
Corresponding authors: ?E-mail:ssbao@sdu.edu.cn
Received:2019-03-13Accepted:2019-05-31Online:2019-09-1
Fund supported: |

Abstract
Keywords:
PDF (211KB)MetadataMetricsRelated articlesExportEndNote|Ris|BibtexFavorite
Cite this article
Shou-Shan Bao. Study of the FCNH Coupling with Boosted Higgs at LHC*. [J], 2019, 71(9): 1093-1096 doi:10.1088/0253-6102/71/9/1093
1 Introduction
The discovery of the Higgs boson at Large Hadron Collider (LHC)[1-2] is a great triumph of the Standard Model (SM). Following that, one can expect to test and search new physics through the precision measurement of the Higgs boson properties. In SM, the Higgs doublet is introduced to generate masses for the gauge bosons and the fermions. Most parameters in SM are related to Yukawa couplings. But the large difference between the Yukawa couplings has no answer in SM. On the other hand, the flavor changing neutral current couplings are absent at tree-level and suppressed at loop-level in SM. So that such processes are thought to be good clues to the new physics beyond SM, which have been widely studied through B-meson decays.At present, the top quark is the heaviest elementary particle discovered, the mass of which is close to the electro-weak scale and could provide better clues to study the mechanism of electro-weak symmetry breaking and new physics beyond SM. The flavor changing neutral Higgs (FCNH) interactions of top quark are small and the branch ratios of FCNH decays are predicted as Br$(t\to Hc)\sim 10^{-14}$ and Br$(t\to Hu)\sim 10^{-17}$ in SM,[3-4] which are far below the sensitivity of LHC. Several extensions to the SM can enhance such interaction at tree level or loop level, e.g. two Higgs doublet model,[5-7] supersymmetry model,[8-10] and other new physics models.[11] One can expect that some new physics contributions would play significant roles in such FCNH interactions and such contribution could be discovered or constrained at LHC. The aim of this work is to study the sensitivity of LHC to the FCNH couplings of top quark.
In this work, we focus on the effective FCNH interaction between the Higgs boson and the top quark which can be expressed in general as[12-13]
where the $y_{tq}$ is the flavor changing coupling constant. Such anomalous couplings have been studied through $t\to Hq$ rare decays,[13-15] the single top quark production with Higgs boson,[16-18] and same sign top quark production.[19] Through $pp\to t\bar{t}$ with $t\to H q$ using $H\to b\bar{b}$ channel, ATLAS[20] and CMS[21-22] set upper limits at the 95% confidence level (C.L.) on the couplings as
In this work, the process $pp\to H t/\bar{t}$ with $H\to b\bar{b}$ and $t\to b l\nu$ is considered. This process suffers large background from $pp\to t\bar{t}\to l^\pm+\mathbb{E}_{T}+bbjj$. Since the $b-$jets in background are from two different top quarks, they would be separated largely if the top quarks are boosted. At other side, the two nearby, separate resolved b-jets in signal events can be used to reconstruct the boosted Higgs boson efficiently.[23]
This work is organized as follows. In Sec. 1, a brief introduction is given. In Sec. 2, the cross-section of the signal process $pp\to tH$ is calculated. In Sec. 3, the backgrounds and the significance are analyzed. Finally, a summary is given in Sec. 4.
2 Cross-Section of $Ht/\bar{t}$ at LHC
With the effective interactions in Eq. (1), the Feynman diagrams for the production of Higgs boson associated with a single top quark are shown in Fig. 1. Due to the effect of Parton Distribution Functions (PDF), the cross-section of $g\bar{u}\to H\bar{t}$ is overwhelmed by $gu\to H t$ while the cross-section of $g\bar{c}\to H\bar{t}$ is same to that of $gc\to Ht$ at LHC. One can choose the signal $gu\to H t(bW^+) $ with half backgrounds to study the effective interaction $y_{\rm tu}$ while combine $gc\to Ht $ and $g\bar{c}\to H \bar{t}$ to study the coupling $y_{\rm tc}$. In this sense, one can get a more stringent constrain on $y_{\rm tu}$ than that on $y_{\rm tc}$ at LHC.Fig. 1
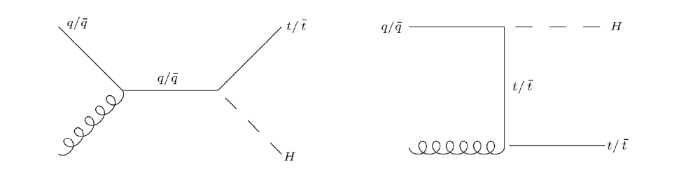
Fig. 1The Feynman diagrams of $H t/\bar{t}$ production at LHC, where $q=u, c$.
The numerical values of the inputs are listed in Table 1. The PDF set CT14NNLO[25] is used in this work. With these inputs, the leading order cross-sections are obtained and shown in Table 2, where the NLO QCD correction has been studied in Ref. [17]. In the analysis, the events are generated at tree-level and the cross-sections are rescaled with the QCD $k$-factor as shown in Table 2.
Tab.1
Tab.1
![]() |
New window|CSV
Tab.2
Tab.2
![]() |
New window|CSV
If one takes $y_{\rm tc}={\sqrt{m_t m_c}}/{v}\simeq 6\times 10^{-2}$ which parametrization was used in Refs. [6-7], there would be
where $l^\pm$ denotes $\mu^\pm$ and $e^\pm$.
The $H\to b\bar{b}$ decay mode provides a promising avenue for the $H t/\bar{t}$ searching due to the large branching ratio of this channel. Considering semi-leptonic decay of top quark, the final states of the signal are $l^\pm+\mathbb{E}_{T}+3b$. The dominated background is $pp\to t\bar{t}\to l^\pm+\mathbb{E}_{T}+bbjj$.
Top quark pair production in hadron collision can be calculated in perturbative QCD. However, higher order QCD corrections involving either virtual or real gluon emission lead to a sizable enhancement to the cross-section. Large logarithmically enhanced corrections due to soft-gluon radiation need to be resumed to improve the result. The $pp\to t\bar{t}$ events are generated at LO and then rescaled to the NNLO+NNLL total cross-section as studied in Ref. [26]. Other background like $W+3j$ as studied in Refs. [17-18, 27] can be suppressed strongly by the b-tag and large $p_T$ cuts. In this work, only the $t\bar{t}$ background is considered. All the events are simulated in MG5[28]+Pythia[29]+Delphes[30] with FastJet.[31] The Snowmass parameterizations[32-33] are applied in the simulations.
3 Cuts and Selections
The event selection requires one isolated high $p_T$ lepton ($e$ or $\mu$), missing energy $\mathbb{E}_{T}$ in the W boson decay, and three tagged b-jets, one of which is from the top quark decay and the others are from the Higgs boson decay. Since then the basic acceptance cuts referred as Cut-I are applied as follows.$\bullet$ Select the events with number of jets $3\leq N_j\leq4$ and number of charged lepton $N_l=1$.
$\bullet$ Select the events 3 b-jets with $P_T>$25 GeV and $|\eta|<$2.5.
$\bullet$ Charged lepton $P_T>$25 GeV and $|\eta|<2.5$ while the missing $E_T$ $\mathbb{E}_{T}>$25 GeV.
$\bullet$ Apply the cuts $\Delta R_{\alpha\beta}>0.4$ ($\alpha,\beta=$jets and lepton).
The three b-jets in the selected events are from the Higgs boson decay and top quark decay. To specify them, the all possible invariant mass $m^2_{\alpha\beta}=(p_{b_\alpha}+p_{b_\beta})^2$ are calculated. The two b-jets whose invariant mass are most close to the Higgs boson mass 125 GeV are chosen to construct the Higgs boson
The distributions of the signal and background on the $\Delta R$ and $m^r_H$ of the two chosen b-jets are shown in Fig. 2. The signal-background ratios $S/B$ at different range of $\Delta R $ are given in Table 3. According the distributions, the following cuts are introduced as Cut-II,
Fig. 2
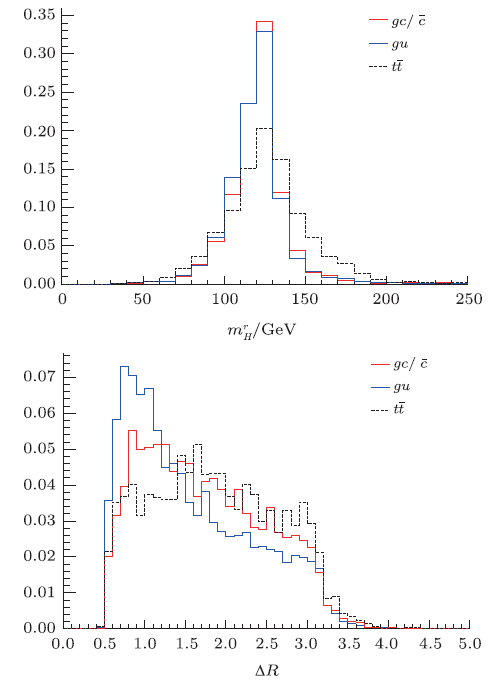
Fig. 2The normalized distributions of the $m^r_{H}$ and $\Delta R_{b\bar{b}}$.
Tab.3
Tab.3
![]() |
New window|CSV
The charged lepton and left b-jet would be recognized as the final states from top quark decay.
The plot of the distribution $({1}/{\sigma})/({d\sigma}/{d p_T({\rm top})})$ is given in Fig. 3. The signal-background ratios in different range of $p_T(\rm top)$ are also got and listed in Table 4. To construct the Higgs boson, the two b-jets with small $0.4<\Delta R<1.4$ were selected. That means the Higgs boson could be boosted with large $P_T$. Since then the cut of top quark transverse momentum is applied as $P_T({\rm top})>160$ GeV, which is referred as Cut-III in this work.
Fig. 3
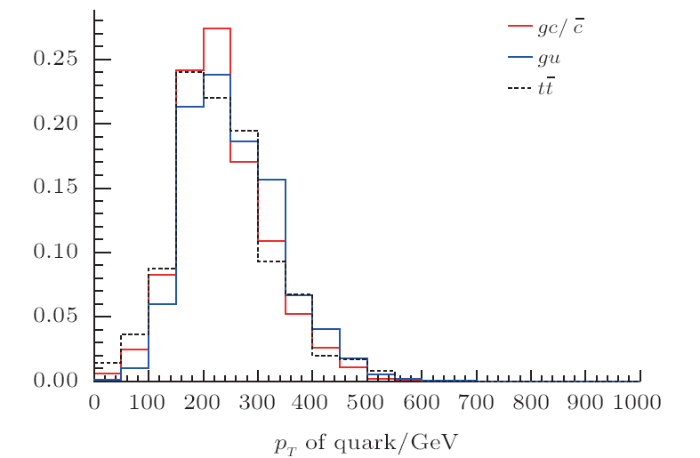
Fig. 3Distribution of top quark $p_T$.
Tab.4
Tab.4
![]() |
New window|CSV
The efficiencies of the above cuts are listed in Table 5. To estimate the LHC detect potential to the FCNH couplings, the significance of the signal events ($N_S$) relative to a background events ($N_B$) in terms of the Gaussian statistics is defined as
for which a signal at 95% (99%) C.L. corresponds to $\sigma_S=2(3)$. With the definition and the cut efficiencies, the limits of the FCNH couplings at LHC with high luminosity $\mathcal{L}=3000 {\rm fb}^{-1}$ could be obtained as
Tab.5
Tab.5
![]() |
New window|CSV
This limits of FCNH couplings can be translated to limits of the top quark rare decay brach ratios,
One can see that the $H t/\bar{t}$ production channel has more sensitivity to $y_{\rm tu}$ than $y_{\rm tc}$ at LHC, while the process $pp\to t\bar{t}$ with $t\to Hq$ has the similar sensitivity to $y_{\rm tu}$ and $y_{\rm tc}$.
4 Conclusion
In this work, the anomaly Yukawa couplings $y_{\rm tq}$ are studied through single top quark production associated with an SM Higgs boson. Such anomaly couplings can be introduced at tree-level or enhanced at loop-level in some new physics models. The boosted region is considered to reconstruct the Higgs boson and top quark. It is found that, the reconstruction is efficient in this region at LHC. One can expect such region more efficient at higher energy collider such as SPPC[34-35] in the future. And also the limits on the FCNH coupling of top quark could be set more stronger in 100 TeV proton-proton collision as studied in Ref. [36]. With high luminosity, the LHC could constrain the $y_{\rm tq}$ strongly. Through $H\to b\bar{b}$ channel, the limits could be set as Br$(t\to H c)\leq 4.1\times10^{-3}$ and Br$(t\to H u)\leq 6.2\times10^{-4}$ at 95% C.L. with $3000 {\rm fb}^{-1}$ at LHC.Acknowledgments
The author would like to thank C. Kao and T. Han for helpful discussions.Reference By original order
By published year
By cited within times
By Impact factor
[Cited within: 1]
[Cited within: 1]
[Cited within: 1]
[Cited within: 1]
[Cited within: 1]
[Cited within: 1]
[Cited within: 2]
[Cited within: 1]
[Cited within: 1]
[Cited within: 1]
[Cited within: 1]
[Cited within: 2]
[Cited within: 1]
[Cited within: 1]
[Cited within: 2]
[Cited within: 2]
[Cited within: 1]
[Cited within: 1]
[Cited within: 1]
[Cited within: 1]
[Cited within: 1]
[Cited within: 1]
[Cited within: 1]
[Cited within: 1]
[Cited within: 1]
[Cited within: 1]
[Cited within: 1]
[Cited within: 1]
[Cited within: 1]
[Cited within: 1]
[Cited within: 1]
[Cited within: 1]
[Cited within: 1]
[Cited within: 1]