1.
Introduction
Recent years have witnessed the prosperity of perovskite-based solar cell materials, represented by organic-inorganic methylammonium lead halide perovskite (MAPbI3). MAPbI3-based solar cell has refreshed its certificated record of PCE from the first reported 3.8%[1] to now 22.1%[2]. The long-term stability now is the most crucial issue to impede the commercialization. Apart from that, the toxicity of Pb should also be properly taken care of. The longest reported longevity of a perovskite solar cell now is only up to 1 year (with efficiency ~11%)[3], far less than the at least 25 years for silicon solar panels. Confronting these problems, inorganic double perovskites are recently proposed as possible candidates for overcoming those problems. As shown in Fig. 1, in this short review, we will focus on a few double perovskites of intensive recent research and discuss their properties and problems.

class="figure_img" id="Figure1"/>
Download
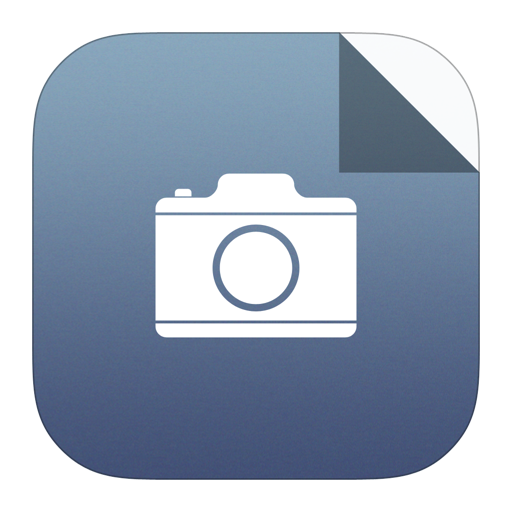
Larger image
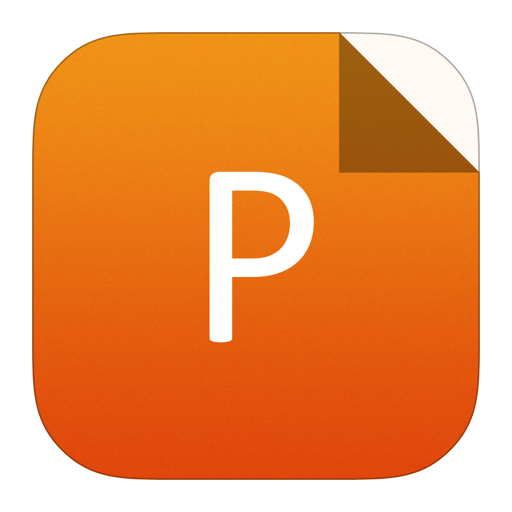
PowerPoint slide
Figure1.
(Color online) Possible route to achieve Pb-free stable perovskite solar cell absorbers, which are discussed in this short review paper.
2.
Isovalent elemental replacement for MAPbI3
Taking MAPbI3 as the benchmark, the most straightforward way to construct a new perovskite is by isovalent ion substitution[4–6] or alloys[7]. According to the perovskite ABX3 formula, diverse A, B and X site ions could be chosen, such as monovalent organic cation (A = NH4+, MA = CH3NH3+, FA = CH(NH2)2) or inorganic cation (Na+, K+, Rb+, Cs+) for A site, divalent cation (Sn2+, Ge2+) for B site, halogen or pseudohalogen (F–, Cl–, Br–, I–, CN–, SCN–) for X site. However, this class of perovskites suffer from the low-valence Sn2+ or Ge2+, which is easily oxidized into high valence state (4+), making them much more unstable than Pb-containing perovskites and hopeless for long-term applications.
3.
Heterovalent elemental replacement for MAPbI3
Double perovskites with a formula A2B1B2X6 that can be considered as the split of B-cation in ABX3 to B1 and B2 cations, offers immense opportunities towards potential photovoltaic materials, since the combinations of multinary elements lead to tremendous possible candidates. As shown in Fig. 2, a simple multiplication of 7 × 8 × 34 × 5 yields a stunning 9520 combinations[8], where these four integers correspond to the number of elements that can occupy the A+, B+, B3+ and X– sites respectively. After applying the Goldschmidt’s rules, we can expect 900 possible compounds (10%), out of which more than 600 compounds have never hitherto been investigated. Therefore, great efforts including both theoretical and experimental works have been recently made to advance the progress in this field.

class="figure_img" id="Figure2"/>
Download
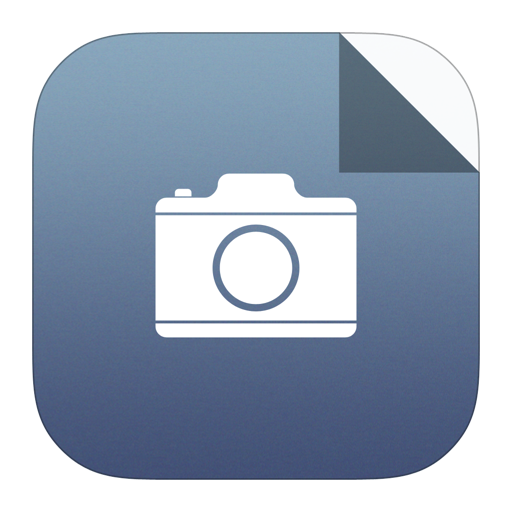
Larger image
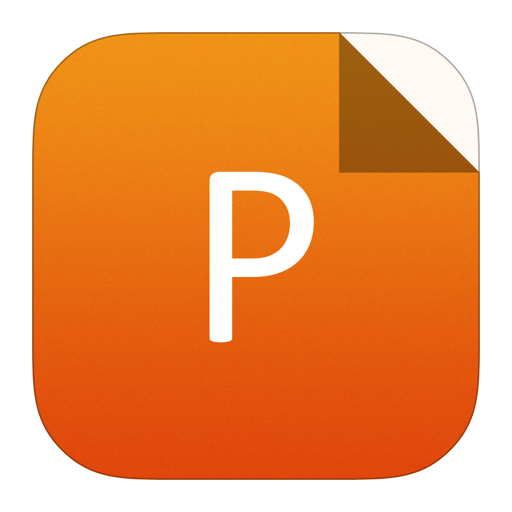
PowerPoint slide
Figure2.
(Color online) Elements forming double halide perovskites with composition A2B1+B3+X6. An element is colored in light blue if at least one compound with double halide perovskites structure containing that element has been synthesized. The triangular color tag specifies the site occupied by the element, according to the legend at the top. The compounds used to generate the table can be found in Ref. [8] and reference therein. Only structures that crystallize in the space group Fm
3.1
Cs2AgBiBr6 and its family Cs2M(IB)M(III)X6 [M(IB) = Cu, Ag; M(III) = Sb, Bi; X = I, Br, Cl]
Cs2AgBiBr6 is the first double halide perovskite that was successfully synthesized via a solid state or solution route by McClure[10] and Slavney[11] [Fig. 3] for potential photovoltaic applications in early 2016 and then stimulating more and more research interests in the field during the last two years. The as-prepared Cs2AgBiCl6 and Cs2AgBiBr6 are cubic perovskites, with space group Fm


class="figure_img" id="Figure3"/>
Download
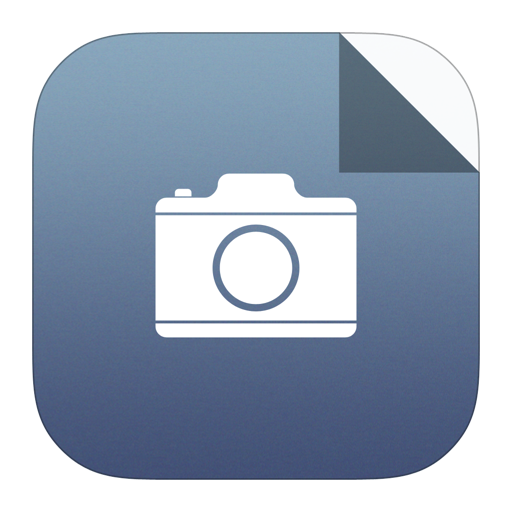
Larger image
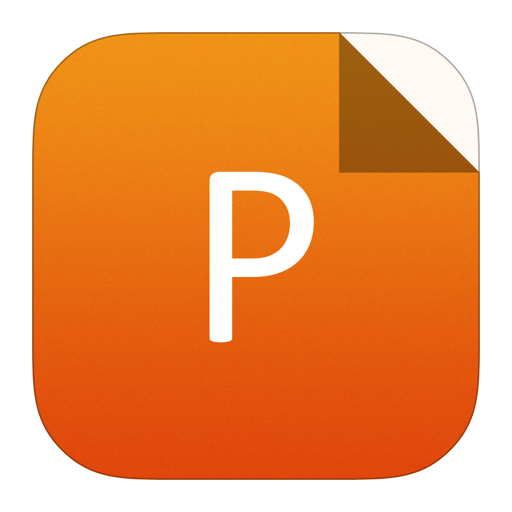
PowerPoint slide
Figure3.
(Color online) (a) X-ray structure of the ordered double perovskite Cs2AgBiBr6. Orange, gray, turquoise, and brown spheres represent Bi, Ag, Cs and Br atoms, respectively. (b) Photograph of a single crystal of Cs2AgBiBr6. (c) The Bi3+ face-centered-cubic sublattice in Cs2AgBiBr6, consisting of edge-sharing tetrahedra. (d) Diffuse reflectance spectra of Cs2AgBiBr6 (blue) and CH3NH3PbBr3 (red). Modified with permission from Refs. [10, 11].
One possible benefit for Cs2AgBiBr6 is the lone-pair s electrons of Bi, which was expected to inherit the superior performance of CH3NH3PbI3 derived from Pb lone-pair s orbitals. Unfortunately, Cs2AgBiX6 (X = Br, Cl) has indirect band gaps due to chemical mismatch between Ag and Bi[12]. As shown in Fig. 4, first principles calculations[10, 13] indicate that the conduction band minimum (CBM) of Cs2AgBiX6 mainly consists of the Bi-6p/halogen-p antibonding states, locating at the L point (π/a, π/a, π/a), while the valence band maximum (VBM) is associated with Ag-4d/halogen-p hybrid orbitals at the X point (2π/a, 0, 0), which can be seen as the shift result from R point (π/a, π/a, π/a) in the direct band gap of MAPbI3, inducing the formation of observed indirect band gap. Apart from that, the band gap size of Cs2AgBiBr6 and Cs2AgBiCl6 are 1.8–2.2 and 2.2–2.8 eV respectively, which are much larger for single-junction solar cell absorber.

class="figure_img" id="Figure4"/>
Download
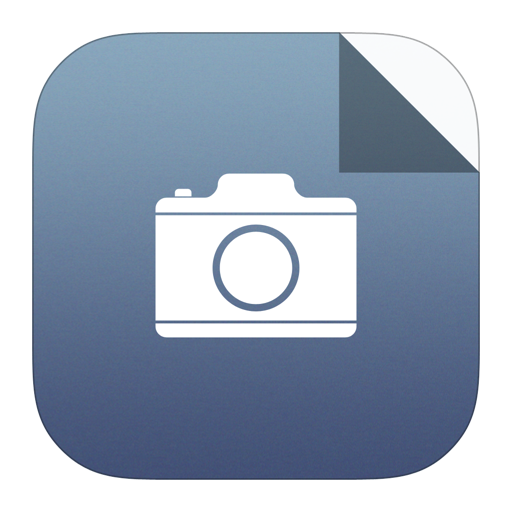
Larger image
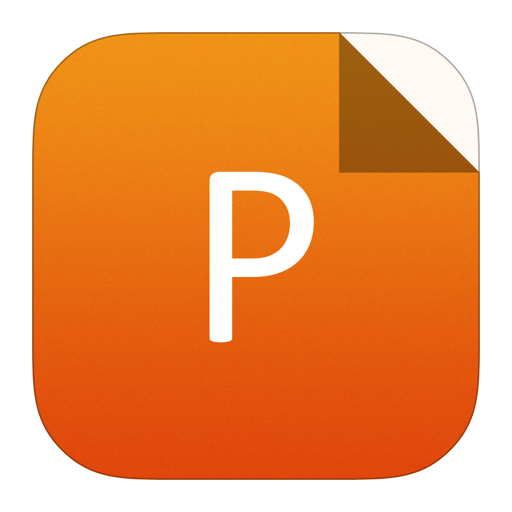
PowerPoint slide
Figure4.
Band structure of (a) CsPbBr3, (b) Cs2AgBiBr6, (c) CsPbCl3 and (d) Cs2AgBiCl6. Reprinted from Ref. [10].
Such indirect band gap feature is favorable for its long carrier recombination lifetime[10, 11], which is 660 ns as determined from the room-temperature time-resolved photoluminescence decay measurement. This value is much larger than the recombination lifetime for high-quality MAPbBr3 films (170 ns), and approximately close to that of MAPbI3 film (0.736–1 μs). Meanwhile, Cs2AgBiCl6 (Cs2AgBiBr6) has an approximate electron effective mass of 0.53me (0.37me) along the L to W direction, while a hole effective mass of 0.15me (0.14me) along the X to Γ direction are calculated, which turns out to be lighter than their lead analogs[10]. Although not an ideal absorber for high-efficiency solar cell, Cs2AgBiBr6 was very recently used as single-crystal X-ray detector with a low detection limit, opening a new direction for the application of lead-free double halide perovskites[14].
Cs2AgBiBr6 shows benign defect properties[15]. Specifically, Ag vacancies are shallow acceptors and can easily form, leading to an intrinsic p-type conductivity, while Bi vacancies and AgBi antisites are deep acceptors and should be the dominant defects under the Br-rich growth conditions, suggesting that to maximize the photovoltaic performance of Cs2AgBiBr6, the formation of deep defects should be suppressed, which means in this case, the growth condition is preferred to be Br-poor/Bi-rich. In contrast, no intrinsic defect can be an effective n-type source (i.e., with shallow transition levels and a low formation energy); therefore, it is unlikely to obtain intrinsic n-type conductivity in Cs2AgBiBr6.
As an alternative to Ag-based double halide perovskites, Cu-based Cs2CuM(III)X6 perovskites have also been proposed by Volonakis[16] et al. and Zhao et al.[17, 18] but so far not successfully synthesized. Xiao et al.[19, 20] widely searched existing Cu-based compounds, in particular Cu halides, and found that different from Ag-based compounds, which could have both 4- and 6-fold coordinations, Cu-based compounds rarely have 6-fold coordination. They concluded that Cu(I) atom energetically favors 4-fold coordination, forming [CuX4] tetrahedral (X = halogen), but not 6-fold coordination as required for [CuX6] octahedra. They explained that Cu 3d[10] electrons are unstable due to the high- energy level and must hybridize with Cu 4s and 4p to lower the energy. Therefore, Cu(I) is more covalent and favors the low coordination environment (Fig. 5).
Instead of three-dimensional double perovskites, a two-dimensional layered structure Cs4CuSb2Cl12[21] has been synthesized. Different from Cu in hypothesized Cs2CuM(III)X6, the Cu in Cs4CuSb2Cl12 is 2+. It has a direct bandgap of 1.0 eV and conductivity 1 order of magnitude greater than that of MAPbI3. However, the potential applications of Cs4CuSb2Cl12 can be limited by its layered structure which has limited carrier mobility perpendicular to the layers[22].
3.2
Cs2AgInCl6 and its family Cs2M(IB)M(III)X6 [M(IB) = Cu, Ag; M(III) = Ga, In; X = I, Br, Cl]
To remedy the indirect band gap of bismuth-based double perovskite, one straightforward element In is taken into consideration. In3+ has no lone-pair s electrons thus can match well with the Ag d orbit, forming a direct band gap[23]. However, the initial idea of Cs2AgInCl6 for potential high-efficiency solar cell absorber is questionable, since both Ag and In have no lone-pair s orbital and the materials should not inherit superior solar cell performance of CH3NH3PbI3[24–27].
Cs2AgInX6 (X = Br, Cl) was firstly synthesized by Volonakis et al. in early 2017[23], with a reported band gap of 1.33 eV for Cs2AgInBr6 and 2.0 eV for Cs2AgInCl6, but the observed optical band gap of Cs2AgInCl6 is 3.3 eV. The confusing discrepancy hindering its application in solar cells comes from the inversion-symmetry-induced parity-forbidden transitions[28] claimed by Meng et al. (Fig. 6), which explain very well why the synthesized powders exhibit white coloration with an experimentally measured optical band gap of 3.3 eV, while the photoluminescence (PL) emission energy is only 2.0 eV. For double perovskite with a formula A2B1B2X6, as shown in Table 1, nine possible types of Pb-free metal halide double perovskites are designed, among which, six have direct band gaps. Of these six types, four show inversion-symmetry-induced parity-forbidden or weak transitions between band edges, making them not ideal for thin-film solar cell applications. Only one type of Pb-free double perovskite shows optical absorption and electronic properties suitable for solar cell applications, namely, those with B+ = In, Tl and B3+ = Sb, Bi. Unfortunately, Tl is extremely toxic and In1+-based perovskites are proven to be unstable under redox reaction[19] (Fig. 5), although tuning tolerance factor by mixing Cs/MA/FA at A site may stabilize A2InBiBr6 to some extent[29]. The instability of In1+ is an intrinsic problem that would impede the long-term application for those compounds.

class="figure_img" id="Figure5"/>
Download
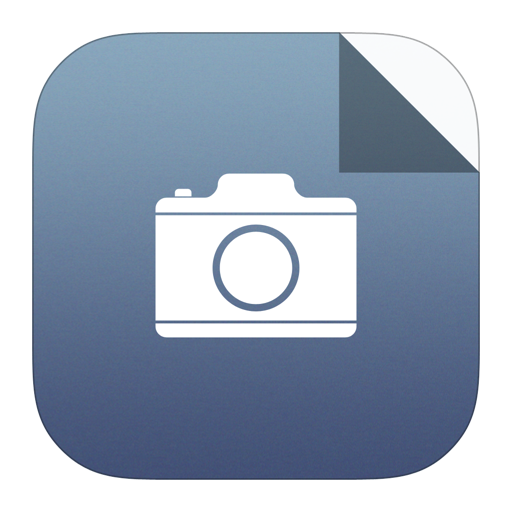
Larger image
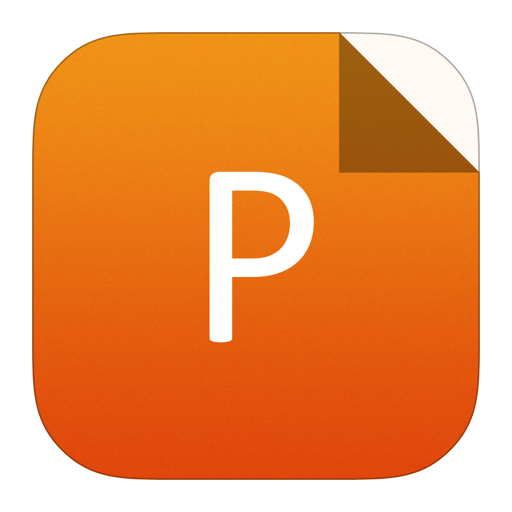
PowerPoint slide
Figure5.
(Color online) Calculated decomposition energies (ΔHD) of In1+ (left), Cu1+ and Ag1+ (right)-related double halide perovskites under different decomposition pathways. Positive ΔHD means stable and negative ΔHD means unstable. Modified with permission from Refs. [19, 20].

class="figure_img" id="Figure6"/>
Download
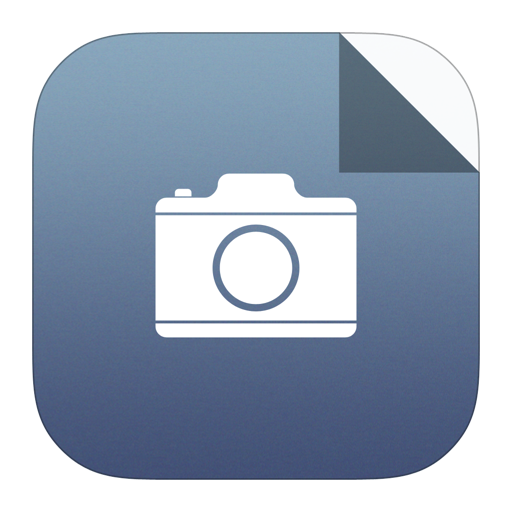
Larger image
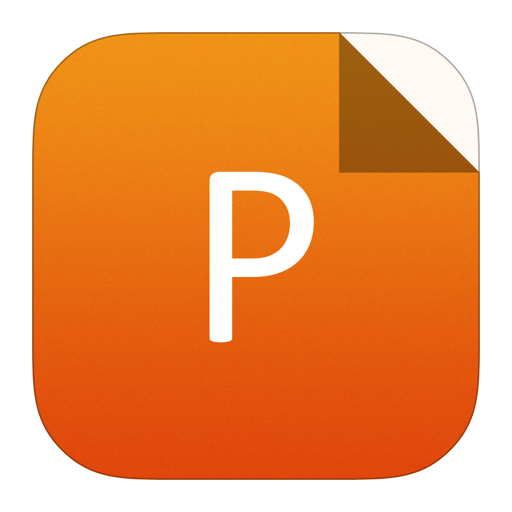
PowerPoint slide
Figure6.
(Color online) The nature of optical transitions for possible combinations of A2B1+B3+X6 double halide perovskites. Reprinted from Ref. [28].
3.3
Double halide perovskite alloys between Cs2AgBiBr6 and Cs2AgInCl6 families
As discussed above, Cs2AgBiBr6 contains lone-pair s orbital elements and may inherit some of the superior properties of CH3NH3PbI3 such as balanced carrier effective mass but suffer from the indirect band gap. Cs2AgInCl6 has direct band gap but dipole-forbidden band edge transition and there is no B-site element with lone-pair s electron. A straightforward idea is to alloy two perovskite families and expect to see the combined advantage of the two.
Du et al.[30] synthesized alloyed double perovskites Cs2Ag(Bi1?xInx)Br6 and Cs2Ag(Bi1?xSbx)Br6 with x ∈ [0, 0.75] and x ∈ [0, 0.375] respectively, with the double perovskite phase being unstable substantially outside the listed range of x. Notably, increasing In content enlarges the band gap, whereas increasing Sb content reduces the band gap. Tran et al.[31] synthesized Cs2AgSbxIn1?xCl6 intending to take advantage of both Cs2AgSbCl6 and Cs2AgInCl6. Results revealed that at x = 0.4, the phase diagram showed a crossover from indirect to direct optical absorption at 2.92 eV (Fig. 7). Although they seem promising, the most severe problem of alloyed double perovskites is still their instability and narrow phase diagram region. On the other hand, although In corporation at B-site can make Cs2AgBiBr6 family a direct band gap, the nature of direct band gap originates from Cs2AgInCl6 and may not have very strong optical absorptions at band edge like Cs2AgInCl6. Therefore, more detailed studies on the strength of optical absorptions for the alloys are still called for.

class="figure_img" id="Figure7"/>
Download
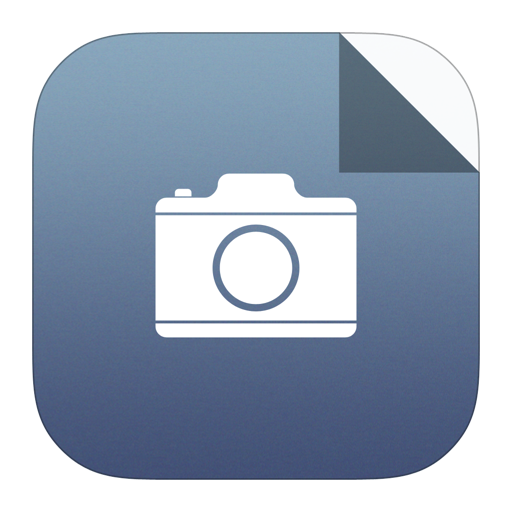
Larger image
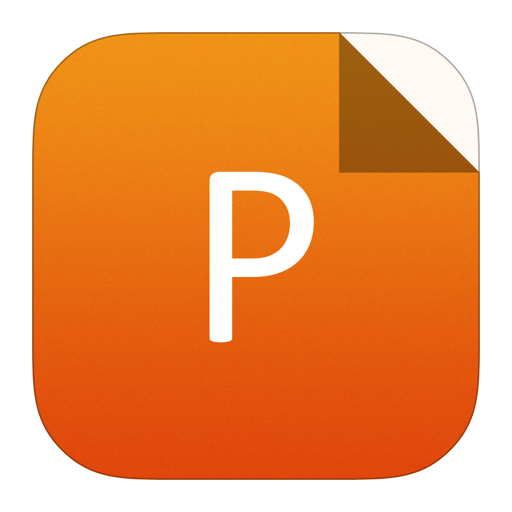
PowerPoint slide
Figure7.
(Color online) (a) Tauc plots for Cs2AgSbCl6, Cs2AgSb0.5In0.5Cl6, Cs2AgSb0.4In0.6Cl6, Cs2AgSb0.2In0.8Cl6 and Cs2AgInCl6. The plots show the characteristics of an indirect bandgap for Cs2AgSbCl6, a direct bandgap for Cs2AgInCl6 and a transition from indirect to direct bandgap of Cs2AgSbxIn1?xCl6 at x = 0.4. (b) Phase diagram for Cs2AgSbxAg1?xCl6 showing the bandgap trend as a function of Sb composition and a crossover from indirect to direct optical absorption in a solid solution at x = 0.4.
4.
Outlook
Currently, numerous types of double perovskites are designed according to diverse design principles and progress is made with the successful synthesis of several representative double perovskites, such as Cs2AgBiBr6 and Cs2AgInCl6. However, all those double halide perovskites have their own intrinsic electronic and optical problems. More importantly, the long-term stability of those halides is not proven. Therefore, there are few reports of working devices on those class of materials. Frankly speaking, it does not seem optimistic to find stable Pb-free double perovskites that have performance comparable to their organic–inorganic counterpart MAPbI3 or FAPbI3. But as mentioned above [Fig. 2], there is still a huge territory of perovskites waiting to be charted. High-throughput calculations with material genome technologies can be applied here for effectively searching stable Pb-free perovskite for high-efficiency solar cell applications.