attracting a great deal of attention, for the potential benefits of utilizing both the high light-emission efficiency of the III–V semiconductor materials and the great integration compatibility of silicon. Notably, hybrid silicon evanescent lasers coupled to a silicon waveguide were demonstrated through the direct molecular bonding[10–13], the benzocyclobutene (BCB) adhesive bonding[14–17], and the selective-area metal bonding technologies[18].
The distributed Bragg reflector[19] and distributed feedback[20] structures with high facet reflectivity were typically used to realize single-mode hybrid silicon lasers with short cavities. Previously, we found a metal-coated layer can enhance the mode quality (Q) factors of the Fabry–Pérot(FP)-type modes in square and circular microcavities[21, 22], and improve the thermal conductivity of hybrid III–V/Si lasers[23]. In this paper, we propose and demonstrate metal-confined AlGaInAs/InP FP lasers BCB adhesively bonded on the fabricated silicon-on-insulator (SOI) waveguide. The etched sidewalls and the end facets of the AlGaInAs/InP FP cavity are covered by a thin SiO2 layer and a p-electrode metal layer, which allows strong optical confinement in the gain material with near-total facet reflection, improves the thermal properties of the hybrid laser, and compels the laser emission to the underneath silicon waveguide through evanescent-field coupling. For the 415 μm-long, 7 μm-wide hybrid AlGaInAs/Si FP laser, we obtain a continuous-wave (CW) lasing threshold current of 45 mA at a heat-sink temperature of 285 K. In addition, single-mode lasing is achieved with a threshold current of 16 mA and a side-mode-suppression ratio (SMSR) of 29 dB for the 150 μm-long, 6 μm-wide hybrid FP laser.
2.
Numerical simulations of hybrid FP cavity
Fig. 1(a) shows a schematic diagram of a hybrid AlGaInAs/Si FP laser. The silicon waveguide is fabricated on the SOI wafer and the BCB adhesive bonding technology is used to bond the AlGaInAs/InP multiple quantum wells (MQWs) die onto the SOI wafer. The facets of the AlGaInAs/InP FP cavity are covered with the p-electrode metal for enhancing the mode confinement after cavity fabrication, and a thin InP contacting layer is left for current injection. Fig. 1(b) shows the cross-sectional view of the hybrid FP cavity. The mode characteristics of the transverse electric (TE) modes are investigated by the three-dimensional (3D) finite-difference time-domain (FDTD) method for a small-size hybrid FP cavity with a length of 4 μm and a width of 1 μm. The simulated cavity size is relatively small, because of the limitation of our computation power. The thicknesses of the InP upper-cladding and lower-cladding layers, the AlGaInAs MQWs layer, the separate-confinement heterostructure (SCH) layer, the BCB bonding layer, and the silicon waveguide are 1.365, 0.35, 0.09, 0.125, 0.08 and 0.34 μm, respectively, following the parameters used in the experiment. The corresponding refractive indices of the InP cladding layer, the SCH layer, the MQWs layer, the BCB bonding layer, and the silicon waveguide are 3.17, 3.4, 3.47, 1.54, and 3.48, respectively. The thicknesses of the SiO2 layer and the p-electrode gold metal layer are 200 nm and 100 nm, with the refractive indices of 1.45 and 0.574 + i9.66, respectively. The external region of the FP cavity is removed except for a thin n-InP bottom contacting layer with a thickness of t. We calculate the intensity spectra by the 3D FDTD simulation and the Padé approximation[23] and plot them in Fig. 2. The spectra show a clear longitudinal mode structure for the metal-coated hybrid laser cavity with t = 100 and 200 nm, and we cannot obtain an accurate spectrum for the cavity without metal coating because of the high modal loss. The mode wavelengths and Q factors can be obtained by fitting the resonance peaks in the intensity spectra with a Lorentzian function. The mode Q factor of mode A in Fig. 2 is 360 and 210 for the hybrid FP cavity confined by a p-electrode metal layer with t = 100 and 200 nm, respectively.

class="figure_img" id="Figure1"/>
Download
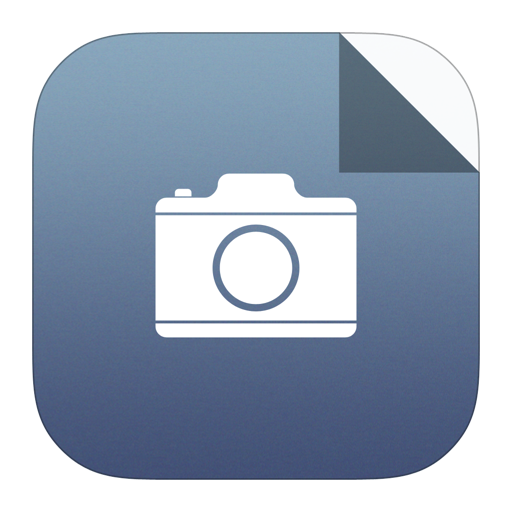
Larger image
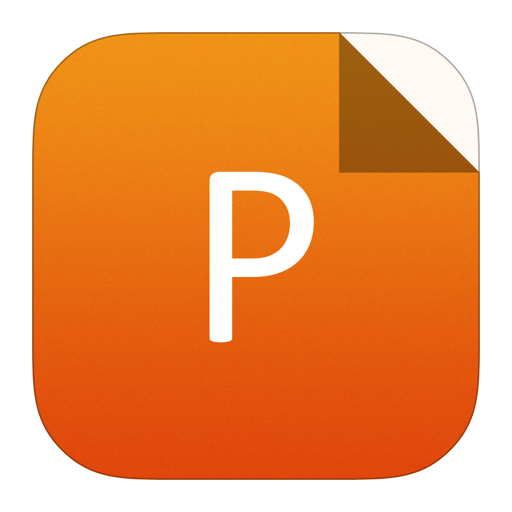
PowerPoint slide
Figure1.
(Color online) (a) 3-D schematic diagram and (b) cross-sectional view of the hybrid AlGaInAs/Si FP laser.

class="figure_img" id="Figure2"/>
Download
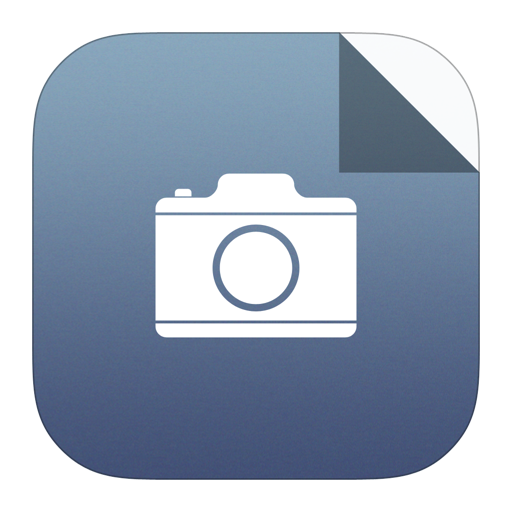
Larger image
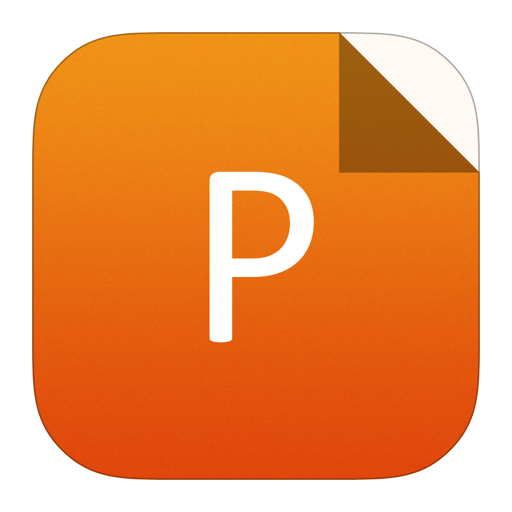
PowerPoint slide
Figure2.
(Color online) Simulated intensity spectra of the TE modes in the hybrid FP cavity with a width of 1 μm and a length of 4 μm. Solid line: t = 100 nm. Dashed line: t = 200 nm. A: resonance at 1487 nm. B: resonance at 1503 nm.
In order to illustrate the influence of the coated p-electrode metal on the mode confinement, we estimate the mode Q factor of the FP cavity from
$$Q = - {n_{ m{g}}}{k_0}L/ln R,$$ ![]() | (1) |
where ng, k0, L, and R are the group refractive index, the propagation constant in a vacuum, the cavity length, and the reflectivity at the FP cavity facet, respectively. The simulated group refractive index ng of the 1 μm-wide FP cavity is about 3.7 at ~1.5 μm considering the structure dispersion. The reflectivity R is about 0.27 and 0.93 at the InP-air and InP-Au boundaries with the refractive index contrasts of 3.17/1 and 3.17/0.574 + i9.66, respectively (given by Fresnel reflection at normal incidence). The Q factor is then obtained as ~50 and ~850 from Eq. (1), which show more than one order enhancement of the Q factor for the hybrid FP laser confined by the p-electrode metal.
The mode-field patterns can be simulated under a narrowband source centered at the resonant wavelength of the desired mode in the FDTD simulation. For mode A at 1487 nm in Fig. 2, the mode-field distributions of the magnetic field component in the y-direction (Hy) are obtained by the FDTD simulation. Figs. 3(a), 3(b), and 3(c) show the Hy distribution in the x–y plane at y = 0.1 μm, the x–y planes at z = 0.1 μm, and z = 8 μm, respectively. Here, the bottom contacting layer thickness t is 100 nm. The simulated mode-field pattern reveals the fundamental mode distribution in the y-direction, with the longitudinal mode number of 18 neglecting the field in the SiO2 layer. Furthermore, we find that the minor peak marked by B at 1503 nm in Fig. 2 is the second-order transverse mode in the y-direction, with the longitudinal mode number of 16, which has weak optical confinement in MQWs and a low Q factor.

class="figure_img" id="Figure3"/>
Download
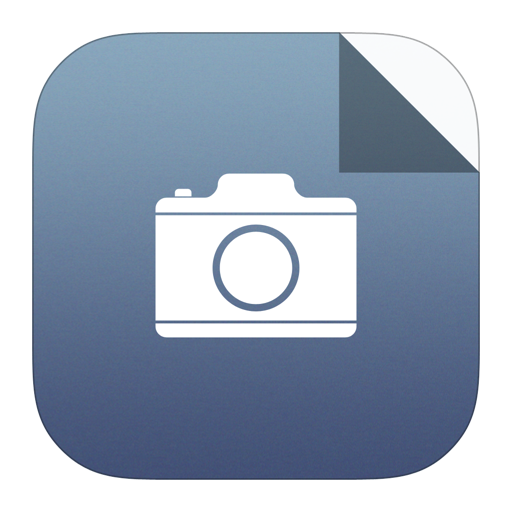
Larger image
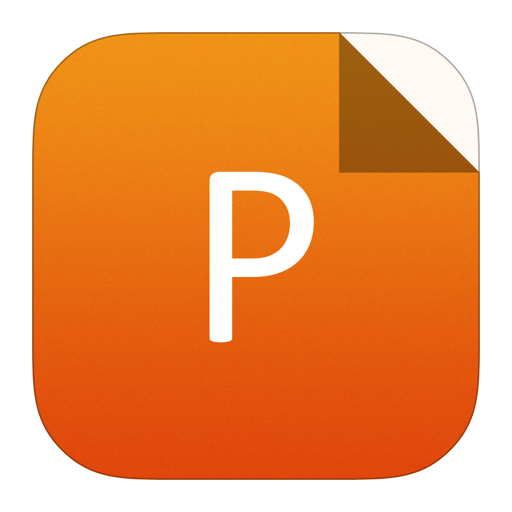
PowerPoint slide
Figure3.
(Color online) Simulated magnetic-field Hy distributions in (a) the x–z plane at y = 0.1 μm, the x–y planes at (b) z = 0.1 μm, and (c) z = 8 μm for the mode A at 1487 nm, in the hybrid FP cavity with t = 100 nm.
The mode Q factor obtained by the FDTD simulation Qt is decomposed into the radiation Q factor (Qr), and the metal absorption Q factor (Qm) with the relation of Qt?1 = Qr?1 + Qm?1. Based on the simulated mode field distribution, Qr is calculated by
$${Q_{ m{r}}} = 2pi fW/{P_{ m{r}}},$$ ![]() | (2) |
where W is the total energy stored in the cavity, f is the mode frequency, and Pr is the total radiant power calculated by integrating the power flux density at all the surfaces surrounding the metal-confined FP cavity. The waveguide coupling efficiency ηc is defined as
$${eta _{ m{c}}} = {P_{ m{w}}}/{P_{ m{r}}},$$ ![]() | (3) |
where Pw is the radiant power confined in the Si waveguide. Thus the output extraction efficiency ηe can be calculated from
$${eta _{ m{e}}} = {eta _{ m{c}}}{Q_{ m{t}}}/{Q_{ m{r}}}.$$ ![]() | (4) |
The simulated mode Q factor Qt, radiation Q factor Qr, waveguide coupling efficiency ηc, and the output extraction efficiency ηe are summarized in Table 1 at t = 50, 100, 150, and 200 nm. The results show that the output extraction efficiency ηe can be several tens percent in the consideration of the metal absorption, while the Q factors are greatly enhanced compared with the FP cavity confined by air. The mode-field distribution in the bottom InP contacting layer cannot be confined by the p-electrode, so Qt and Qr decrease with the increase of
t (nm) | Qt | Qr | ηc (%) | ηe (%) |
50 | 570 | 1800 | 93 | 30 |
100 | 360 | 630 | 67 | 39 |
150 | 260 | 340 | 39 | 31 |
200 | 210 | 270 | 38 | 29 |
Table1.
Simulated mode Q factor Qt, radiation Q factor Qr, waveguide coupling efficiency ηc, and output extraction efficiency ηe versus the thickness t for mode A.
Table options
-->

Download as CSV
t (nm) | Qt | Qr | ηc (%) | ηe (%) |
50 | 570 | 1800 | 93 | 30 |
100 | 360 | 630 | 67 | 39 |
150 | 260 | 340 | 39 | 31 |
200 | 210 | 270 | 38 | 29 |
Hence, the simulation results indicate that the hybrid FP cavity encased by the metal layer can enhance the mode confinement and Q factor, compel efficient lasing emission from the underneath silicon waveguide, and maintain a high optical confinement in the active region. The mode Q factor for the 15 μm-long hybrid FP cavity with the bottom contacting layer thickness of 100 nm can be estimated as ~1400 from Table 1 assuming the same coupling and facet loss. Thus, a hybrid FP laser with a cavity length of 15 μm can be expected with a modal gain of ~100 cm?1. More detailed analysis of the mode characteristics is needed for optimizing such hybrid silicon FP lasers. Indeed, the thicknesses of the InP cladding layer, the SiO2 isolating layer, and the bottom InP contacting layer can be adjusted to further optimize the output extraction efficiency and the mode confinement.
3.
Hybrid FP laser confined by the metal layer
The hybrid FP lasers are fabricated using similar processes as in Ref. [14]. Differently, a p-electrode Ti–Pt–Au layer is deposited to cover the sidewalls and the facets of the AlGaInAs FP cavity. Fig. 4(a) shows the cross-sectional view scanning-electron microscope (SEM) image of the AlGaInAs/Si FP cavity. Fig. 4(b) shows the top-view SEM image of the dry-etched facet of the AlGaInAs FP cavity, which is covered by the insulating SiO2 layer and the p-electrode layer.

class="figure_img" id="Figure4"/>
Download
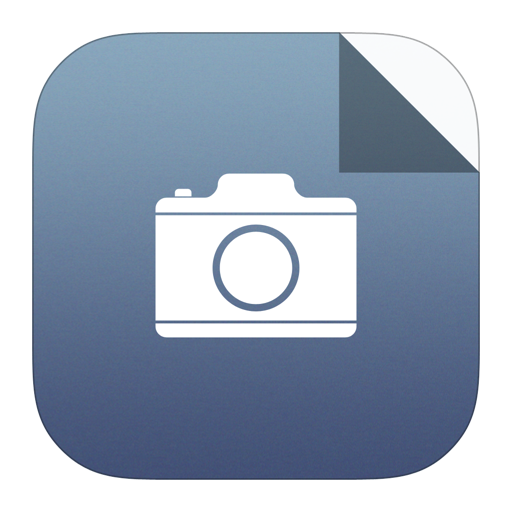
Larger image
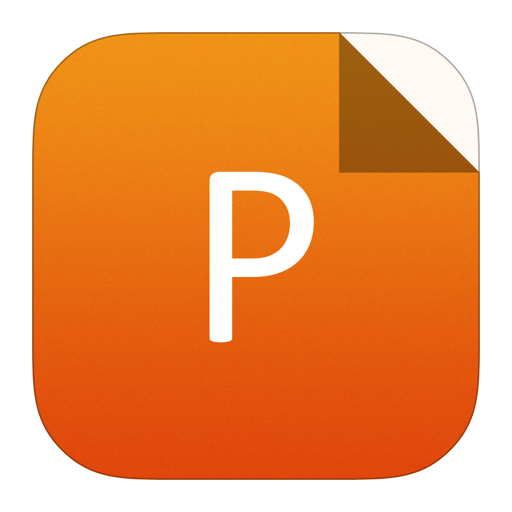
PowerPoint slide
Figure4.
(a) Cross-sectional-view SEM image of the AlGaInAs/Si FP laser covered by the SiO2 and p-electrode metal layers with the evanescently coupled silicon waveguide underneath. (b) Top-view SEM image of the p-electrode metal coated FP laser.
After cleaving the silicon waveguide, we characterize the hybrid FP lasers with a thermoelectric cooler (TEC) to control the substrate temperature. For a 415 μm-long, 7 μm-wide hybrid AlGaInAs/Si FP laser with p-electrode coated on the facets, the output powers versus CW injection currents are measured and plotted in Fig. 5(a) at the TEC temperatures of 281, 285, and 293 K. A series resistance of 13.1 is estimated from the voltage–current (V–I) curve around the threshold. The CW threshold currents are about 40 and 45 mA at 281 and 285 K, respectively, which correspond to the current densities of 1.38 and 1.55 kA/cm2. The output power increases to about 120 μW around the injection current of 73.5 mA and then experiences a kink, where the slope efficiency decreases from 4.0 to 2.0 mW/A, as shown in Fig. 5(a). CW injection lasing is not realized for the hybrid FP laser without metal coating on the facet. As a comparison, Fig. 5(b) shows the peak powers versus the pulsed current with a repetition rate of 1 MHz and a pulse width of 100 ns at 285 K for both lasers. The threshold currents are 36 and 45 mA, the peak powers are 1.15 and 0.55 mW at the current of 120 mA, and the slope efficiencies are 15.1 and 7.4 mW/A for the hybrid FP laser with and without metal coating, respectively. Therefore, low threshold operation and efficient output to the silicon waveguide are demonstrated with the p-electrode metal coating, which is in accordance with the simulation results.

class="figure_img" id="Figure5"/>
Download
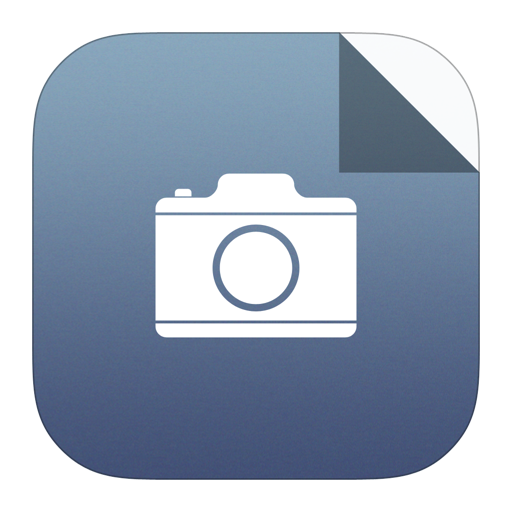
Larger image
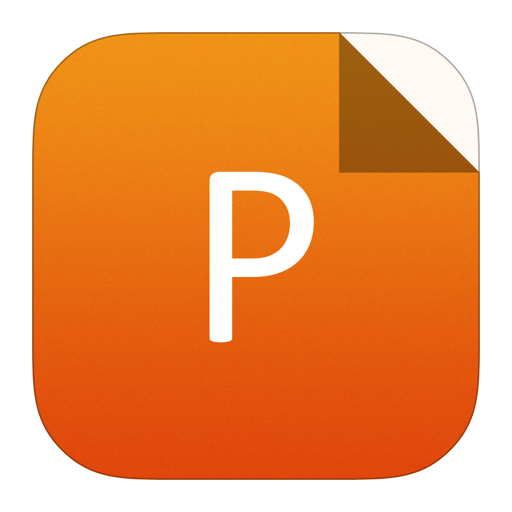
PowerPoint slide
Figure5.
(Color online) (a) Output powers from and applied voltage versus CW injection current for the metal-coated hybrid FP laser. (b) Output peak power versus pulsed injection currents for the hybrid FP lasers with and without p-electrode metal on facets.
The lasing spectra of the metal-coated hybrid FP laser are measured by the optical spectrum analyzer (OSA) with a resolution of 0.067 nm at 285 K. The measured lasing spectra at the CW injection currents of 50 and 60 mA are plotted in Figs. 6(a) and 6(b), respectively. Two sets of longitudinal modes are observed, with the minor set marked by the arrows in Fig. 6. The main lasing mode jumps over 7 nm by 9 longitudinal modes when the CW current increases from 50 mA to 60 mA, and an additional thermal-induced wavelength redshift of about 0.6 nm is also observed. The longitudinal mode interval is 0.78 nm for the modes around 1548 nm, which corresponds to a group refractive index of 3.7 with the cavity length of 415 μm.

class="figure_img" id="Figure6"/>
Download
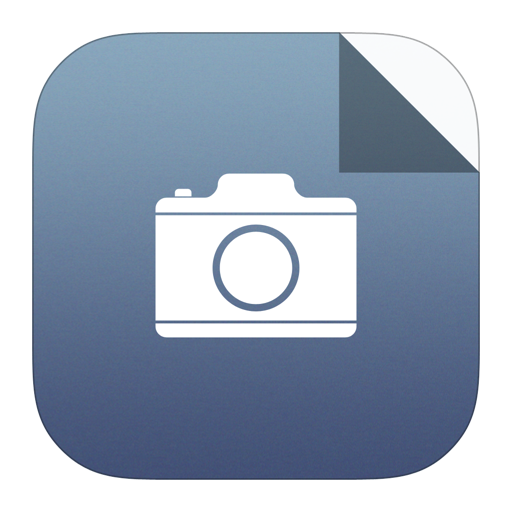
Larger image
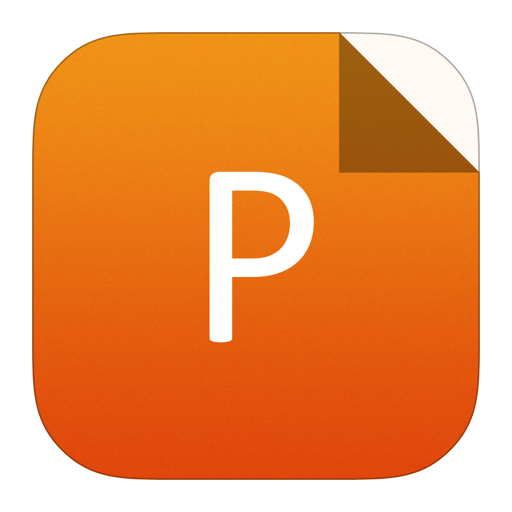
PowerPoint slide
Figure6.
(Color online) Lasing spectra of the metal-coated hybrid FP laser measured at the CW injection currents of (a) 50 mA and (b) 60 mA.
The optical microscope images for the near-field emission pattern of the silicon waveguide end-face and the top-view of the elastic scattering light are shown in Figs. 7(a) and 7(b), respectively, for the hybrid FP laser at a pulsed injection current of 40 mA. The near-field emission pattern indicates the output power is mainly confined in the silicon waveguide. The scattering light is clearly observed at the cleaved silicon waveguide end face, which is about 50 μm away from the end face of the FP cavity. Both of these images reveal efficient lasing output from the silicon waveguide for the hybrid FP laser.

class="figure_img" id="Figure7"/>
Download
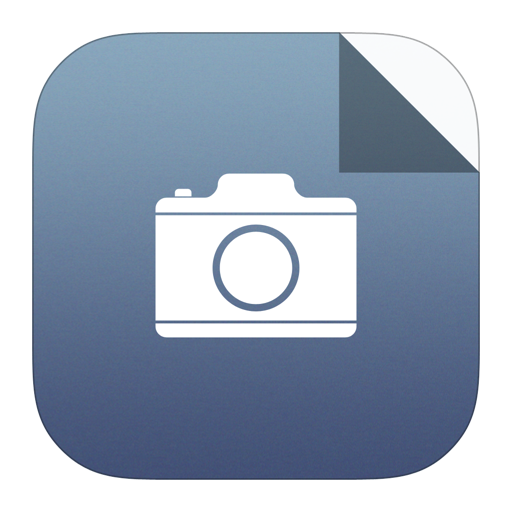
Larger image
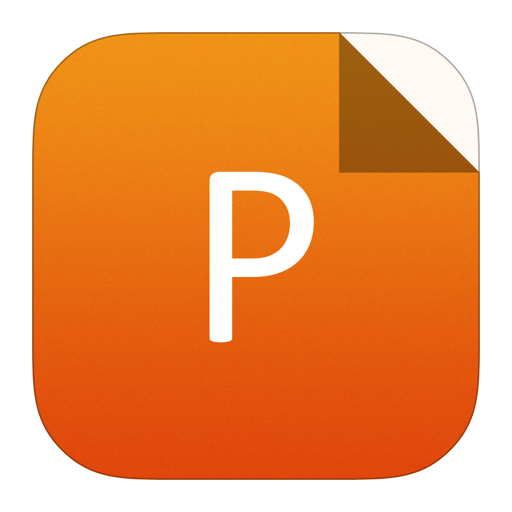
PowerPoint slide
Figure7.
Microscope images of (a) the near-field lasing-emission pattern, and (b) the top-view scattering light from the cleaved silicon waveguide facet of a hybrid FP laser at a pulsed injection current of 40 mA.
Single-mode lasing is also realized by further reducing the cavity length. For the 150 μm-long 6-μm-wide metal-coated hybrid FP laser, the output peak power versus the pulsed injection current, with a repetition rate of 1 MHz and a pulse width of 100 ns, is measured at 285 K and plotted in Fig. 8(a). The threshold current is about 16 mA corresponding to a current density of 1.78 kA/cm2. A series resistance of 27 Ω is estimated according to the V–I curve around the threshold. Fig. 8(b) shows the lasing spectra at pulsed injection currents of 20 and 40 mA at 285 K. The dominant lasing mode jumps from 1539.5 to 1546.4 nm when the injection current increases from 20 to 40 mA, and the corresponding SMSRs are 15 dB and 29 dB, respectively.

class="figure_img" id="Figure8"/>
Download
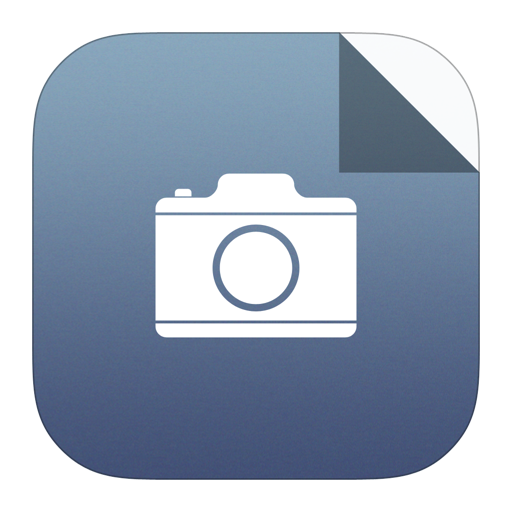
Larger image
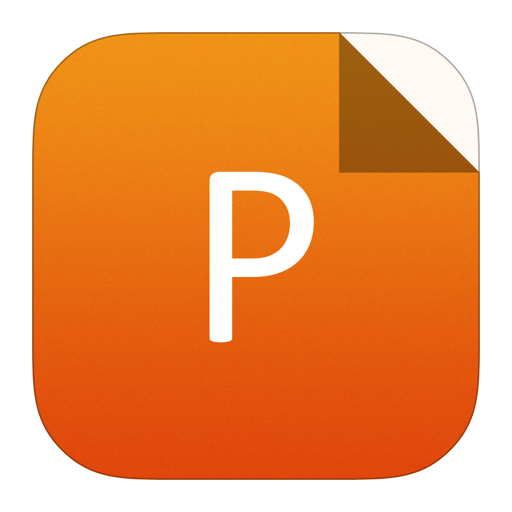
PowerPoint slide
Figure8.
(Color online) (a) The output peak power versus the pulsed injection current, and (b) the lasing spectra at the pulsed currents of 20 and 40 mA, for the metal-coated 150-μm-long 6-μm-wide hybrid FP laser at 285 K.
4.
Summary
In summary, we have proposed and experimentally demonstrated the hybrid AlGaInAs/Si FP lasers, with the FP cavity facet coated by the p-electrode metal for enhancing the mode reflectivity. In such hybrid silicon lasers, the mode fields are mainly confined in the gain region for maintaining a high optical confinement factor, while efficient lasing output is realized through the evanescently-coupled silicon waveguide underneath. The metal-coated hybrid AlGaInAs/Si FP laser with lower threshold current and higher output are demonstrated experimentally compared with the laser without metal coating.