1.
Introduction
Due to the requirement of high crystalline quality and smooth surface morphology in fabrication of optoelectronic and electronic devices, GaN, AlN and AlGaN epitaxial thin films were mostly grown on foreign substrate with metal polarity (0001) direction[1, 2]. Recently, N-polar III-nitride has attracted great interests due to its unique optical and electrical property[3]. N-polar light-emitting-diode shows higher radiative recombination rate inside active region compared to III-polar device since the built-in electrical field of N-polar film follows the same direction as that of the internal polarization field[3]. Earlier reports suggested that surface polarity of III-nitride can be controlled by low temperature buffer layer deposition and NH3 nitridation conditions[4]. N-polar thin films were systematically obtained on bare sapphire substrate with NH3 annealing at temperature higher than 1000 °C; on the other hand, Ga-polar or Al-polar thin films can be achieved on regions where low temperature AlN buffers act as a nucleation layer[5]. Polarities of III-nitrides can be easily distinguished by the observation of surface morphologies: III-polar thin films are usually atomically smooth, while N-polar thin films are composed of hexagonal hillocks of several micrometers.
Despite great improvements made on polarity control of III-nitride thin films and numerous investigations performed on dislocation behavior, surface stoichiometry and atom bonding conditions[6, 7], there are seldom reports on simultaneously growing III-polar and N-polar nitride thin films side by side on sapphire substrate. Hite et al.[8] reported a periodically oriented GaN on sapphire substrate with well-defined boundaries between N-polar and Ga-polar domains. Tuning growth conditions of MOCVD can result in the same growth rate of N-polar and Ga-polar GaN where the whole surface is uniform. Following a similar idea, Collazo et al. grew lateral-polarity structured (LPS) GaN on sapphire and fabricated a depletion-mode metal semiconductor field effect transistor (MESFET). An improvement in contact resistance was observed[9]. It was suggested that application of lateral III-polar/ N-polar structures in semiconductor devices is advantageous, where oxygen defect concentration, spontaneous polarization field and thus non-linear optical coefficients can be periodically changed[10], which has potential use in lateral p-n devices, waveguide formation and second-harmonic generation, etc[11].
In this work, we report low temperature (LT)-AlN buffer layers patterned by photolithography and subsequent epitaxial growth of both GaN and AlN thin films via MOCVD. N-polar and III-polar domains were simultaneously grown on the substrate. Surface morphologies, chemical reactivity, and strain conditions of N-polar and III-polar III-nitride thin films were investigated in detail. Due to the surface energy difference between two polarities, N-polar regions are n-type conductive while Ga-polar regions are semi-insulating[12]. Utilizing this effect, GaN Schottky barrier diode structure was fabricated with ohmic contact deposited on N-polar GaN while Schottky contact deposited on Ga-polar GaN. The influence of lateral polarity control on forward and reverse current of the device was further investigated.
2.
Experimental details
20 nm low temperature AlN buffer was grown on 2 inch sapphire substrates via AMEC Prismo D-Blue? metalorganic chemical vapor deposition (MOCVD) at 800 °C. The buffer layer was patterned by standard photolithography, and periodic patterning was achieved by reactive ion etching (RIE). Patterned AlN buffer substrate was re-loaded into the MOCVD chamber and 3 μm GaN, 1 μm AlN were epitaxially grown at temperature of 1100 and 1250 °C, respectively. III-nitride grown on AlN buffer exhibits III-polarity, while III-nitride grown on RIE etched sapphire substrate shows N-polarity. Surface morphologies of III-polar and N-polar III-nitride epitaxial layers were characterized by Olympus differential interference contrast (DIC) optical microscopy and Veeco dimension 3100 V atomic force microscopy. Strain condition of GaN was studied by Renishaw Raman spectroscopy.
A prototype Schottky barrier diode structure was fabricated based on conventional Ga-polar GaN and LPS GaN where Ga-polar and N-polar domains are oriented side by side. Ring-shape ohmic contact consisting Ti/Al/Ni/Au metal layer was e-beam evaporated on N-polar region of the GaN followed by lift-off. Rapid thermal annealing (RTA) was performed on the ohmic contact at temperature of 800 °C for 1 min. Ni/Au Schottky contact was deposited on Ga-polar domains followed by RTA at 300 °C for 1 min. I–V characteristic of Schottky barrier diode (SBD) was analyzed by Keithley 4200-SCS semiconductor characterization system.
3.
Results and discussions
AFM image of as-deposited 20 nm LT-AlN buffer on top of 2 inch sapphire substrate is shown in Fig. 1(a). LT-AlN buffer exhibits polycrystalline morphology with rms roughness of 2.26 nm. GaN and AlN grown on as-deposited LT-AlN buffers exhibit III-polarity. Surface morphologies are smooth, bi-layer steps and step-bunching are observed in Figs. 1(b) and 1(c), respectively. Surface morphology of RIE etched LT-AlN buffers is indicated in Fig. 1(d). It was shown that rms roughness decreases to 0.5 nm compared to as-deposited AlN buffer, similar to that of bare sapphire substrate, indicating that LT-AlN buffers are completely removed. After NH3 nitridation of 5 min at 1100 °C, surface damage on sapphire substrate was recovered. As shown in Figs. 1(d) and 1(e), rough surfaces with 3D growth mode are observed in N-polar GaN and AlN due to smaller surface energy of N-polarity compared to III-polarity[13]. Additionally, surface morphologies of N-polar GaN and AlN are also different. N-polar GaN is covered with irregular 3D spirals with dimensions larger than 1 μm, whereas N-polar AlN exhibits columnar structure that is coalesced at the bottom of the thin film. This could be attributed to larger surface diffusion length of Ga atoms compared to Al atoms[14]. Polarities of GaN and AlN can be unambiguously distinguished by KOH wet chemical etching, as previously reported in reference[15]. After 3 mol/L KOH etching for 10 min, GaN surface was transformed into random distributed small hillocks bounded by (10–1–1) crystallographic planes. In contrast, the surface morphology of Ga-polar domains remains unchanged due to negatively charged dangling bonds on N atoms in III-polar thin films[16].

class="figure_img" id="Figure1"/>
Download
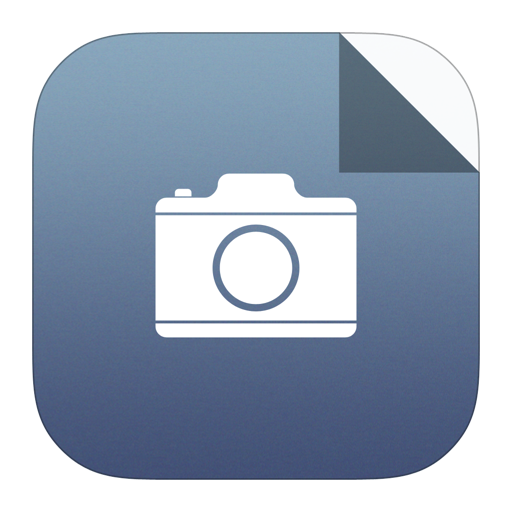
Larger image
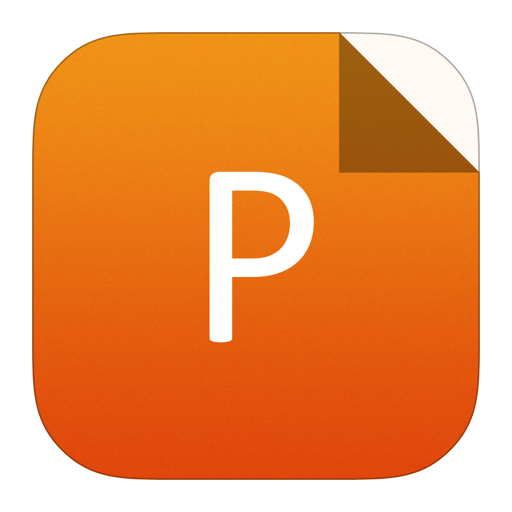
PowerPoint slide
Figure1.
(Color online) AFM images of (a) low temperature AlN buffer, (b) GaN and (c) AlN epitaxial thin films grown on AlN buffers, (d) RIE etched AlN buffers on top of sapphire substrate, (e) GaN and (f) AlN epitaxial thin films grown on nitridated surface of RIE etched AlN buffers.
Usually, most GaN and AlN epitaxial thin films exhibit remnant strains due to lattice mismatch and thermal mismatch between thin films and foreign substrate. Strains are the main cause of dislocations and thin film cracking, which are undesired in device fabrication. Therefore, in order to understand the strain behavior of LPS GaN and AlN thin films to further optimize device structure, Raman spectroscopies of N-polar and III-polar GaN, AlN LPS were characterized. Raman shift of E2 (high) mode was used to estimate strain state of semiconductor thin films[17]. Peak position of 571.5 cm?1 is observed in Fig. 2(a) for both N-polar and Ga-polar LPS GaN. If the position of strain-free GaN at 567.6 cm?1 was utilized[18], a compressive strain of ?1.52 GPa can be derived. However, from Raman spectroscopy of AlN LPS, peak positions of N-polar and Al-polar AlN are 658.5 and 654.8 cm?1, respectively. When E2 (high) position of strain-free AlN of 656 cm?1 was used, a compressive strain of ?0.74 GPa and tensile strain of 0.35 GPa were achieved for N-polar and Al-polar AlN domains, respectively[19]. Strain can be relaxed through surface roughening and column growth, where dislocations bend at the column interfaces. The discrepancy of strain condition between GaN and AlN could be attributed to much larger surface diffusion length of Ga atoms during MOCVD, in which case Ga atom diffusion at inversion domain boundaries (IDB) balanced out the strain difference. In contrast, Al atoms have smaller diffusion length, thus strain state in two polarities cannot fully compromise. In both cases, no surface cracking or buckling were observed, suggesting that polarity control is a promising approach in achieving crack-free, high efficiency optoelectronic and electronic devices.

class="figure_img" id="Figure2"/>
Download
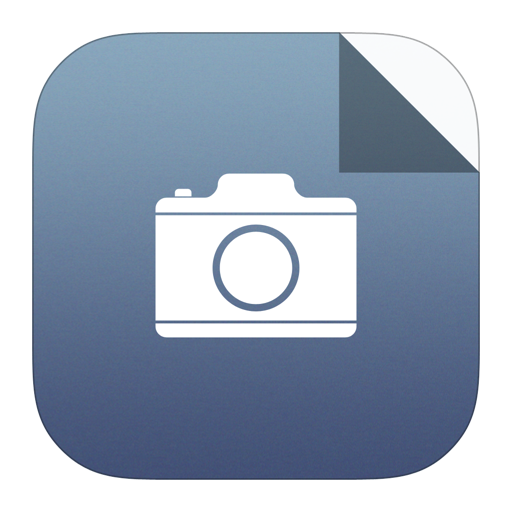
Larger image
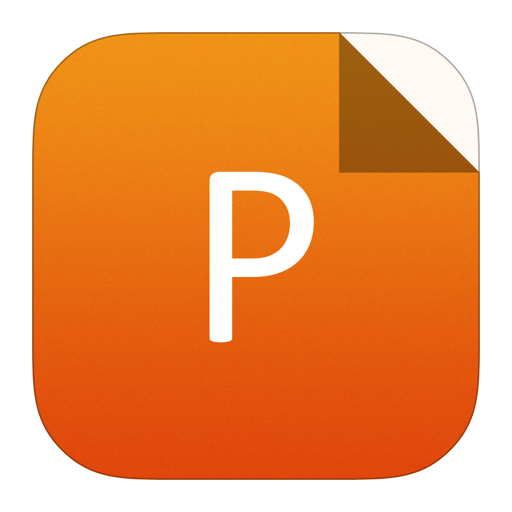
PowerPoint slide
Figure2.
(Color online) Raman spectroscopy of (a) Ga-polar, N-polar GaN thin film and (b) Al-polar, N-polar AlN epitaxial thin film grown on patterned AlN buffers.
Followed by III-nitride polarity control and fabrication of LPS, we further utilize the LPS in the application of GaN SBD, usually doping is necessary to achieve good ohmic contact, however, this inevitably leads to high leakage current and early reverse breakdown. Utilizing polarity control scheme of III-nitride material, selective doping can achieve LPS GaN, where N-polar GaN has high electron concentration of 1.2 × 1019 cm?3, while Ga-polar GaN exhibits a carrier concentration of only 1.4 × 1017 cm?3. The influence of polarity control on ohmic contact resistivity and electrical behavior of SBD was discussed. Fig. 3(a) shows DIC microcopy of lithography patterned LT-AlN buffers, and Fig. 3(b) shows 3 μm GaN grown on patterned LT-AlN buffers. N-polar is covered with hexagonal hillocks while Ga-polar is relatively smooth, which is consistent with AFM results shown in Fig. 1. There is a ring-shape region at the IDB, where N-polar diffused into Ga-polar by 20 μm laterally. Schematic structures of conventional SBD and LPS-SBD are shown in Fig. 3(c), and I–V characteristic was compared in Fig. 3(d). For conventional SBD, ohmic contact was annealed in 800 °C for 1 min. For LPS SBD, ohmic contact was either as-grown or annealed at 800 °C for 1 min. Schottky contacts were all annealed at 300 °C for 1 min. As can be seen from I–V curve, forward current reaches at 10?5 A at 10 V, and reverse current is lower than 10?10 A at ?10 V. When ohmic contact was deposited on N-polar domains, forward current dramatically increased, Ron decreased from 1000 Ω·cm2 in conventional SBD to 567 Ω·cm2 in LPS SBD with as-grown ohmic contact. When ohmic contact was further annealed, rectification ratio further enhanced, indicating an improved performance of SBD. Ron further decreased to 77 Ω·cm2. However, it must be noted that reverse leakage current for LPS-based SBD is still relatively high compared to conventional SBD. This could be attributed to existence of dislocations or nanoscale inversion domains at the interface between N-polar and Ga-polar, which act as non-radiative recombination centers and current leakage paths. Fig. 3(e) illustrates the tilted view SEM image of N-polar domains adjacent to IDB after KOH etching. Random distributed small hillocks and irregular mesas are observed, indicating existence of mix-polarity due to surface damages at the interface[16, 20]. Fig. 3(f) shows the tilted view SEM image of GaN LPS in IDB, where left side is N-polar and right side is Ga-polar. A height difference of approximately 5 μm can be ambiguously identified, suggesting the lateral atom diffusion due to surface energy differences between N-polar and Ga-polar GaN[21, 22]. Further improvement in interface quality and smoothness is under investigation in order to reduce reverse leakage current of SBD. One of the most important considerations is surface passivation of SiN or SiO2 at IDB, in which case traps and defects can be annihilated. Another approach involves the optimization of MOCVD condition. V/III ratio will be tuned in order to achieve N-polar and Ga-polar with similar heights, reducing current leakage[23]. In all, the results have demonstrated that the utilization of LPS in the design of SBD provides an alternative way in realizing lateral n+/n? devices without intentional doping, which is promising in future development of high performance SBD and other electronic devices.

class="figure_img" id="Figure3"/>
Download
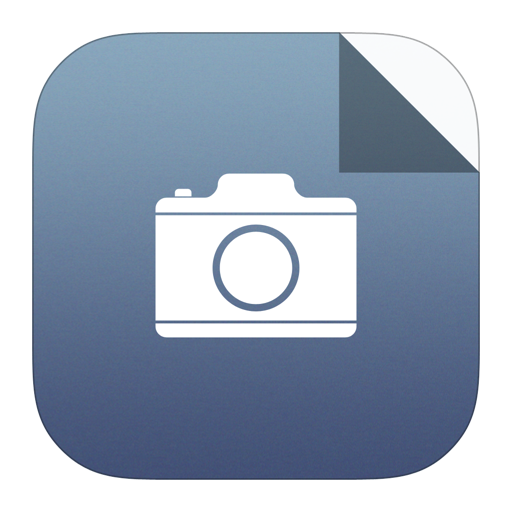
Larger image
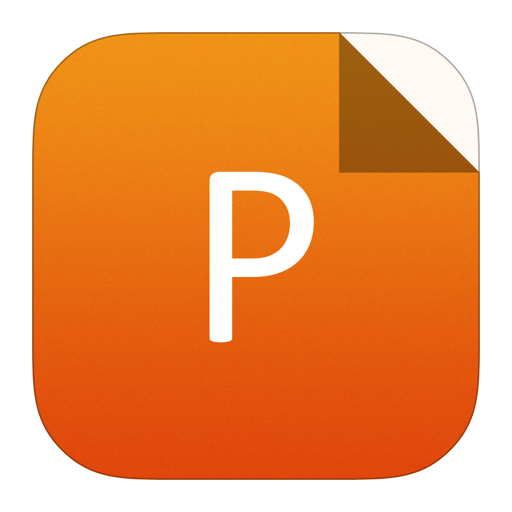
PowerPoint slide
Figure3.
(Color online) DIC microscope images of lithography patterned low temperature (a) AlN buffers and (b) GaN LPS grown on top of the patterned AlN buffers. (c) Schematic structure of conventional Schottky barrier diode (SBD) and SBD fabricated on LPS GaN. (d) I–V characteristic of conventional SBD and LPS SBD. (e) Tilted view SEM images of N-polar domains adjacent to IDB after KOH etching. (f) Tilted view SEM images of IDB with N-polar and Ga-polar domains grown side by side.
4.
Conclusion
In summary, GaN and AlN lateral polarity structures were grown by MOCVD on sapphire substrate. N-polar and III-polar exhibit dramatic difference in surface morphology and chemical etching susceptibility. Raman spectroscopy indicated that N-polar and Ga-polar GaN have similar strain conditions. However, in AlN LPS, Al-polar AlN domains exhibit tensile strain while N-polar AlN domains show compressive strain due to smaller diffusion length of Al atoms. A prototype Schottky barrier diode structure was fabricated on GaN LPS, forward current increased by 4 magnitudes, taking advantage of better ohmic contact between N-polar GaN and Ti/Al/Ni/Au metal stack. Lateral polarity control of GaN and AlN thin films has provided promising opportunities in future development of high efficiency optoelectronic and electronic devices.