HTML
--> --> -->Constructing a reasonable nuclear potential between an α-particle and a daughter nucleus is the most crucial issue in many α decay theoretical models, because the α decay half-life is mainly determined by the barrier penetrating probability [22]. There are many α decay models choosing different nuclear potentials between the α-particle and the daughter nucleus, such as the Coulomb and proximity potential model with proximity potential [23-26], the two-potential approach with a cosh parametrized form nuclear potential [27-29], the density-dependent cluster model with a double-folding integral of the renormalized M3Y nucleon-nucleon potential [30-34], the preformed cluster model with SLy4 Skyrme-like effective interaction [9, 35, 36], and so on.
Unlike other models, the Generalized Liquid Drop Model (GLDM) has two major advantages: introducing the quasimolecular shape mechanism [37], which can describe the complex deformation process from the parent nucleus continuous transition to the appearance of a deep and narrow neck, finally resulting in two tangential fragments, and adding the proximity energy, including an accurate radius and mass asymmetry. When a neck or a gap appears in one-body shapes or between separated fragments, proximity energy plays a key role in taking into account the effects of the nuclear forces between the close surfaces, balancing the repulsion of the Coulomb barrier, and reasonably constructing the barrier heights and positions of the nucleus in complex deformation processes [37-40]. Therefore, the GLDM can successfully deal with proton radioactivity [41], cluster radioactivity [42], fusion [43], fission [44] and the α decay process [22, 37, 40, 45-48].
The proximity energy was first proposed by Blocki et al. [49] for describing the interaction energy associated with the crevice or neck in the nuclear configuration that would be expected immediately after contact of two nuclei in heavy-ion reactions. Therefore, it was also introduced into the GLDM by Royer [37] to take account of the effects of the nucleon–nucleon force inside the neck or the gap between the nascent or separated α-particle and daughter nucleus. The proximity energies are also used to study the fusion reaction cross sections and nuclear decay (including proton radioactivity, α decay, and cluster radioactivity), because these decay modes proceed in the opposite direction of fusion between a particle or cluster and the daughter nucleus [50, 51]. The proximity energy is based on the proximity force theorem [49, 52], which is described as the product of a factor depending on the mean curvature of the interaction surface and a universal function (depending on the separation distance) and is independent of the masses of colliding nuclei [53, 54]. In the past few decades, numerous works have been devoted to improving the original proximity energy (Prox. 77) [49], by either adopting a better form of the surface energy coefficients [55-62] or introducing an improved universal function or another nuclear radius parameterization [52, 53, 63-74].
In order to obtain more precise calculations of α decay half-lives for known nuclei and to enhance the prediction ability of α decay half-lives and the island of stability for superheavy nuclei, it is very important and interesting to develop the GLDM by adopting a more suitable proximity energy for constructing a reasonable potential barrier based on available α decay experimental data. This is the purpose of the present work. We also notice that there have been some works using the GLDM with proximity energy Prox. 81 [52] or proximity energy Denisov [73] instead of the original proximity energy formalism in the GLDM to study the α decay [75-78]. The calculations can reproduce the experimental data better than those calculated by the original GLDM. However, in the present work, we find that proximity energy Prox. 81 [52] is not the most suitable substitute for the original one, and the proximity energy Denisov [73] has a different nuclear radius formalism from that of the GLDM, which is not self-consistent for calculation. In this work, for self-consistency, we choose 16 various versions of proximity energies that have the same radii forms as the GLDM and systematically study the applicability of these when applied to the GLDM. The calculations indicate that GLDM with the proximity energy Prox. 77-Set 13 gives the lowest root-mean-square (RMS) deviation in reproducing experimental α half-lives. Using GLDM with proximity energy Prox. 77-Set 13, we predict α decay half-lives of superheavy even-even nuclei with
This article is organized as follows. In Sec. II, theoretical framework for the α decay half-life and the GLDM are briefly presented. The detailed calculations and discussion are given in Sec. III. Sec. IV presents a brief summary.
A.The generalized liquid drop model
The α decay half-life can be calculated with decay constant $T_{1/2} = \frac{\ln{2}}{\lambda} .$ ![]() | (1) |
$\lambda = P_{\alpha}{\nu}P .$ ![]() | (2) |
$\log_{10}P_{\alpha} = a+bA^{1/6}\sqrt{Z}+c\frac{Z}{\sqrt{Q_{\alpha}}}-d\chi^{\prime}-e\rho^{\prime}+f\sqrt{l(l+1)} ,$ ![]() | (3) |
The assault frequency
$ \nu = \frac{1}{2R_0}\sqrt{\frac{2E_{\alpha}}{M}} ,$ ![]() | (4) |
$R_{i} = 1.28A_{i}^{1/3}-0.76+0.8A_{i}^{-1/3}(i = 0,1,2) . $ ![]() | (5) |
$P = \exp\bigg[-\frac{2}{\hbar}{\int_{r_{{\rm{in}}}}^{r_{{\rm{out}}}} \sqrt{2B(r)(E_{r}-E({\rm{sphere}}))} {\rm d}r}\bigg] ,$ ![]() | (6) |
The total interaction potential
$E = E_{\rm V}+E_{\rm S}+E_{\rm C}+E_{{\rm{Prox}}}+E_{l} .$ ![]() | (7) |
$E_{\rm V} = -15.494(1-1.8I^2)A ,$ ![]() | (8) |
$E_{\rm S} = 17.9439(1-2.6I^2)A^{2/3}(S/4{\pi}R_0^2) ,$ ![]() | (9) |
$E_{\rm C} = 0.6e^2(Z^2/R_0)\times0.5\int(V(\theta)/V_0)(R(\theta)/R_0)^3\sin\theta{{\rm d}\theta} ,$ ![]() | (10) |
For two separated fragments, the volume, surface, and Coulomb energies are defined as
$E_{\rm V} = -15.494[(1-1.8I_1^2)A_1+(1-1.8I_2^2)A_2] ,$ ![]() | (11) |
$E_{\rm S} = 17.9439[(1-2.6I_1^2)A_1^{2/3}+(1-2.6I_2^2)A_2^{2/3}] ,$ ![]() | (12) |
$E_{\rm C} = 0.6e^2Z_1^2/R_1+0.6e^2Z_2^2/R_2+e^2Z_1Z_2/r ,$ ![]() | (13) |
The centrifugal barrier
$E_l(r) = \frac{{\hbar}^2l(l+1)}{2{\mu}r^2} .$ ![]() | (14) |
$l_{\min} = \left\{\begin{array}{llll} {\Delta}_j,&{\rm{for\;even}}\;{\Delta}_j\;{\rm{and}}\;{\pi}_p = {\pi}_d,\\ {\Delta}_j+1,&{\rm{for\;even}}\;{\Delta}_j\;{\rm{and}}\;{\pi}_p\ne{\pi}_d,\\ {\Delta}_j,&{\rm{for\;odd}}\;{\Delta}_j\;{\rm{and}}\;{\pi}_p\ne{\pi}_d,\\ {\Delta}_j+1,&{\rm{for\;odd}}\;{\Delta}_j\;{\rm{and}}\;{\pi}_p = {\pi}_d, \end{array}\right.$ ![]() | (15) |
2
B.The proximity energy
The surface energy comes from the effects of the surface tension forces in half-space. When a neck or a gap appears in one-body shapes or between separated fragments, an additional term called the proximity energy must be added to take into account the effects of the nuclear forces between the close surfaces [37, 40]. The proximity energy is described as the product of a factor depending on the mean curvature of the interaction surface and a universal function depending on the separation distance [53, 54].In the present work, for self-consistency, we choose 16 various versions of proximity energies that have the same radii forms as the GLDM, including proximity energy formalisms Prox. 77 [49] and its 12 modified forms on the basis of improving the surface energy coefficients [55-62], Bass 80 [69], Prox. 81 [52], and Guo 2013 [74]. Proximity energy Prox. 77 [49] and its 12 modified forms are expressed as
$E_{\rm{Prox}}(r) = 4\pi{\gamma}b\bar{R}\phi(\xi) ,$ ![]() | (16) |
$\bar{R} = \frac{C_1C_2}{C_1+C_2} ,$ ![]() | (17) |
$C_i = R_i\left[1-(\frac{b}{R_i})^2\right] (i = 1,2) ,$ ![]() | (18) |
The surface energy coefficient
$\gamma = \gamma_0(1-k_sA_s^2),$ ![]() | (19) |
Set 1:
Set 2:
Set 3:
Set 4:
Set 5:
Set 6:
Set 7:
Set 8:
Set 9:
Set 10:
Set 11:
Set 12:
Set 13:
The universal function
$\phi(\xi) = \left\{\begin{array}{ll} -\dfrac{1}{2}(\xi-\xi_0)^2-0.0852(\xi-\xi_0)^3,&0<\xi\leqslant{1.2511},\\ -3.347\exp\left(\dfrac{-\xi}{0.75}\right),&\xi\geqslant{1.2511}, \end{array}\right. $ ![]() | (20) |
If the improved versions of the original proximity energy can be applied to the GLDM, three conditions need to be met: first, the radii formulas for the proximity energy and GLDM should be the same; second, the total GLDM energy, including the proximity energy, between the α-particle and daughter nucleus should be reasonable; and finally, the calculated α decay half-lives by the GLDM with the best selected proximity energy should give the lowest RMS deviation in reproducing experimental α half-lives. Therefore, we comparatively study the abilities of 16 various versions of proximity energies when they are applied to the GLDM for describing the α decay half-lives, in which the proximity energies have the same radii forms as the GLDM. These proximity energies include Prox. 77 [49] and its 12 modified forms on the basis of improving the surface energy coefficients [55-62], Bass 80 [69], Prox. 81 [52], and Guo 2013 [74].
In these various versions of proximity energies, we find that the proximity energy Guo 2013 [74] is not suitable for application to the GLDM because for some α decay nuclei such as 148Gd, the total nuclear potential distribution shows short-term decline, even less than zero, after the two tangent fragments have separated, which results from the proximity energy determining too strong an attractive interaction potential.
For choosing the most suitable one from the remainder of proximity energies that can be applied to the GLDM, we calculate the RMS deviation between the calculated α decay half-lives by the GLDM with various proximity energies, where the α-particle preformation factor is assumed as the constant
$\sigma = \sqrt{\frac{1}{n}\sum \left({\log_{10}T_{1/2}^{{\rm{cal}}}-\log_{10}T_{1/2}^{{\rm{exp}}}}\right)^2} .$ ![]() | (21) |
GLDM with proximity energy | ![]() ![]() | GLDM with proximity energy | ![]() ![]() |
Prox. 77-Set 1 | 1.472 | Prox. 77-Set 2 | 1.488 |
Prox. 77-Set 3 | 1.579 | Prox. 77-Set 4 | 1.534 |
Prox. 77-Set 5 | 1.525 | Prox. 77-Set 6 | 1.546 |
Prox. 77-Set 7 | 1.541 | Prox. 77-Set 8 | 1.467 |
Prox. 77-Set 9 | 1.542 | Prox. 77-Set 10 | 1.543 |
Prox. 77-Set 11 | 1.505 | Prox. 77-Set 12 | 1.471 |
Prox. 77-Set 13 | 1.459 | Bass 80 | 1.644 |
Prox. 81 | 1.500 | original one | 1.605 |
Table1.The RMS deviations between calculated α decay half-lives by GLDM with different versions of proximity energies and experimental data.
The experimental data and calculations of α decay half-lives for 148Gd, as an example, are listed in Table 2. From this table, one can find that the GLDM with various proximity energies calculates different α decay half-lives. Further, all calculations are an order of magnitude smaller than the experimental data, indicating that the α-particle preformation factor is on the order of
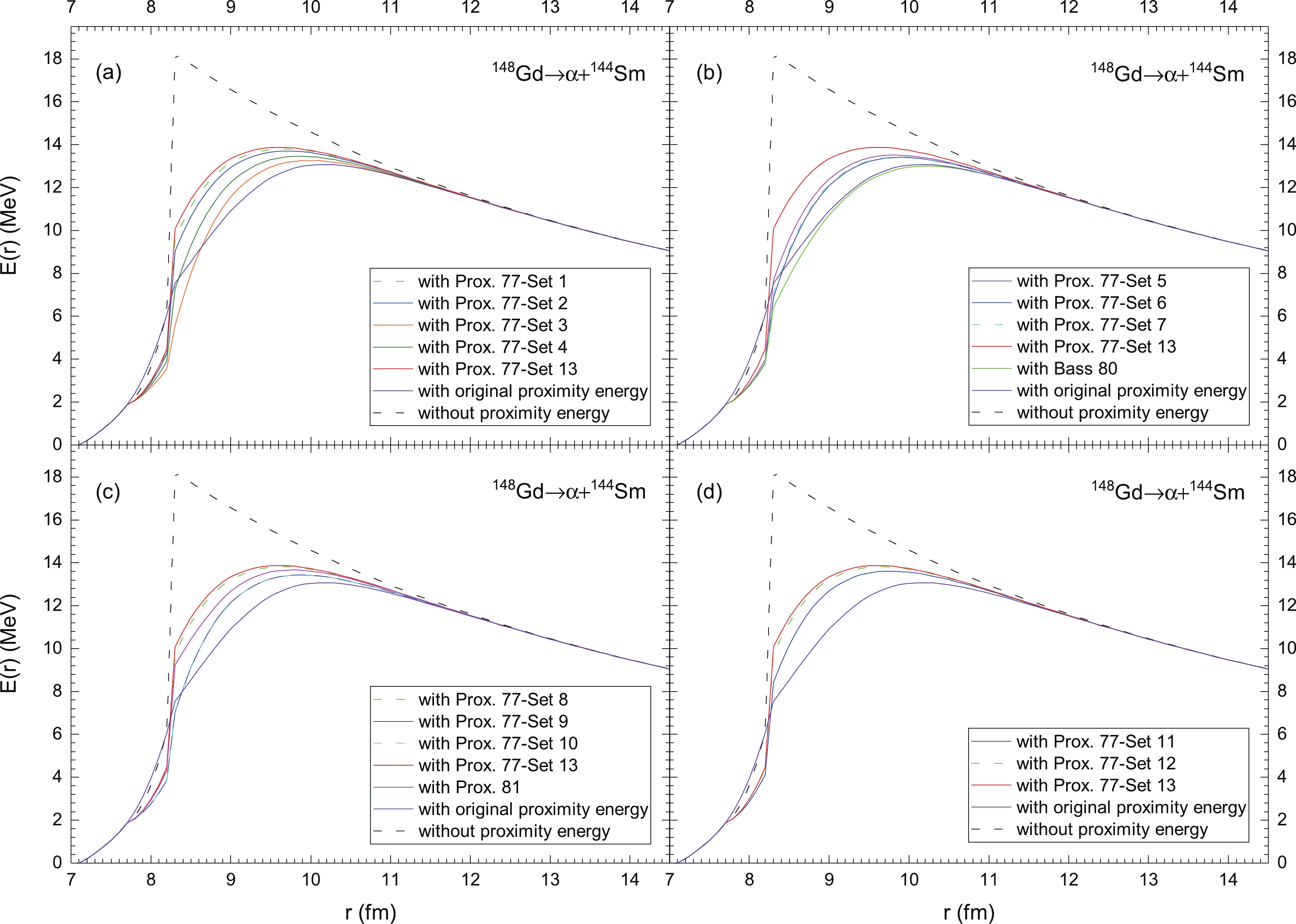
method | α decay half-lives for 148Gd/s |
experimental data | ![]() ![]() |
GLDM with original proximity energy | ![]() ![]() |
GLDM with Prox. 77- Set 1 | ![]() ![]() |
GLDM with Prox. 77- Set 2 | ![]() ![]() |
GLDM with Prox. 77- Set 3 | ![]() ![]() |
GLDM with Prox. 77- Set 4 | ![]() ![]() |
GLDM with Prox. 77- Set 5 | ![]() ![]() |
GLDM with Prox. 77- Set 6 | ![]() ![]() |
GLDM with Prox. 77- Set 7 | ![]() ![]() |
GLDM with Prox. 77- Set 8 | ![]() ![]() |
GLDM with Prox. 77- Set 9 | ![]() ![]() |
GLDM with Prox. 77- Set 10 | ![]() ![]() |
GLDM with Prox. 77- Set 11 | ![]() ![]() |
GLDM with Prox. 77- Set 12 | ![]() ![]() |
GLDM with Prox. 77- Set 13 | ![]() ![]() |
GLDM with Bass 80 | ![]() ![]() |
GLDM with Prox. 81 | ![]() ![]() |
Table2.The α decay half-lives of calculations by GLDM with different versions of proximity energies and experimental data for 148Gd.
The same values of
In our previous work [81], we proposed an analytic formula for estimating the α-particle preformation factor, i.e., Eq. (3). In this work, because we choose a more suitable proximity energy in the GLDM, the parameters of Eq. (3) should be redetermined. First, we extract the α-particle preformation factor using the ratio of the calculated α decay half-life within the GLDM with proximity energy Prox. 77-Set 13 and
nuclei | region | ![]() ![]() | ![]() ![]() | ![]() ![]() | ![]() ![]() | ![]() ![]() | ![]() ![]() |
even-even nuclei | ![]() ![]() | ?9.4985 | ?8.9005 | 4.0450 | 1.0432 | ?2.9731 | ? |
![]() ![]() | ?2.1047 | 0.1230 | 4.2051 | 1.0681 | 0.0533 | ? | |
odd-A nuclei | ![]() ![]() | ?24.5445 | ?13.2233 | 9.9493 | 2.5690 | ?4.5754 | ?0.0350 |
![]() ![]() | 1.3626 | ?6.2523 | ?0.0252 | ?0.0155 | ?1.9616 | ?0.0937 | |
doubly odd nuclei | ![]() ![]() | ?2.7484 | ?4.2572 | 2.2748 | 0.5947 | ?1.3917 | ?0.0901 |
![]() ![]() | ?37.5320 | ?20.0571 | 23.5391 | 6.0638 | ?6.9409 | ?0.2030 |
Table3.The parameters of Eq. (3) for estimating the α-particle preformation factor.
The calculated α decay half-lives and experimental data are listed in Tables 4-6 for even-even nuclei, odd-
α transition | ![]() ![]() | ![]() ![]() | ![]() ![]() | ![]() ![]() | ![]() ![]() | ![]() ![]() | ![]() ![]() | α transition | ![]() ![]() | ![]() ![]() | ![]() ![]() | ![]() ![]() | ![]() ![]() | ![]() ![]() | ![]() ![]() | |||
148Gd | 144Sm | 3.27 | 0 | 9.35 | 8.68 | 8.86 | 0.2841 | 9.41 | 150Gd | 146Sm | 2.81 | 0 | 13.75 | 13.17 | 13.17 | 0.3578 | 13.62 | |
150Dy | 146Gd | 4.35 | 0 | 3.07 | 2.17 | 2.21 | 0.1775 | 2.96 | 152Dy | 148Gd | 3.73 | 0 | 6.93 | 6.26 | 6.32 | 0.2117 | 6.99 | |
154Dy | 150Gd | 2.95 | 0 | 13.98 | 13.17 | 13.39 | 0.3075 | 13.9 | 152Er | 148Dy | 4.94 | 0 | 1.06 | 0.12 | 0.26 | 0.1635 | 1.05 | |
154Er | 150Dy | 4.28 | 0 | 4.68 | 3.72 | 3.95 | 0.1845 | 4.69 | 156Er | 152Dy | 3.48 | 0 | 10.24 | 9.49 | 9.61 | 0.2389 | 10.23 | |
154Yb | 150Er | 5.47 | 0 | ?0.35 | ?1.39 | ?1.15 | 0.1601 | ?0.36 | 156Yb | 152Er | 4.81 | 0 | 2.41 | 1.79 | 1.95 | 0.1725 | 2.71 | |
158Yb | 154Er | 4.17 | 0 | 6.63 | 5.52 | 5.55 | 0.1917 | 6.27 | 156Hf | 152Yb | 6.03 | 0 | ?1.63 | ?2.73 | ?2.55 | 0.1609 | ?1.75 | |
158Hf | 154Yb | 5.41 | 0 | 0.35 | ?0.18 | ?0.15 | 0.165 | 0.64 | 160Hf | 156Yb | 4.9 | 0 | 3.28 | 2.28 | 2.38 | 0.1672 | 3.15 | |
162Hf | 158Yb | 4.42 | 0 | 5.69 | 5.07 | 5.2 | 0.1719 | 5.96 | 158W | 154Hf | 6.62 | 0 | ?2.9 | ?4.04 | ?3.9 | 0.1645 | ?3.12 | |
160W | 156Hf | 6.07 | 0 | ?0.99 | ?2.05 | ?1.91 | 0.1616 | ?1.12 | 162W | 158Hf | 5.68 | 0 | 0.42 | ?0.46 | ?0.31 | 0.1549 | 0.5 | |
164W | 160Hf | 5.28 | 0 | 2.22 | 1.31 | 1.52 | 0.1501 | 2.34 | 166W | 162Hf | 4.86 | 0 | 4.74 | 3.49 | 3.58 | 0.1475 | 4.41 | |
168W | 164Hf | 4.5 | 0 | 6.2 | 5.57 | 5.71 | 0.1439 | 6.55 | 180W | 176Hf | 2.52 | 0 | 25.75 | 24.54 | 24.72 | 0.1572 | 25.52 | |
162Os | 158W | 6.77 | 0 | ?2.68 | ?3.76 | ?3.58 | 0.1623 | ?2.79 | 166Os | 162W | 6.14 | 0 | ?0.53 | ?1.55 | ?1.43 | 0.1412 | ?0.58 | |
168Os | 164W | 5.82 | 0 | 0.68 | ?0.23 | ?0.08 | 0.1327 | 0.79 | 170Os | 166W | 5.54 | 0 | 1.89 | 1.01 | 1.21 | 0.124 | 2.12 | |
172Os | 168W | 5.22 | 0 | 3.23 | 2.46 | 2.54 | 0.1171 | 3.48 | 174Os | 170W | 4.87 | 0 | 5.25 | 4.35 | 4.53 | 0.1122 | 5.48 | |
186Os | 182W | 2.82 | 0 | 22.8 | 21.72 | 21.89 | 0.1048 | 22.87 | 166Pt | 162Os | 7.29 | 0 | ?3.52 | ?4.73 | ?4.58 | 0.1518 | ?3.76 | |
168Pt | 164Os | 6.99 | 0 | ?2.69 | ?3.78 | ?3.65 | 0.14 | ?2.8 | 172Pt | 168Os | 6.46 | 0 | ?1 | ?2.02 | ?1.9 | 0.1185 | ?0.98 | |
174Pt | 170Os | 6.18 | 0 | 0.06 | ?0.95 | ?0.76 | 0.1096 | 0.2 | 176Pt | 172Os | 5.89 | 0 | 1.2 | 0.28 | 0.48 | 0.1019 | 1.47 | |
178Pt | 174Os | 5.57 | 0 | 2.43 | 1.63 | 1.71 | 0.0953 | 2.73 | 180Pt | 176Os | 5.24 | 0 | 4.27 | 3.28 | 3.47 | 0.0898 | 4.51 | |
182Pt | 178Os | 4.95 | 0 | 5.62 | 4.83 | 5.02 | 0.0842 | 6.09 | 184Pt | 180Os | 4.6 | 0 | 7.77 | 6.94 | 7.06 | 0.0803 | 8.15 | |
190Pt | 186Os | 3.27 | 0 | 19.31 | 17.86 | 17.98 | 0.0797 | 19.08 | 172Hg | 168Pt | 7.53 | 0 | ?3.64 | ?4.79 | ?4.65 | 0.1315 | ?3.77 | |
174Hg | 170Pt | 7.23 | 0 | ?2.7 | ?3.9 | ?3.7 | 0.1206 | ?2.78 | 176Hg | 172Pt | 6.9 | 0 | ?1.65 | ?2.79 | ?2.59 | 0.1114 | ?1.63 | |
178Hg | 174Pt | 6.58 | 0 | ?0.53 | ?1.69 | ?1.59 | 0.1029 | ?0.6 | 180Hg | 176Pt | 6.26 | 0 | 0.73 | ?0.47 | ?0.28 | 0.0952 | 0.74 | |
182Hg | 178Pt | 6 | 0 | 1.89 | 0.62 | 0.81 | 0.0876 | 1.87 | 184Hg | 180Pt | 5.66 | 0 | 3.44 | 2.12 | 2.23 | 0.0816 | 3.32 | |
186Hg | 182Pt | 5.2 | 0 | 5.7 | 4.37 | 4.55 | 0.0778 | 5.66 | 178Pb | 174Hg | 7.79 | 0 | ?3.64 | ?4.94 | ?4.8 | 0.114 | ?3.86 | |
180Pb | 176Hg | 7.42 | 0 | ?2.39 | ?3.81 | ?3.62 | 0.1049 | ?2.64 | 184Pb | 180Hg | 6.77 | 0 | ?0.21 | ?1.64 | ?1.53 | 0.0885 | ?0.48 | |
186Pb | 182Hg | 6.47 | 0 | 1.07 | ?0.54 | ?0.36 | 0.0813 | 0.73 | 188Pb | 184Hg | 6.11 | 0 | 2.43 | 0.94 | 1.13 | 0.0752 | 2.25 | |
190Pb | 186Hg | 5.7 | 0 | 4.24 | 2.81 | 2.93 | 0.0703 | 4.08 | 192Pb | 188Hg | 5.22 | 0 | 6.55 | 5.26 | 5.44 | 0.0664 | 6.62 | |
186Po | 182Pb | 8.5 | 0 | ?4.47 | ?6.36 | ?6.16 | 0.086 | ?5.09 | 190Po | 186Pb | 7.69 | 0 | ?2.61 | ?4.08 | ?3.98 | 0.0724 | ?2.84 | |
194Po | 190Pb | 6.99 | 0 | ?0.41 | ?1.79 | ?1.6 | 0.0608 | ?0.38 | 196Po | 192Pb | 6.66 | 0 | 0.75 | ?0.58 | ?0.47 | 0.0557 | 0.78 | |
198Po | 194Pb | 6.31 | 0 | 2.27 | 0.82 | 1 | 0.0512 | 2.29 | 200Po | 196Pb | 5.98 | 0 | 3.79 | 2.26 | 2.44 | 0.047 | 3.76 | |
202Po | 198Pb | 5.7 | 0 | 5.14 | 3.53 | 3.64 | 0.0431 | 5.01 | 204Po | 200Pb | 5.49 | 0 | 6.27 | 4.61 | 4.77 | 0.0393 | 6.18 | |
206Po | 202Pb | 5.33 | 0 | 7.14 | 5.44 | 5.61 | 0.0357 | 7.06 | 208Po | 204Pb | 5.22 | 0 | 7.96 | 6.06 | 6.17 | 0.0323 | 7.66 | |
212Po | 208Pb | 8.95 | 0 | ?6.53 | ?8 | ?7.81 | 0.1047 | ?6.83 | 214Po | 210Pb | 7.83 | 0 | ?3.79 | ?4.94 | ?4.75 | 0.1419 | ?3.91 | |
216Po | 212Pb | 6.91 | 0 | ?0.84 | ?1.86 | ?1.69 | 0.1921 | ?0.97 | 218Po | 214Pb | 6.12 | 0 | 2.27 | 1.28 | 1.45 | 0.2616 | 2.03 | |
194Rn | 190Po | 7.86 | 0 | ?3.11 | ?3.9 | ?3.72 | 0.0708 | ?2.57 | 196Rn | 192Po | 7.62 | 0 | ?2.33 | ?3.16 | ?3.08 | 0.0641 | ?1.89 | |
200Rn | 196Po | 7.04 | 0 | 0.07 | ?1.22 | ?1.04 | 0.053 | 0.23 | 202Rn | 198Po | 6.77 | 0 | 1.09 | ?0.27 | ?0.16 | 0.0482 | 1.15 | |
204Rn | 200Po | 6.55 | 0 | 2.01 | 0.61 | 0.78 | 0.0438 | 2.14 | 206Rn | 202Po | 6.38 | 0 | 2.74 | 1.27 | 1.45 | 0.0396 | 2.85 | |
208Rn | 204Po | 6.26 | 0 | 3.37 | 1.79 | 1.91 | 0.0358 | 3.35 | 210Rn | 206Po | 6.16 | 0 | 3.95 | 2.16 | 2.32 | 0.0323 | 3.81 | |
212Rn | 208Po | 6.38 | 0 | 3.16 | 1.15 | 1.31 | 0.0287 | 2.86 | 214Rn | 210Po | 9.21 | 0 | ?6.57 | ?7.97 | ?7.77 | 0.0891 | ?6.72 | |
Continued on next page |
Table4.Calculations of α decay half-lives for even-even nuclei. Experimental α decay half-lives are taken from the latest evaluated nuclear properties table NUBASE2016 [83]. The α decay energies are taken from the latest evaluated atomic mass table AME2016 [84, 85]. The α decay energies and half-lives are in units of MeV and s, respectively.
Table 4-continued from previous page | ||||||||||||||||||
α transition | ![]() ![]() | ![]() ![]() | ![]() ![]() | ![]() ![]() | ![]() ![]() | ![]() ![]() | ![]() ![]() | α transition | ![]() ![]() | ![]() ![]() | ![]() ![]() | ![]() ![]() | ![]() ![]() | ![]() ![]() | ![]() ![]() | |||
216Rn | 212Po | 8.2 | 0 | ?4.35 | ?5.3 | ?5.13 | 0.1146 | ?4.19 | 218Rn | 214Po | 7.26 | 0 | ?1.47 | ?2.37 | ?2.2 | 0.1515 | ?1.38 | |
220Rn | 216Po | 6.41 | 0 | 1.75 | 0.92 | 1.09 | 0.206 | 1.78 | 222Rn | 218Po | 5.59 | 0 | 5.52 | 4.76 | 4.91 | 0.2943 | 5.44 | |
202Ra | 198Rn | 7.88 | 0 | ?2.39 | ?3.34 | ?3.24 | 0.0557 | ?1.98 | 204Ra | 200Rn | 7.64 | 0 | ?1.22 | ?2.58 | ?2.41 | 0.0504 | ?1.11 | |
208Ra | 204Rn | 7.27 | 0 | 0.1 | ?1.37 | ?1.26 | 0.0412 | 0.13 | 214Ra | 210Rn | 7.27 | 0 | 0.39 | ?1.49 | ?1.31 | 0.03 | 0.21 | |
216Ra | 212Rn | 9.53 | 0 | ?6.74 | ?8.08 | ?7.9 | 0.075 | ?6.77 | 218Ra | 214Rn | 8.55 | 0 | ?4.6 | ?5.61 | ?5.44 | 0.094 | ?4.41 | |
220Ra | 216Rn | 7.59 | 0 | ?1.75 | ?2.71 | ?2.54 | 0.1221 | ?1.63 | 222Ra | 218Rn | 6.68 | 0 | 1.53 | 0.65 | 0.81 | 0.1653 | 1.59 | |
224Ra | 220Rn | 5.79 | 0 | 5.5 | 4.7 | 4.85 | 0.2376 | 5.48 | 226Ra | 222Rn | 4.87 | 0 | 10.7 | 10.04 | 10.2 | 0.3838 | 10.61 | |
208Th | 204Ra | 8.2 | 0 | ?2.62 | ?3.65 | ?3.54 | 0.0485 | ?2.22 | 212Th | 208Ra | 7.96 | 0 | ?1.5 | ?2.98 | ?2.81 | 0.0394 | ?1.41 | |
214Th | 210Ra | 7.83 | 0 | ?1.06 | ?2.58 | ?2.41 | 0.0355 | ?0.96 | 216Th | 212Ra | 8.07 | 0 | ?1.59 | ?3.41 | ?3.24 | 0.0319 | ?1.75 | |
218Th | 214Ra | 9.85 | 0 | ?6.93 | ?8.21 | ?8.03 | 0.0634 | ?6.83 | 220Th | 216Ra | 8.95 | 0 | ?5.01 | ?6.04 | ?5.86 | 0.0765 | ?4.74 | |
222Th | 218Ra | 8.13 | 0 | ?2.65 | ?3.69 | ?3.52 | 0.0934 | ?2.5 | 224Th | 220Ra | 7.3 | 0 | 0.02 | ?0.93 | ?0.76 | 0.1182 | 0.16 | |
226Th | 222Ra | 6.45 | 0 | 3.27 | 2.46 | 2.62 | 0.1578 | 3.42 | 228Th | 224Ra | 5.52 | 0 | 7.78 | 7.04 | 7.15 | 0.2354 | 7.77 | |
230Th | 226Ra | 4.77 | 0 | 12.38 | 11.74 | 11.89 | 0.3509 | 12.34 | 216U | 212Th | 8.53 | 0 | ?2.16 | ?4.05 | ?3.89 | 0.0382 | ?2.47 | |
218U | 214Th | 8.78 | 0 | ?3.26 | ?4.81 | ?4.64 | 0.0344 | ?3.17 | 222U | 218Th | 9.48 | 0 | ?5.33 | ?6.74 | ?6.57 | 0.0612 | ?5.36 | |
224U | 220Th | 8.63 | 0 | ?3.4 | ?4.48 | ?4.31 | 0.0736 | ?3.18 | 226U | 222Th | 7.7 | 0 | ?0.57 | ?1.56 | ?1.4 | 0.0935 | ?0.37 | |
230U | 226Th | 5.99 | 0 | 6.24 | 5.48 | 5.63 | 0.1673 | 6.4 | 232U | 228Th | 5.41 | 0 | 9.34 | 8.64 | 8.79 | 0.2148 | 9.46 | |
234U | 230Th | 4.86 | 0 | 12.89 | 12.22 | 12.37 | 0.2849 | 12.91 | 236U | 232Th | 4.57 | 0 | 14.87 | 14.21 | 14.35 | 0.3309 | 14.83 | |
228Pu | 224U | 7.94 | 0 | 0.32 | ?1.65 | ?1.54 | 0.0785 | ?0.44 | 230Pu | 226U | 7.18 | 0 | 2.01 | 1.08 | 1.23 | 0.0971 | 2.24 | |
232Pu | 228U | 6.72 | 0 | 4.24 | 2.98 | 3.13 | 0.1114 | 4.08 | 234Pu | 230U | 6.31 | 0 | 5.72 | 4.82 | 4.97 | 0.1269 | 5.87 | |
236Pu | 232U | 5.87 | 0 | 7.96 | 6.98 | 7.12 | 0.1491 | 7.95 | 238Pu | 234U | 5.59 | 0 | 9.44 | 8.49 | 8.63 | 0.1647 | 9.41 | |
240Pu | 236U | 5.26 | 0 | 11.32 | 10.52 | 10.66 | 0.1897 | 11.38 | 242Pu | 238U | 4.98 | 0 | 13.07 | 12.3 | 12.45 | 0.2137 | 13.12 | |
244Pu | 240U | 4.67 | 0 | 15.4 | 14.6 | 14.73 | 0.2508 | 15.33 | 234Cm | 230Pu | 7.37 | 0 | 2.28 | 1.15 | 1.31 | 0.08 | 2.41 | |
236Cm | 232Pu | 7.07 | 0 | 3.35 | 2.26 | 2.41 | 0.0859 | 3.47 | 238Cm | 234Pu | 6.67 | 0 | 5.31 | 3.95 | 4.1 | 0.0962 | 5.11 | |
240Cm | 236Pu | 6.4 | 0 | 6.37 | 5.2 | 5.35 | 0.1037 | 6.33 | 242Cm | 238Pu | 6.22 | 0 | 7.15 | 6.08 | 6.23 | 0.1085 | 7.19 | |
244Cm | 240Pu | 5.9 | 0 | 8.76 | 7.7 | 7.84 | 0.1204 | 8.76 | 246Cm | 242Pu | 5.48 | 0 | 11.17 | 10.07 | 10.2 | 0.1424 | 11.05 | |
248Cm | 244Pu | 5.16 | 0 | 13.08 | 12.05 | 12.19 | 0.1621 | 12.98 | 238Cf | 234Cm | 8.13 | 0 | 1.02 | ?0.91 | ?0.76 | 0.0562 | 0.49 | |
240Cf | 236Cm | 7.71 | 0 | 1.61 | 0.55 | 0.7 | 0.0616 | 1.91 | 242Cf | 238Cm | 7.52 | 0 | 2.42 | 1.26 | 1.41 | 0.0636 | 2.61 | |
244Cf | 240Cm | 7.33 | 0 | 3.07 | 1.96 | 2.11 | 0.0657 | 3.29 | 246Cf | 242Cm | 6.86 | 0 | 5.11 | 3.84 | 3.99 | 0.0745 | 5.11 | |
248Cf | 244Cm | 6.36 | 0 | 7.46 | 6.16 | 6.31 | 0.0869 | 7.37 | 250Cf | 246Cm | 6.13 | 0 | 8.62 | 7.33 | 7.46 | 0.0927 | 8.49 | |
252Cf | 248Cm | 6.22 | 0 | 7.94 | 6.85 | 6.99 | 0.0872 | 8.05 | 254Cf | 250Cm | 5.93 | 0 | 9.22 | 8.31 | 8.45 | 0.0955 | 9.47 | |
244Fm | 240Cf | 8.55 | 0 | ?0.11 | ?1.63 | ?1.48 | 0.0434 | ?0.12 | 248Fm | 244Cf | 8 | 0 | 1.56 | 0.18 | 0.33 | 0.0477 | 1.65 | |
252Fm | 248Cf | 7.15 | 0 | 4.96 | 3.39 | 3.53 | 0.0578 | 4.77 | 254Fm | 250Cf | 7.31 | 0 | 4.07 | 2.67 | 2.81 | 0.0539 | 4.08 | |
256Fm | 252Cf | 7.03 | 0 | 5.07 | 3.83 | 3.98 | 0.0573 | 5.22 | 254No | 250Fm | 8.23 | 0 | 1.75 | 0.03 | 0.17 | 0.0387 | 1.58 | |
256No | 252Fm | 8.58 | 0 | 0.46 | ?1.18 | ?1.04 | 0.0347 | 0.42 | 258No | 254Fm | 8.15 | 0 | 2.08 | 0.24 | 0.37 | 0.0376 | 1.8 | |
256Rf | 252No | 8.93 | 0 | 0.32 | ?1.55 | ?1.4 | 0.0297 | 0.13 | 258Rf | 254No | 9.19 | 0 | ?0.98 | ?2.4 | ?2.26 | 0.0275 | ?0.7 | |
260Rf | 256No | 8.9 | 0 | 0.02 | ?1.53 | ?1.4 | 0.0286 | 0.15 | 260Sg | 256Rf | 9.9 | 0 | ?1.91 | ?3.77 | ?3.63 | 0.0218 | ?1.96 | |
264Hs | 260Sg | 10.59 | 0 | ?2.97 | ?4.97 | ?4.82 | 0.0173 | ?3.06 | 268Hs | 264Sg | 9.63 | 0 | 0.15 | ?2.44 | ?2.3 | 0.0195 | ?0.59 | |
270Hs | 266Sg | 9.07 | 0 | 0.95 | ?0.75 | ?0.62 | 0.0213 | 1.05 | 270Ds | 266Hs | 11.12 | 0 | ?3.69 | ?5.69 | ?5.55 | 0.014 | ?3.69 | |
286Fl | 282Cn | 10.37 | 0 | ?0.46 | ?2.8 | ?2.67 | 0.0115 | ?0.73 | 288Fl | 284Cn | 10.07 | 0 | ?0.12 | ?1.99 | ?1.87 | 0.0118 | 0.05 | |
290Lv | 286Fl | 11.01 | 0 | ?2.1 | ?3.92 | ?3.79 | 0.0094 | ?1.77 | 292Lv | 288Fl | 10.78 | 0 | ?1.62 | ?3.36 | ?3.23 | 0.0095 | ?1.21 | |
294Og | 290Lv | 11.84 | 0 | ?2.94 | ?5.36 | ?5.23 | 0.0076 | ?3.11 |
α transition | ![]() ![]() | ![]() ![]() | ![]() ![]() | ![]() ![]() | ![]() ![]() | ![]() ![]() | ![]() ![]() | α transition | ![]() ![]() | ![]() ![]() | ![]() ![]() | ![]() ![]() | ![]() ![]() | ![]() ![]() | ![]() ![]() | |||
149Tb | 145Eu | 4.08 | 2 | 4.95 | 3.68 | 3.8 | 0.0841 | 4.88 | 151Tb | 147Eu | 3.5 | 2 | 8.82 | 7.76 | 7.79 | 0.1413 | 8.64 | |
151Dy | 147Gd | 4.18 | 0 | 4.28 | 3.19 | 3.25 | 0.0986 | 4.25 | 153Dy | 149Gd | 3.56 | 0 | 8.39 | 7.54 | 7.67 | 0.1652 | 8.45 | |
151Ho | 147Tbm | 4.64 | 0 | 2.2 | 1.09 | 1.18 | 0.0838 | 2.25 | 151Hom | 147Tb | 4.74 | 0 | 1.79 | 0.6 | 0.7 | 0.0774 | 1.81 | |
153Hom | 149Tb | 4.12 | 0 | 5.47 | 4.14 | 4.27 | 0.1119 | 5.23 | 153Er | 149Dy | 4.8 | 0 | 1.84 | 0.78 | 0.91 | 0.0796 | 2 | |
155Er | 151Dy | 4.12 | 0 | 6.15 | 4.74 | 4.86 | 0.119 | 5.79 | 153Tm | 149Ho | 5.25 | 0 | 0.21 | ?0.87 | ?0.75 | 0.076 | 0.37 | |
153Tmm | 149Hom | 5.24 | 0 | 0.43 | ?0.85 | ?0.72 | 0.0763 | 0.39 | 155Tm | 151Ho | 4.57 | 0 | 3.38 | 2.55 | 2.69 | 0.1032 | 3.67 | |
155Yb | 151Er | 5.34 | 0 | 0.3 | ?0.8 | ?0.65 | 0.0782 | 0.46 | 157Yb | 153Er | 4.62 | 0 | 3.89 | 2.76 | 2.81 | 0.1057 | 3.78 | |
155Lu | 151Tm | 5.8 | 0 | ?1.12 | ?2.3 | ?2.14 | 0.0789 | ?1.04 | 155Lum | 151Tmm | 5.73 | 0 | ?0.74 | ?2 | ?1.84 | 0.0821 | ?0.76 | |
155Lun | 151Tm | 7.58 | 8 | ?2.57 | ?4.48 | ?4.29 | 0.0181 | ?2.55 | 157Lum | 153Tm | 5.13 | 0 | 1.89 | 0.64 | 0.72 | 0.0967 | 1.73 | |
157Hf | 153Yb | 5.89 | 0 | ?0.91 | ?2.22 | ?2.08 | 0.0827 | ?0.99 | 157Tan | 153Lu | 7.95 | 8 | ?2.77 | ?4.75 | ?4.46 | 0.0246 | ?2.85 | |
159Ta | 155Lum | 5.66 | 0 | 0.48 | ?0.83 | ?0.6 | 0.1006 | 0.39 | 159Tam | 155Lu | 5.75 | 0 | 0.01 | ?1.19 | ?0.97 | 0.0964 | 0.05 | |
159W | 155Hf | 6.45 | 0 | ?2 | ?3.46 | ?3.23 | 0.0926 | ?2.2 | 161W | 157Hf | 5.92 | 0 | ?0.25 | ?1.46 | ?1.31 | 0.0963 | ?0.29 | |
163W | 159Hf | 5.52 | 0 | 1.27 | 0.24 | 0.4 | 0.0961 | 1.42 | 159Rem | 155Ta | 6.97 | 0 | ?3.54 | ?4.8 | ?4.56 | 0.1005 | ?3.57 | |
161Rem | 157Tam | 6.43 | 0 | ?1.8 | ?2.97 | ?2.82 | 0.1018 | ?1.82 | 163Re | 159Ta | 6.01 | 0 | 0.08 | ?1.41 | ?1.24 | 0.0998 | ?0.24 | |
163Rem | 159Tam | 6.07 | 0 | ?0.49 | ?1.63 | ?1.46 | 0.0975 | ?0.45 | 165Re | 161Ta | 5.69 | 0 | 1.25 | ?0.13 | 0.08 | 0.0949 | 1.1 | |
165Rem | 161Tam | 5.66 | 0 | 1.12 | 0.02 | 0.22 | 0.0963 | 1.24 | 167Rem | 163Ta | 5.41 | 0 | 2.77 | 1.17 | 1.29 | 0.0898 | 2.34 | |
169Re | 165Ta | 5.01 | 3 | 5.18 | 3.79 | 3.99 | 0.0681 | 5.15 | 169Rem | 165Tam | 5.16 | 3 | 3.88 | 3 | 3.19 | 0.0632 | 4.39 | |
161Os | 157W | 7.07 | 0 | ?3.19 | ?4.74 | ?4.57 | 0.1068 | ?3.6 | 163Os | 159W | 6.69 | 0 | ?2.26 | ?3.5 | ?3.31 | 0.101 | ?2.32 | |
165Os | 161W | 6.34 | 0 | ?1.1 | ?2.28 | ?2.08 | 0.0951 | ?1.05 | 167Os | 163W | 5.99 | 0 | 0.21 | ?0.93 | ?0.79 | 0.0903 | 0.25 | |
169Os | 165W | 5.71 | 0 | 1.4 | 0.21 | 0.42 | 0.0837 | 1.49 | 165Irm | 161Rem | 6.89 | 0 | ?2.57 | ?3.82 | ?3.6 | 0.1031 | ?2.62 | |
167Ir | 163Re | 6.51 | 0 | ?1.17 | ?2.51 | ?2.36 | 0.0971 | ?1.35 | 167Irm | 163Rem | 6.56 | 0 | ?1.55 | ?2.71 | ?2.56 | 0.0954 | ?1.54 | |
169Ir | 165Re | 6.14 | 0 | ?0.18 | ?1.13 | ?0.92 | 0.0916 | 0.11 | 169Irm | 165Rem | 6.27 | 0 | ?0.45 | ?1.63 | ?1.42 | 0.0877 | ?0.37 | |
171Ir | 167Rem | 5.87 | 0 | 1.31 | ?0.05 | 0.06 | 0.0842 | 1.14 | 171Irm | 167Re | 6.16 | 2 | 0.43 | ?0.94 | ?0.84 | 0.0625 | 0.37 | |
173Irm | 169Re | 5.94 | 2 | 1.26 | ?0.09 | 0.04 | 0.0562 | 1.29 | 175Ir | 171Re | 5.43 | 2 | 3.02 | 2.23 | 2.42 | 0.0567 | 3.67 | |
177Ir | 173Re | 5.08 | 0 | 4.69 | 3.67 | 3.79 | 0.0664 | 4.97 | 167Pt | 163Os | 7.16 | 0 | ?3.1 | ?4.33 | ?4.17 | 0.104 | ?3.18 | |
171Pt | 167Os | 6.61 | 0 | ?1.3 | ?2.54 | ?2.43 | 0.0851 | ?1.36 | 173Pt | 169Os | 6.36 | 0 | ?0.35 | ?1.64 | ?1.5 | 0.0767 | ?0.38 | |
175Pt | 171Os | 6.16 | 2 | 0.58 | ?0.57 | ?0.38 | 0.056 | 0.87 | 177Pt | 173Os | 5.64 | 0 | 2.27 | 1.36 | 1.48 | 0.0676 | 2.65 | |
179Pt | 175Os | 5.41 | 2 | 3.94 | 2.72 | 2.81 | 0.0503 | 4.11 | 181Pt | 177Os | 5.15 | 0 | 4.85 | 3.74 | 3.93 | 0.0564 | 5.17 | |
183Pt | 179Os | 4.82 | 0 | 6.61 | 5.57 | 5.69 | 0.0535 | 6.96 | 171Aum | 167Irm | 7.16 | 0 | ?2.76 | ?4.04 | ?3.95 | 0.0947 | ?2.93 | |
173Au | 169Ir | 6.84 | 0 | ?1.53 | ?2.96 | ?2.82 | 0.0863 | ?1.75 | 173Aum | 169Irm | 6.9 | 0 | ?1.86 | ?3.17 | ?3.02 | 0.085 | ?1.95 | |
175Au | 171Ir | 6.59 | 0 | ?0.64 | ?2.08 | ?1.88 | 0.0773 | ?0.77 | 175Aum | 171Irm | 6.59 | 0 | ?0.75 | ?2.08 | ?1.88 | 0.0773 | ?0.77 | |
177Au | 173Ir | 6.3 | 0 | 0.56 | ?1 | ?0.89 | 0.0702 | 0.26 | 177Aum | 173Irm | 6.26 | 0 | 0.25 | ?0.85 | ?0.74 | 0.0709 | 0.41 | |
179Au | 175Ir | 5.98 | 1 | 1.51 | 0.35 | 0.44 | 0.0575 | 1.68 | 181Au | 177Ir | 5.75 | 2 | 2.7 | 1.56 | 1.75 | 0.0475 | 3.07 | |
183Au | 179Ir | 5.47 | 0 | 3.89 | 2.59 | 2.7 | 0.053 | 3.98 | 185Au | 181Ir | 5.18 | 0 | 4.98 | 4.05 | 4.23 | 0.0488 | 5.54 | |
171Hg | 167Pt | 7.67 | 2 | ?4.15 | ?4.9 | ?4.8 | 0.0898 | ?3.75 | 173Hg | 169Pt | 7.38 | 2 | ?3.1 | ?4.04 | ?3.88 | 0.0804 | ?2.79 | |
177Hg | 173Pt | 6.74 | 2 | ?0.82 | ?1.92 | ?1.82 | 0.0657 | ?0.64 | 179Hg | 175Pt | 6.36 | 0 | 0.14 | ?0.86 | ?0.76 | 0.0737 | 0.37 | |
181Hg | 177Pt | 6.28 | 2 | 1.12 | ?0.27 | ?0.09 | 0.0519 | 1.2 | 183Hg | 179Pt | 6.04 | 0 | 1.9 | 0.42 | 0.53 | 0.0566 | 1.78 | |
185Hg | 181Pt | 5.77 | 0 | 2.91 | 1.58 | 1.77 | 0.0511 | 3.06 | 177Tl | 173Au | 7.07 | 0 | ?1.61 | ?2.97 | ?2.88 | 0.0951 | ?1.86 | |
177Tlm | 173Aum | 7.66 | 0 | ?3.44 | ?4.91 | ?4.78 | 0.0849 | ?3.71 | 179Tl | 175Au | 6.71 | 0 | ?0.36 | ?1.75 | ?1.64 | 0.0863 | ?0.57 | |
179Tlm | 175Aum | 7.38 | 0 | ?2.85 | ?4.07 | ?3.93 | 0.0756 | ?2.81 | 181Tlm | 177Aum | 6.97 | 2 | ?0.46 | ?2.42 | ?2.23 | 0.0567 | ?0.99 | |
Continued on next page |
Table5.Same as Table 4, but for α decay of odd-A nuclei. Elements with superscript "m," "n," "p," or "x" indicate assignments to excited isomeric states (defined as higher states with half-lives greater than 100 ns). Elements with superscript "p" also indicate non-isomeric levels but are used in AME2016 [84, 85].
Table 5-continued from previous page | ||||||||||||||||||
α transition | ![]() ![]() | ![]() ![]() | ![]() ![]() | ![]() ![]() | ![]() ![]() | ![]() ![]() | ![]() ![]() | α transition | ![]() ![]() | ![]() ![]() | ![]() ![]() | ![]() ![]() | ![]() ![]() | ![]() ![]() | ![]() ![]() | |||
183Tl | 179Au | 5.98 | 0 | 2.54 | 1.16 | 1.28 | 0.072 | 2.42 | 183Tlm | 179Au | 6.61 | 3 | 0.54 | ?0.83 | ?0.72 | 0.0474 | 0.6 | |
187Tlm | 183Au | 5.66 | 2 | 4 | 2.87 | 3.05 | 0.0453 | 4.39 | 179Pb | 175Hg | 7.6 | 2 | ?2.41 | ?4.06 | ?3.93 | 0.0764 | ?2.81 | |
183Pbm | 179Hg | 7.02 | 3 | ?0.38 | ?1.93 | ?1.83 | 0.0555 | ?0.58 | 185Pbm | 181Hgm | 6.56 | 0 | 0.91 | ?0.82 | ?0.63 | 0.0674 | 0.54 | |
187Pb | 183Hg | 6.39 | 2 | 2.2 | 0.06 | 0.24 | 0.0482 | 1.56 | 187Pbm | 183Hgm | 6.21 | 0 | 2.18 | 0.53 | 0.71 | 0.0609 | 1.93 | |
189Pb | 185Hg | 5.92 | 2 | 3.99 | 2.1 | 2.21 | 0.0447 | 3.56 | 191Pbm | 187Hgm | 5.4 | 0 | 5.82 | 4.29 | 4.47 | 0.0512 | 5.76 | |
187Bi | 183Tl | 7.78 | 5 | ?1.43 | ?3.24 | ?3.07 | 0.0385 | ?1.65 | 187Bim | 183Tl | 7.89 | 0 | ?3.43 | ?5.02 | ?4.82 | 0.0591 | ?3.59 | |
189Bi | 185Tl | 7.27 | 5 | ?0.18 | ?1.64 | ?1.54 | 0.0351 | ?0.09 | 191Bi | 187Tlm | 6.45 | 0 | 1.36 | ?0.07 | 0.12 | 0.0522 | 1.4 | |
191Bim | 187Tl | 7.02 | 0 | ?0.78 | ?2.26 | ?2.08 | 0.048 | ?0.76 | 193Bi | 189Tlm | 6.02 | 0 | 3.26 | 1.68 | 1.86 | 0.0474 | 3.19 | |
193Bim | 189Tl | 6.61 | 0 | 0.56 | ?0.8 | ?0.61 | 0.0433 | 0.75 | 195Bi | 191Tlm | 5.54 | 0 | 5.76 | 4.01 | 4.19 | 0.0436 | 5.55 | |
195Bim | 191Tl | 6.23 | 0 | 2.42 | 0.73 | 0.92 | 0.0389 | 2.33 | 209Bi | 205Tl | 3.14 | 5 | 26.8 | 24.29 | 24.43 | 0.0145 | 26.27 | |
211Bi | 207Tl | 6.75 | 5 | 2.11 | ?0.21 | ?0.05 | 0.0287 | 1.49 | 213Bi | 209Tl | 5.99 | 5 | 5.12 | 2.96 | 3.12 | 0.031 | 4.63 | |
187Po | 183Pb | 7.98 | 2 | ?2.85 | ?4.61 | ?4.42 | 0.061 | ?3.21 | 189Po | 185Pb | 7.69 | 2 | ?2.42 | ?3.78 | ?3.68 | 0.0536 | ?2.41 | |
195Po | 191Pb | 6.75 | 0 | 0.69 | ?0.89 | ?0.71 | 0.0451 | 0.64 | 195Pom | 191Pbm | 6.84 | 0 | 0.33 | ?1.26 | ?1.07 | 0.0446 | 0.28 | |
197Po | 193Pb | 6.41 | 0 | 2.08 | 0.43 | 0.61 | 0.04 | 2.01 | 197Pom | 193Pbm | 6.51 | 0 | 1.48 | 0.02 | 0.2 | 0.0395 | 1.6 | |
199Po | 195Pb | 6.08 | 0 | 3.64 | 1.84 | 2.02 | 0.0355 | 3.47 | 199Pom | 195Pbm | 6.18 | 0 | 3.02 | 1.36 | 1.53 | 0.035 | 2.99 | |
201Po | 197Pb | 5.8 | 0 | 4.92 | 3.05 | 3.23 | 0.0313 | 4.74 | 201Pom | 197Pbm | 5.9 | 0 | 4.34 | 2.55 | 2.73 | 0.0309 | 4.24 | |
203Po | 199Pb | 5.5 | 2 | 6.29 | 4.86 | 5.02 | 0.0227 | 6.66 | 203Pom | 199Pb | 6.14 | 5 | 5.05 | 2.92 | 3.07 | 0.0165 | 4.85 | |
205Po | 201Pb | 5.33 | 0 | 7.18 | 5.46 | 5.63 | 0.0241 | 7.25 | 207Po | 203Pb | 5.22 | 0 | 7.99 | 6.06 | 6.23 | 0.0208 | 7.91 | |
209Po | 205Pbm | 4.98 | 0 | 9.59 | 7.46 | 7.62 | 0.0183 | 9.36 | 211Pom | 207Pb | 9.06 | 13 | 1.4 | ?0.66 | ?0.53 | 0.0037 | 1.9 | |
213Po | 209Pb | 8.54 | 0 | ?5.43 | ?6.94 | ?6.75 | 0.0666 | ?5.57 | 215Po | 211Pb | 7.53 | 0 | ?2.75 | ?3.99 | ?3.84 | 0.0714 | ?2.69 | |
219Po | 215Pb | 5.92 | 0 | 3.34 | 2.19 | 2.35 | 0.0834 | 3.43 | 191At | 187Bim | 7.71 | 0 | ?2.68 | ?3.77 | ?3.58 | 0.0701 | ?2.42 | |
191Atm | 187Bi | 7.88 | 2 | ?2.66 | ?4 | ?3.81 | 0.0566 | ?2.56 | 193At | 189Bim | 7.39 | 0 | ?1.54 | ?2.76 | ?2.58 | 0.0616 | ?1.37 | |
193Atm | 189Bi | 7.58 | 2 | ?1.68 | ?3.11 | ?2.92 | 0.0497 | ?1.62 | 193Atn | 189Bi | 7.62 | 3 | ?0.93 | ?2.93 | ?2.75 | 0.0456 | ?1.41 | |
195At | 191Bim | 7.1 | 0 | ?0.54 | ?1.79 | ?1.6 | 0.054 | ?0.33 | 197At | 193Bi | 7.11 | 0 | ?0.39 | ?1.84 | ?1.66 | 0.0461 | ?0.32 | |
197Atm | 193Bim | 6.84 | 0 | 0.3 | ?0.87 | ?0.69 | 0.0472 | 0.63 | 199At | 195Bi | 6.78 | 0 | 0.89 | ?0.64 | ?0.46 | 0.0405 | 0.93 | |
199Atm | 195Bi | 7.02 | 5 | 1.44 | ?0.12 | 0.04 | 0.0255 | 1.64 | 201At | 197Bi | 6.47 | 0 | 2.07 | 0.51 | 0.69 | 0.0356 | 2.14 | |
203At | 199Bi | 6.21 | 0 | 3.15 | 1.6 | 1.76 | 0.0312 | 3.27 | 205At | 201Bi | 6.02 | 0 | 4.3 | 2.45 | 2.62 | 0.0271 | 4.18 | |
207At | 203Bi | 5.87 | 0 | 4.81 | 3.12 | 3.29 | 0.0235 | 4.92 | 209At | 205Bi | 5.76 | 0 | 5.67 | 3.66 | 3.83 | 0.0203 | 5.52 | |
211At | 207Bi | 5.98 | 0 | 4.79 | 2.49 | 2.66 | 0.0171 | 4.42 | 213At | 209Bi | 9.25 | 0 | ?6.9 | ?8.4 | ?8.2 | 0.0623 | ?7 | |
215At | 211Bi | 8.18 | 0 | ?4 | ?5.6 | ?5.45 | 0.0663 | ?4.27 | 217At | 213Bi | 7.2 | 0 | ?1.49 | ?2.52 | ?2.35 | 0.0716 | ?1.2 | |
219At | 215Bi | 6.34 | 0 | 1.78 | 0.74 | 0.91 | 0.0781 | 2.02 | 193Rn | 189Po | 8.04 | 2 | ?2.94 | ?4.15 | ?3.96 | 0.0601 | ?2.74 | |
195Rn | 191Po | 7.69 | 0 | ?2.15 | ?3.39 | ?3.2 | 0.0642 | ?2 | 195Rnm | 191Pom | 7.71 | 0 | ?2.22 | ?3.45 | ?3.26 | 0.0641 | ?2.07 | |
197Rn | 193Po | 7.41 | 0 | ?1.27 | ?2.49 | ?2.31 | 0.056 | ?1.06 | 197Rnm | 193Pom | 7.51 | 0 | ?1.59 | ?2.82 | ?2.64 | 0.0556 | ?1.39 | |
203Rn | 199Po | 6.63 | 0 | 1.82 | 0.29 | 0.45 | 0.0369 | 1.88 | 203Rnm | 199Pom | 6.68 | 0 | 1.55 | 0.08 | 0.24 | 0.0368 | 1.67 | |
205Rn | 201Po | 6.39 | 2 | 2.84 | 1.57 | 1.74 | 0.0263 | 3.32 | 207Rn | 203Po | 6.25 | 0 | 3.42 | 1.84 | 2.02 | 0.0277 | 3.57 | |
209Rn | 205Po | 6.16 | 0 | 4 | 2.25 | 2.41 | 0.0239 | 4.03 | 211Rn | 207Po | 5.97 | 2 | 5.28 | 3.34 | 3.5 | 0.017 | 5.27 | |
213Rn | 209Po | 8.25 | 5 | ?1.71 | ?4.03 | ?3.86 | 0.0224 | ?2.21 | 215Rn | 211Po | 8.84 | 0 | ?5.64 | ?7.06 | ?6.9 | 0.0621 | ?5.69 | |
217Rn | 213Po | 7.89 | 0 | ?3.27 | ?4.38 | ?4.21 | 0.0656 | ?3.03 | 219Rn | 215Po | 6.95 | 2 | 0.6 | ?0.95 | ?0.78 | 0.0419 | 0.59 | |
221Rn | 217Po | 6.16 | 2 | 3.84 | 2.27 | 2.44 | 0.0455 | 3.78 | 223Rn | 219Po | 5.28 | 2 | 8.56 | 6.73 | 6.88 | 0.0526 | 8.16 | |
197Fr | 193Atm | 7.88 | 0 | ?2.63 | ?3.64 | ?3.45 | 0.0677 | ?2.29 | 199Fr | 195At | 7.82 | 0 | ?2.18 | ?3.45 | ?3.27 | 0.0582 | ?2.03 | |
199Frm | 195Atm | 7.83 | 0 | ?2.19 | ?3.5 | ?3.32 | 0.0581 | ?2.09 | 201Fr | 197At | 7.52 | 0 | ?1.2 | ?2.54 | ?2.35 | 0.0506 | ?1.06 | |
201Frm | 197Atm | 7.6 | 0 | ?1.77 | ?2.81 | ?2.63 | 0.0504 | ?1.33 | 203Fr | 199At | 7.27 | 0 | ?0.26 | ?1.73 | ?1.56 | 0.0439 | ?0.2 |
Table 5-continued from previous page | ||||||||||||||||||
α transition | ![]() ![]() | ![]() ![]() | ![]() ![]() | ![]() ![]() | ![]() ![]() | ![]() ![]() | ![]() ![]() | α transition | ![]() ![]() | ![]() ![]() | ![]() ![]() | ![]() ![]() | ![]() ![]() | ![]() ![]() | ![]() ![]() | |||
203Frm | 199Atm | 7.39 | 0 | ?0.68 | ?2.14 | ?1.97 | 0.0436 | ?0.61 | 205Fr | 201At | 7.05 | 0 | 0.58 | ?0.95 | ?0.78 | 0.038 | 0.64 | |
207Fr | 203At | 6.89 | 0 | 1.19 | ?0.37 | ?0.19 | 0.0328 | 1.29 | 209Fr | 205At | 6.78 | 0 | 1.75 | 0.06 | 0.23 | 0.0283 | 1.78 | |
211Fr | 207At | 6.66 | 0 | 2.33 | 0.45 | 0.61 | 0.0244 | 2.23 | 213Fr | 209At | 6.91 | 0 | 1.54 | ?0.53 | ?0.36 | 0.0209 | 1.32 | |
215Fr | 211At | 9.54 | 0 | ?7.07 | ?8.42 | ?8.26 | 0.0582 | ?7.03 | 219Fr | 215At | 7.45 | 0 | ?1.7 | ?2.62 | ?2.45 | 0.0667 | ?1.27 | |
221Fr | 217At | 6.46 | 2 | 2.46 | 1.43 | 1.59 | 0.0439 | 2.95 | 223Fr | 219At | 5.56 | 4 | 7.34 | 6.36 | 6.51 | 0.0324 | 8 | |
201Ra | 197Rn | 8 | 0 | ?1.7 | ?3.7 | ?3.51 | 0.0616 | ?2.3 | 201Ram | 197Rnm | 8.07 | 0 | ?2.22 | ?3.89 | ?3.71 | 0.0615 | ?2.5 | |
203Ra | 199Rn | 7.74 | 0 | ?1.44 | ?2.89 | ?2.72 | 0.0533 | ?1.45 | 203Ram | 199Rnm | 7.76 | 0 | ?1.6 | ?2.98 | ?2.81 | 0.0533 | ?1.54 | |
207Ra | 203Rn | 7.27 | 2 | 0.21 | ?1.06 | ?0.88 | 0.0327 | 0.6 | 209Ra | 205Rn | 7.14 | 0 | 0.67 | ?0.91 | ?0.74 | 0.0343 | 0.72 | |
213Ra | 209Rn | 6.86 | 2 | 2.31 | 0.35 | 0.52 | 0.0209 | 2.2 | 215Ra | 211Rn | 8.86 | 5 | ?2.78 | ?5.09 | ?4.96 | 0.0199 | ?3.25 | |
217Ra | 213Rn | 9.16 | 0 | ?5.79 | ?7.22 | ?7.04 | 0.0575 | ?5.8 | 219Ra | 215Rn | 8.14 | 2 | ?2 | ?4.17 | ?3.99 | 0.0361 | ?2.55 | |
221Ra | 217Rn | 6.88 | 2 | 1.45 | 0.14 | 0.31 | 0.0414 | 1.69 | 223Ra | 219Rn | 5.98 | 2 | 5.99 | 4.04 | 4.2 | 0.0465 | 5.53 | |
205Ac | 201Frn | 7.9 | 3 | ?1.1 | ?2.48 | ?2.31 | 0.0427 | ?0.94 | 207Ac | 203Fr | 7.85 | 0 | ?1.51 | ?2.92 | ?2.74 | 0.0486 | ?1.42 | |
211Ac | 207Fr | 7.62 | 0 | ?0.67 | ?2.25 | ?2.08 | 0.0361 | ?0.64 | 215Ac | 211Fr | 7.75 | 0 | ?0.77 | ?2.73 | ?2.59 | 0.0269 | ?1.02 | |
217Ac | 213Fr | 9.83 | 0 | ?7.16 | ?8.45 | ?8.27 | 0.0542 | ?7 | 217Acm | 213Fr | 11.84 | 11 | ?4.77 | ?7.07 | ?6.93 | 0.0037 | ?4.5 | |
219Ac | 215Fr | 8.83 | 0 | ?4.93 | ?6.04 | ?5.86 | 0.0567 | ?4.61 | 221Ac | 217Fr | 7.78 | 0 | ?1.28 | ?2.95 | ?2.78 | 0.0612 | ?1.56 | |
223Ac | 219Fr | 6.78 | 2 | 2.1 | 0.93 | 1.08 | 0.04 | 2.48 | 225Ac | 221Fr | 5.94 | 2 | 5.93 | 4.72 | 4.87 | 0.0446 | 6.23 | |
227Ac | 223Fr | 5.04 | 0 | 10.7 | 9.38 | 9.54 | 0.0897 | 10.59 | 209Thm | 205Ram | 8.28 | 0 | ?2.51 | ?3.91 | ?3.73 | 0.0517 | ?2.45 | |
215Th | 211Ra | 7.67 | 2 | 0.08 | ?1.79 | ?1.65 | 0.027 | ?0.08 | 217Th | 213Ra | 9.44 | 5 | ?3.61 | ?5.9 | ?5.74 | 0.0178 | ?3.99 | |
219Th | 215Ra | 9.51 | 0 | ?5.99 | ?7.44 | ?7.26 | 0.0531 | ?5.98 | 221Th | 217Ra | 8.63 | 2 | ?2.75 | ?4.87 | ?4.7 | 0.0324 | ?3.21 | |
223Th | 219Ra | 7.57 | 2 | ?0.22 | ?1.6 | ?1.44 | 0.0354 | 0.01 | 225Th | 221Ra | 6.92 | 2 | 2.77 | 0.78 | 0.94 | 0.0368 | 2.38 | |
227Th | 223Ra | 6.15 | 2 | 6.21 | 4.08 | 4.24 | 0.0402 | 5.64 | 229Th | 225Ra | 5.17 | 2 | 11.4 | 9.4 | 9.54 | 0.0484 | 10.86 | |
231Th | 227Ra | 4.21 | 2 | 17.36 | 16.34 | 16.48 | 0.063 | 17.68 | 213Pa | 209Ac | 8.4 | 0 | ?2.15 | ?3.97 | ?3.79 | 0.0476 | ?2.47 | |
215Pa | 211Ac | 8.24 | 0 | ?1.85 | ?3.52 | ?3.37 | 0.0409 | ?1.98 | 217Pa | 213Ac | 8.49 | 0 | ?2.46 | ?4.31 | ?4.14 | 0.0357 | ?2.7 | |
221Pa | 217Ac | 9.25 | 0 | ?5.23 | ?6.48 | ?6.29 | 0.0517 | ?5.01 | 223Pa | 219Ac | 8.33 | 0 | ?2.29 | ?3.93 | ?3.77 | 0.0543 | ?2.5 | |
227Pa | 223Ac | 6.58 | 0 | 3.43 | 2.29 | 2.45 | 0.0634 | 3.65 | 229Pa | 225Ac | 5.84 | 1 | 7.43 | 5.91 | 6.06 | 0.0515 | 7.34 | |
219U | 215Th | 9.94 | 5 | ?4.26 | ?6.51 | ?6.34 | 0.0161 | ?4.55 | 221U | 217Th | 9.89 | 0 | ?6.18 | ?7.68 | ?7.5 | 0.0487 | ?6.19 | |
225U | 221Th | 8.02 | 2 | ?1.21 | ?2.34 | ?2.18 | 0.0317 | ?0.68 | 227U | 223Th | 7.23 | 2 | 1.82 | 0.34 | 0.5 | 0.0335 | 1.98 | |
229U | 225Th | 6.48 | 0 | 4.24 | 3.18 | 3.33 | 0.0614 | 4.54 | 231U | 227Th | 5.58 | 2 | 9.95 | 7.99 | 8.13 | 0.0419 | 9.51 | |
233U | 229Th | 4.91 | 0 | 12.7 | 11.87 | 12.02 | 0.0808 | 13.11 | 227Np | 223Pa | 7.82 | 2 | ?0.29 | ?1.34 | ?1.18 | 0.0309 | 0.33 | |
229Np | 225Pa | 7.02 | 1 | 2.55 | 1.41 | 1.56 | 0.0414 | 2.95 | 231Np | 227Pa | 6.36 | 1 | 5.14 | 4.22 | 4.37 | 0.044 | 5.72 | |
235Np | 231Pa | 5.19 | 1 | 12.12 | 10.52 | 10.64 | 0.0515 | 11.93 | 237Np | 233Pa | 4.96 | 1 | 13.83 | 12.07 | 12.21 | 0.0515 | 13.49 | |
239Np | 235Pa | 4.6 | 1 | 16.61 | 14.68 | 14.82 | 0.0542 | 16.08 | 231Pu | 227U | 6.84 | 0 | 3.58 | 2.46 | 2.61 | 0.055 | 3.87 | |
233Pu | 229U | 6.41 | 2 | 6 | 4.61 | 4.76 | 0.0329 | 6.24 | 235Pu | 231U | 5.95 | 0 | 7.72 | 6.55 | 6.67 | 0.0577 | 7.91 | |
239Pu | 235Uxm | 5.24 | 0 | 11.88 | 10.6 | 10.74 | 0.0604 | 11.96 | 241Pu | 237U | 5.14 | 2 | 13.26 | 11.54 | 11.68 | 0.034 | 13.15 | |
229Am | 225Np | 8.14 | 2 | 0.3 | ?1.67 | ?1.51 | 0.0282 | 0.04 | 233Am | 229Np | 7.06 | 1 | 3.62 | 2.07 | 2.22 | 0.0366 | 3.66 | |
235Am | 231Np | 6.59 | 1 | 5.18 | 4.01 | 4.14 | 0.0374 | 5.57 | 237Am | 233Np | 6.2 | 1 | 7.24 | 5.84 | 5.99 | 0.0378 | 7.41 | |
239Am | 235Np | 5.92 | 1 | 8.63 | 7.24 | 7.38 | 0.0375 | 8.81 | 241Am | 237Np | 5.64 | 1 | 10.14 | 8.8 | 8.95 | 0.0375 | 10.37 | |
243Am | 239Np | 5.44 | 1 | 11.37 | 9.96 | 10.1 | 0.0368 | 11.53 | 233Cm | 229Pu | 7.47 | 0 | 2.13 | 0.78 | 0.94 | 0.0468 | 2.27 | |
237Cm | 233Pu | 6.78 | 0 | 4.82 | 3.5 | 3.64 | 0.0459 | 4.98 | 241Cm | 237Pu | 6.19 | 3 | 8.45 | 6.78 | 6.93 | 0.0213 | 8.6 | |
243Cm | 239Pu | 6.17 | 2 | 8.96 | 6.57 | 6.71 | 0.0246 | 8.32 | 245Cm | 241Pu | 5.62 | 2 | 11.42 | 9.46 | 9.59 | 0.0264 | 11.17 | |
247Bk | 243Am | 5.89 | 2 | 10.64 | 8.43 | 8.56 | 0.0232 | 10.2 | 241Cf | 237Cmp | 7.46 | 0 | 2.75 | 1.51 | 1.66 | 0.0354 | 3.11 | |
243Cf | 239Cm | 7.42 | 3 | 3.66 | 2.16 | 2.29 | 0.0156 | 4.1 | 245Cf | 241Cm | 7.26 | 0 | 3.87 | 2.18 | 2.32 | 0.0314 | 3.83 |
Table 5-continued from previous page | ||||||||||||||||||
α transition | ![]() ![]() | ![]() ![]() | ![]() ![]() | ![]() ![]() | ![]() ![]() | ![]() ![]() | ![]() ![]() | α transition | ![]() ![]() | ![]() ![]() | ![]() ![]() | ![]() ![]() | ![]() ![]() | ![]() ![]() | ![]() ![]() | |||
247Cf | 243Cm | 6.5 | 2 | 7.5 | 5.78 | 5.92 | 0.0203 | 7.61 | 251Cf | 247Cm | 6.18 | 5 | 10.45 | 8.38 | 8.51 | 0.0098 | 10.52 | |
245Es | 241Bkp | 7.87 | 0 | 2.22 | 0.28 | 0.42 | 0.0285 | 1.97 | 247Es | 243Bkp | 7.44 | 1 | 3.59 | 1.93 | 2.08 | 0.021 | 3.75 | |
249Es | 245Bkp | 6.9 | 1 | 6.03 | 4.2 | 4.34 | 0.0218 | 6 | 253Es | 249Bk | 6.74 | 0 | 6.25 | 4.77 | 4.91 | 0.0262 | 6.49 | |
255Es | 251Bkm | 6.4 | 0 | 7.63 | 6.3 | 6.44 | 0.0263 | 8.02 | 243Fm | 239Cf | 8.7 | 1 | ?0.6 | ?1.98 | ?1.83 | 0.0201 | ?0.14 | |
247Fm | 243Cf | 8.26 | 4 | 1.69 | 0.13 | 0.27 | 0.0095 | 2.29 | 247Fmm | 243Cf | 8.31 | 0 | 0.76 | ?0.88 | ?0.73 | 0.0248 | 0.87 | |
253Fm | 249Cfm | 7.05 | 2 | 6.33 | 4.07 | 4.21 | 0.0147 | 6.04 | 257Fm | 253Cf | 6.86 | 2 | 6.94 | 4.81 | 4.93 | 0.0131 | 6.82 | |
247Md | 243Es | 8.77 | 1 | 0.08 | ?1.91 | ?1.76 | 0.0172 | 0.004 | 247Mdm | 243Es | 9.03 | 3 | ?0.5 | ?2.29 | ?2.14 | 0.0106 | ?0.17 | |
251Md | 247Es | 7.96 | 1 | 3.4 | 0.73 | 0.86 | 0.0169 | 2.64 | 251No | 247Fm | 8.76 | 0 | ?0.02 | ?1.65 | ?1.51 | 0.0203 | 0.18 | |
251Nom | 247Fmm | 8.82 | 0 | 0.01 | ?1.84 | ?1.7 | 0.0201 | ?0.003 | 253No | 249Fm | 8.42 | 1 | 2.23 | ?0.48 | ?0.34 | 0.0146 | 1.5 | |
255No | 251Fmm | 8.23 | 2 | 2.84 | 0.26 | 0.4 | 0.0112 | 2.35 | 259No | 255Fm | 7.85 | 2 | 3.66 | 1.56 | 1.69 | 0.0102 | 3.68 | |
253Lr | 249Md | 8.93 | 0 | ?0.15 | ?1.84 | ?1.7 | 0.0185 | 0.03 | 253Lrm | 249Mdm | 8.86 | 0 | 0.17 | ?1.62 | ?1.48 | 0.0187 | 0.25 | |
255Lr | 251Mdp | 8.5 | 0 | 1.49 | ?0.54 | ?0.4 | 0.0183 | 1.34 | 257Lr | 253Md | 9.08 | 4 | 0.78 | ?1.58 | ?1.45 | 0.0059 | 0.78 | |
259Lr | 255Mdp | 8.58 | 0 | 0.9 | ?0.83 | ?0.7 | 0.0155 | 1.11 | 255Rf | 251No | 9.06 | 1 | 0.54 | ?1.86 | ?1.71 | 0.0125 | 0.19 | |
257Rfm | 253No | 9.16 | 2 | 0.69 | ?2.03 | ?1.9 | 0.0091 | 0.14 | 259Rf | 255Nop | 9.03 | 0 | 0.46 | ?1.9 | ?1.76 | 0.0146 | 0.07 | |
261Rf | 257No | 8.65 | 0 | 0.9 | ?0.72 | ?0.59 | 0.0144 | 1.26 | 263Rf | 259No | 8.26 | 4 | 3.34 | 1.36 | 1.5 | 0.0054 | 3.77 | |
259Db | 255Lrm | 9.58 | 1 | ?0.29 | ?3.11 | ?2.97 | 0.01 | ?0.97 | 259Sg | 255Rf | 9.77 | 2 | ?0.38 | ?3.14 | ?3 | 0.0079 | ?0.9 | |
259Sgm | 255Rfm | 9.71 | 2 | ?0.63 | ?2.97 | ?2.84 | 0.008 | ?0.74 | 261Sg | 257Rf | 9.71 | 2 | ?0.73 | ?3.02 | ?2.88 | 0.0074 | ?0.75 | |
263Sg | 259Rf | 9.41 | 0 | 0.03 | ?2.41 | ?2.27 | 0.0121 | ?0.35 | 261Bh | 257Db | 10.5 | 3 | ?1.87 | ?4.53 | ?4.39 | 0.0054 | ?2.12 | |
265Hs | 261Sg | 10.47 | 0 | ?2.71 | ?4.7 | ?4.56 | 0.0099 | ?2.56 | 269Hs | 265Sg | 9.35 | 0 | 1.2 | ?1.62 | ?1.48 | 0.0099 | 0.52 | |
267Ds | 263Hs | 11.78 | 0 | ?5 | ?7.1 | ?6.96 | 0.0079 | ?4.85 | 269Ds | 265Hsm | 11.28 | 0 | ?3.64 | ?6.04 | ?5.9 | 0.0078 | ?3.79 | |
271Ds | 267Hs | 10.88 | 5 | ?1.05 | ?4.01 | ?3.88 | 0.0023 | ?1.24 | 271Dsm | 267Hs | 10.95 | 2 | ?2.77 | ?5.08 | ?4.94 | 0.0044 | ?2.58 | |
273Ds | 269Hs | 11.38 | 3 | ?3.62 | ?5.92 | ?5.79 | 0.0031 | ?3.28 | 277Ds | 273Hs | 10.83 | 4 | ?2.22 | ?4.39 | ?4.26 | 0.0023 | ?1.62 | |
277Cn | 273Dsm | 11.42 | 0 | ?3.07 | ?5.9 | ?5.76 | 0.0057 | ?3.52 | 281Cn | 277Ds | 10.46 | 4 | ?0.74 | ?2.87 | ?2.75 | 0.0021 | ?0.07 | |
289Fl | 285Cn | 9.97 | 0 | 0.38 | ?1.72 | ?1.6 | 0.0044 | 0.76 |
α transition | ![]() ![]() | ![]() ![]() | ![]() ![]() | ![]() ![]() | ![]() ![]() | ![]() ![]() | ![]() ![]() | α transition | ![]() ![]() | ![]() ![]() | ![]() ![]() | ![]() ![]() | ![]() ![]() | ![]() ![]() | ![]() ![]() | |||
148Eu | 144Pm | 2.69 | 0 | 14.98 | 13.77 | 13.95 | 0.0795 | 15.04 | 152Ho | 148Tb | 4.51 | 0 | 3.12 | 1.83 | 1.95 | 0.0634 | 3.14 | |
152Hom | 148Tbm | 4.58 | 0 | 2.66 | 1.44 | 1.56 | 0.0635 | 2.76 | 154Ho | 150Tb | 4.04 | 0 | 6.56 | 4.65 | 4.88 | 0.0582 | 6.11 | |
154Tm | 150Ho | 5.09 | 0 | 1.17 | ?0.16 | 0.08 | 0.059 | 1.3 | 154Tmm | 150Hom | 5.18 | 0 | 0.75 | ?0.54 | ?0.31 | 0.0592 | 0.92 | |
156Tm | 152Ho | 4.35 | 0 | 5.12 | 3.88 | 4.03 | 0.0533 | 5.3 | 156Lum | 152Tmm | 5.72 | 0 | ?0.68 | ?1.96 | ?1.78 | 0.0558 | ?0.53 | |
158Ta | 154Lu | 6.13 | 0 | ?1.29 | ?2.71 | ?2.62 | 0.0526 | ?1.34 | 158Tam | 154Lum | 6.21 | 0 | ?1.42 | ?3.01 | ?2.91 | 0.0528 | ?1.63 | |
160Re | 156Ta | 6.7 | 2 | ?2.26 | ?3.6 | ?3.46 | 0.0303 | ?1.94 | 162Re | 158Ta | 6.25 | 0 | ?0.95 | ?2.32 | ?2.15 | 0.0456 | ?0.81 | |
162Rem | 158Tam | 6.28 | 0 | ?1.07 | ?2.43 | ?2.26 | 0.0457 | ?0.92 | 164Rem | 160Tam | 5.77 | 0 | 1.46 | ?0.43 | ?0.22 | 0.0411 | 1.16 | |
164Irm | 160Rem | 7.06 | 0 | ?2.78 | ?4.36 | ?4.15 | 0.0445 | ?2.8 | 166Ir | 162Re | 6.73 | 0 | ?1.95 | ?3.29 | ?3.15 | 0.0405 | ?1.76 | |
166Irm | 162Rem | 6.73 | 0 | ?1.81 | ?3.29 | ?3.15 | 0.0405 | ?1.76 | 168Ir | 164Re | 6.38 | 0 | ?0.64 | ?2.03 | ?1.9 | 0.0368 | ?0.46 | |
168Irm | 164Rem | 6.48 | 0 | ?0.68 | ?2.41 | ?2.27 | 0.0371 | ?0.84 | 170Ir | 166Rep | 5.96 | 0 | 1.24 | ?0.37 | ?0.17 | 0.0332 | 1.31 | |
170Irm | 166Re | 6.27 | 2 | 0.35 | ?1.32 | ?1.12 | 0.0205 | 0.57 | 172Ir | 168Re | 5.99 | 3 | 2.34 | 0.06 | 0.16 | 0.0151 | 1.98 | |
172Irm | 168Re | 6.13 | 0 | 1.36 | ?1.16 | ?1.04 | 0.0314 | 0.46 | 174Ir | 170Re | 5.63 | 2 | 3.17 | 1.31 | 1.5 | 0.0168 | 3.27 | |
174Irm | 170Re | 5.82 | 2 | 2.29 | 0.44 | 0.63 | 0.0172 | 2.4 | 170Au | 166Ir | 7.18 | 0 | ?2.58 | ?4.03 | ?3.82 | 0.0362 | ?2.38 | |
Continued on next page |
Table6.Same as Tables 4 and 5, but for α decay of doubly odd nuclei.
Table 6-continued from previous page | ||||||||||||||||||
α transition | ![]() ![]() | ![]() ![]() | ![]() ![]() | ![]() ![]() | ![]() ![]() | ![]() ![]() | ![]() ![]() | α transition | ![]() ![]() | ![]() ![]() | ![]() ![]() | ![]() ![]() | ![]() ![]() | ![]() ![]() | ![]() ![]() | |||
170Aum | 166Irm | 7.29 | 0 | ?2.84 | ?4.38 | ?4.17 | 0.0365 | ?2.74 | 176Au | 172Ir | 6.44 | 0 | 0.14 | ?1.52 | ?1.32 | 0.0277 | 0.24 | |
186Au | 182Ir | 4.91 | 1 | 7.89 | 5.59 | 5.77 | 0.0122 | 7.69 | 180Tl | 176Aum | 6.57 | 3 | 1.23 | ?0.62 | ?0.43 | 0.0117 | 1.5 | |
186Tlm | 182Au | 6.02 | 6 | 5.66 | 3.01 | 3.18 | 0.0048 | 5.5 | 186Bi | 182Tl | 7.76 | 1 | ?1.83 | ?4.51 | ?4.32 | 0.0162 | ?2.53 | |
186Bim | 182Tlm | 7.88 | 3 | ?2.01 | ?4.38 | ?4.19 | 0.0107 | ?2.22 | 190Bi | 186Tl | 6.86 | 1 | 0.91 | ?1.56 | ?1.46 | 0.013 | 0.43 | |
190Bim | 186Tlm | 6.97 | 3 | 0.94 | ?1.45 | ?1.35 | 0.0086 | 0.72 | 192Bi | 188Tl | 6.38 | 1 | 2.44 | 0.29 | 0.47 | 0.0115 | 2.41 | |
192Bim | 188Tlm | 6.49 | 3 | 2.58 | 0.36 | 0.54 | 0.0076 | 2.66 | 194Bi | 190Tl | 5.92 | 1 | 4.31 | 2.25 | 2.43 | 0.0101 | 4.42 | |
194Bin | 190Tlm | 6.02 | 3 | 4.74 | 2.31 | 2.48 | 0.0067 | 4.66 | 196Bi | 192Tlp | 5.26 | 0 | 7.42 | 5.46 | 5.57 | 0.0114 | 7.51 | |
196Bin | 192Tln | 5.32 | 2 | 7.8 | 5.45 | 5.56 | 0.0069 | 7.72 | 212Bi | 208Tl | 6.21 | 5 | 4 | 1.99 | 2.15 | 0.0085 | 4.22 | |
214Bi | 210Tl | 5.62 | 5 | 6.75 | 4.73 | 4.89 | 0.0091 | 6.93 | 192At | 188Bi | 7.7 | 0 | ?1.94 | ?3.72 | ?3.54 | 0.0175 | ?1.78 | |
192Atm | 188Bim | 7.63 | 3 | ?1.06 | ?2.94 | ?2.76 | 0.0085 | ?0.69 | 194At | 190Bin | 7.33 | 0 | ?0.54 | ?2.59 | ?2.4 | 0.0158 | ?0.6 | |
194Atm | 190Bim | 7.31 | 0 | ?0.49 | ?2.49 | ?2.3 | 0.0157 | ?0.5 | 200At | 196Bi | 6.6 | 0 | 1.92 | 0.06 | 0.24 | 0.0119 | 2.17 | |
200Atn | 196Bim | 6.77 | 3 | 1.88 | ?0.05 | 0.12 | 0.0059 | 2.35 | 202At | 198Bi | 6.35 | 0 | 3.16 | 0.99 | 1.1 | 0.0108 | 3.07 | |
202Atm | 198Bim | 6.26 | 0 | 3.32 | 1.4 | 1.51 | 0.0106 | 3.48 | 202Atn | 198Bin | 6.4 | 0 | 2.68 | 0.79 | 0.9 | 0.0109 | 2.86 | |
204At | 200Bi | 6.07 | 0 | 4.16 | 2.22 | 2.38 | 0.0097 | 4.4 | 206At | 202Bi | 5.89 | 0 | 5.31 | 3.06 | 3.23 | 0.0088 | 5.29 | |
208At | 204Bi | 5.75 | 0 | 6.02 | 3.7 | 3.82 | 0.0081 | 5.91 | 210At | 206Bi | 5.63 | 2 | 7.22 | 4.52 | 4.68 | 0.0045 | 7.03 | |
212At | 208Bi | 7.82 | 5 | ?0.5 | ?3.1 | ?2.93 | 0.0061 | ?0.72 | 218At | 214Bi | 6.87 | 0 | 0.18 | ?1.38 | ?1.21 | 0.0501 | 0.09 | |
220At | 216Bim | 6.05 | 0 | 3.43 | 2.02 | 2.18 | 0.0542 | 3.45 | 200Frm | 196Atm | 7.71 | 0 | ?0.72 | ?3.11 | ?2.92 | 0.0133 | ?1.05 | |
212Fr | 208At | 6.53 | 2 | 3.44 | 1.27 | 1.44 | 0.0047 | 3.77 | 214Fr | 210At | 8.59 | 5 | ?2.29 | ?4.66 | ?4.49 | 0.0058 | ?2.25 | |
218Fr | 214At | 8.01 | 0 | ?3 | ?4.43 | ?4.26 | 0.0513 | ?2.97 | 218Frm | 214Atn | 7.87 | 2 | ?1.66 | ?3.7 | ?3.53 | 0.017 | ?1.76 | |
220Fr | 216At | 6.8 | 1 | 1.44 | ?0.16 | 0.01 | 0.0289 | 1.55 | 216Ac | 212Fr | 9.24 | 5 | ?3.36 | ?5.74 | ?5.57 | 0.0061 | ?3.36 | |
216Acm | 212Fr | 9.28 | 5 | ?3.36 | ?5.84 | ?5.68 | 0.006 | ?3.46 | 218Ac | 214Fr | 9.37 | 0 | ?6 | ?7.43 | ?7.25 | 0.0595 | ?6.02 | |
222Ac | 218Fr | 7.14 | 0 | 0.7 | ?0.72 | ?0.56 | 0.0548 | 0.7 | 226Ac | 222Fr | 5.51 | 2 | 9.23 | 6.98 | 7.13 | 0.0156 | 8.94 | |
220Pa | 216Ac | 9.65 | 0 | ?6.11 | ?7.45 | ?7.27 | 0.0698 | ?6.11 | 224Pa | 220Ac | 7.69 | 2 | ?0.07 | ?1.64 | ?1.48 | 0.017 | 0.29 | |
228Pa | 224Ac | 6.27 | 3 | 6.63 | 4.25 | 4.36 | 0.0078 | 6.47 | 230Pa | 226Ac | 5.44 | 2 | 10.67 | 8.27 | 8.41 | 0.011 | 10.37 | |
242Amm | 238Np | 5.64 | 3 | 11.99 | 9.26 | 9.4 | 0.0018 | 12.15 | 246Es | 242Bkp | 7.5 | 0 | 3.66 | 1.65 | 1.79 | 0.0101 | 3.79 | |
248Es | 244Bk | 7.16 | 2 | 5.76 | 3.24 | 3.38 | 0.0024 | 6 | 252Es | 248Bk | 6.79 | 2 | 7.72 | 4.83 | 4.96 | 0.0013 | 7.85 | |
254Esm | 250Bk | 6.7 | 1 | 7.64 | 4.96 | 5.1 | 0.0016 | 7.9 | 256Mdm | 252Es | 7.91 | 3 | 4.7 | 1.27 | 1.41 | 0.0008 | 4.5 | |
258Md | 254Es | 7.27 | 1 | 6.65 | 3.29 | 3.42 | 0.0014 | 6.27 |
The differences between logarithmic values of the three calculated α decay half-lives and the experimental data are denoted as black open squares, red solid squares, and blue solid circles in Figs. 2-4 for even-even nuclei, odd-
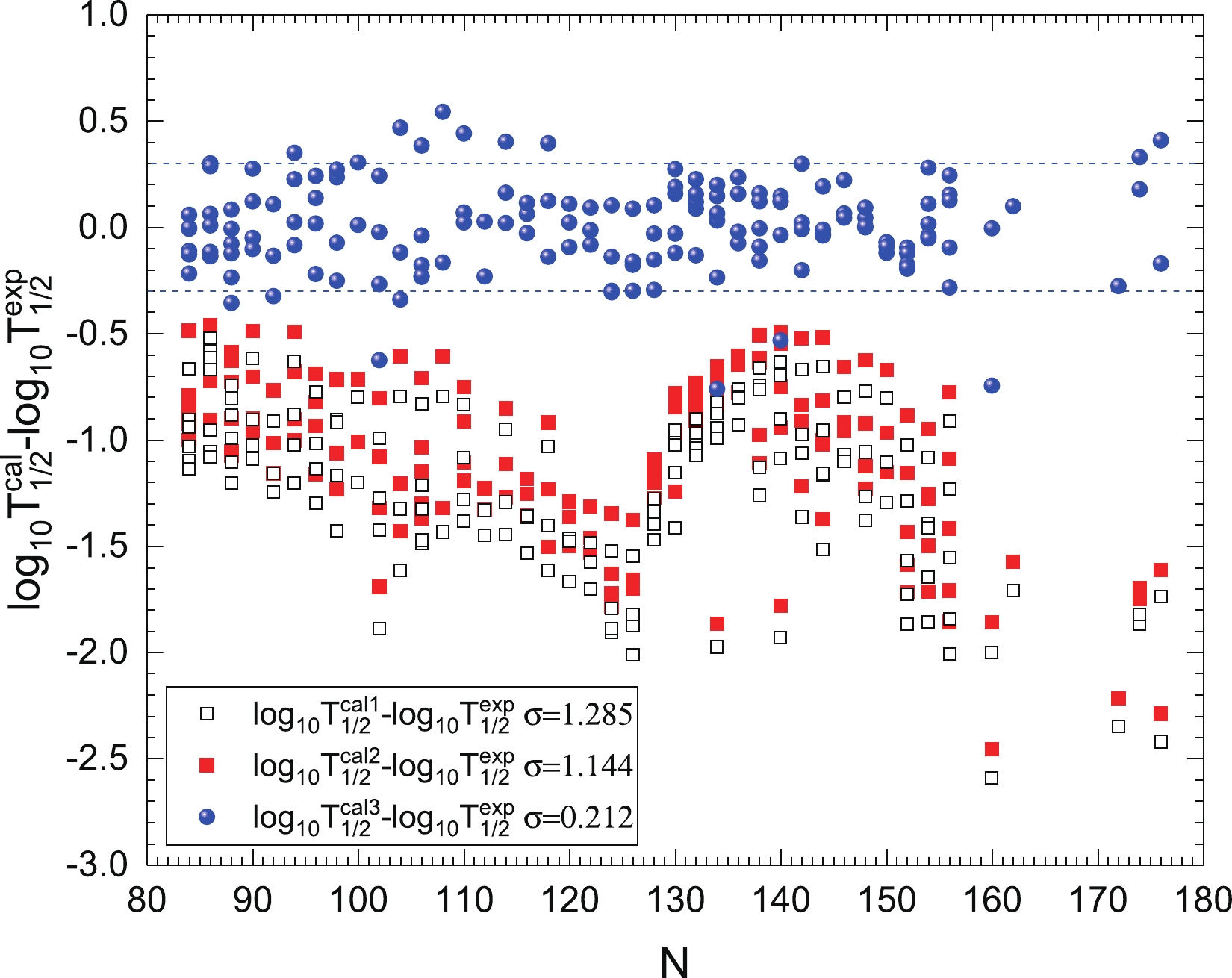
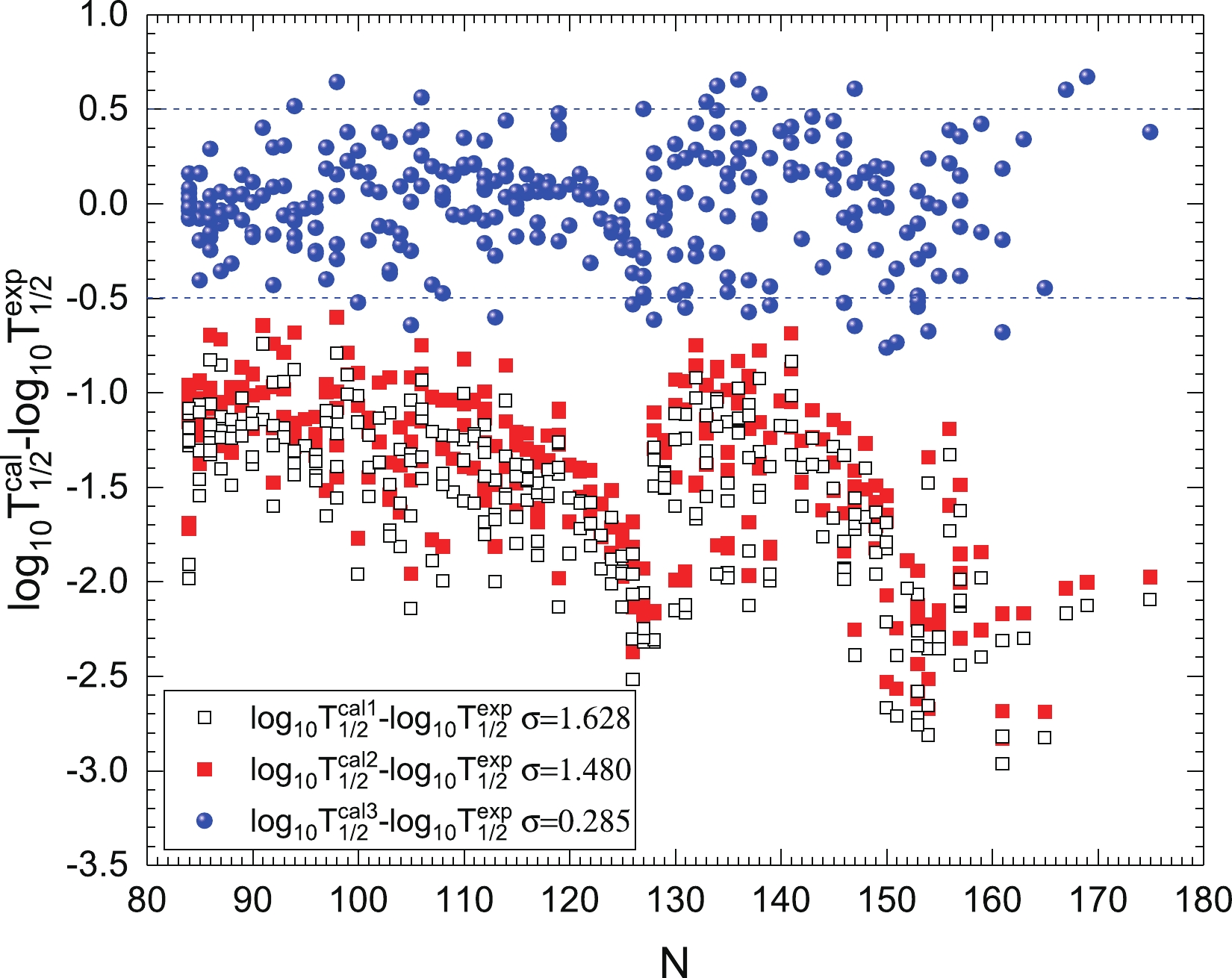
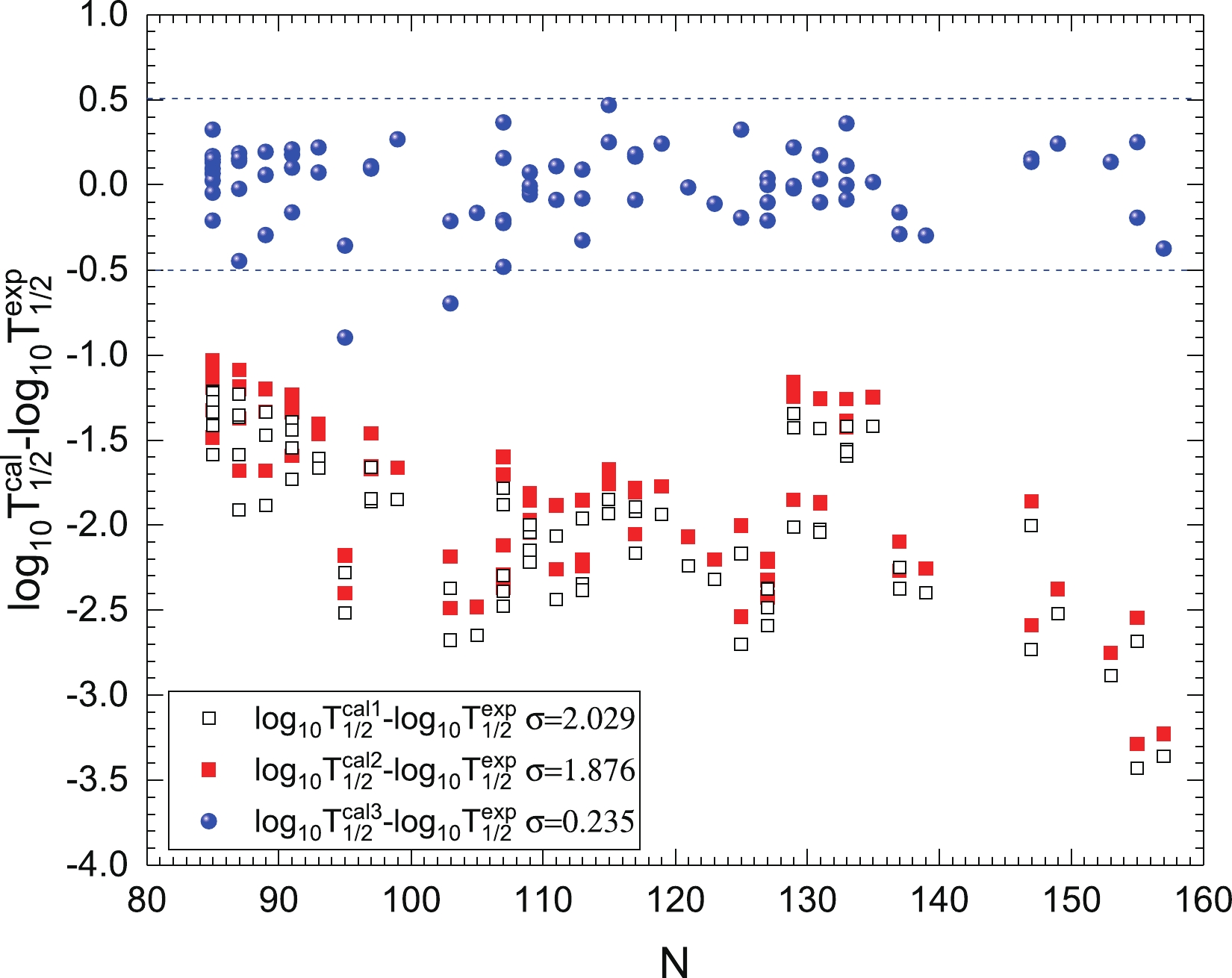
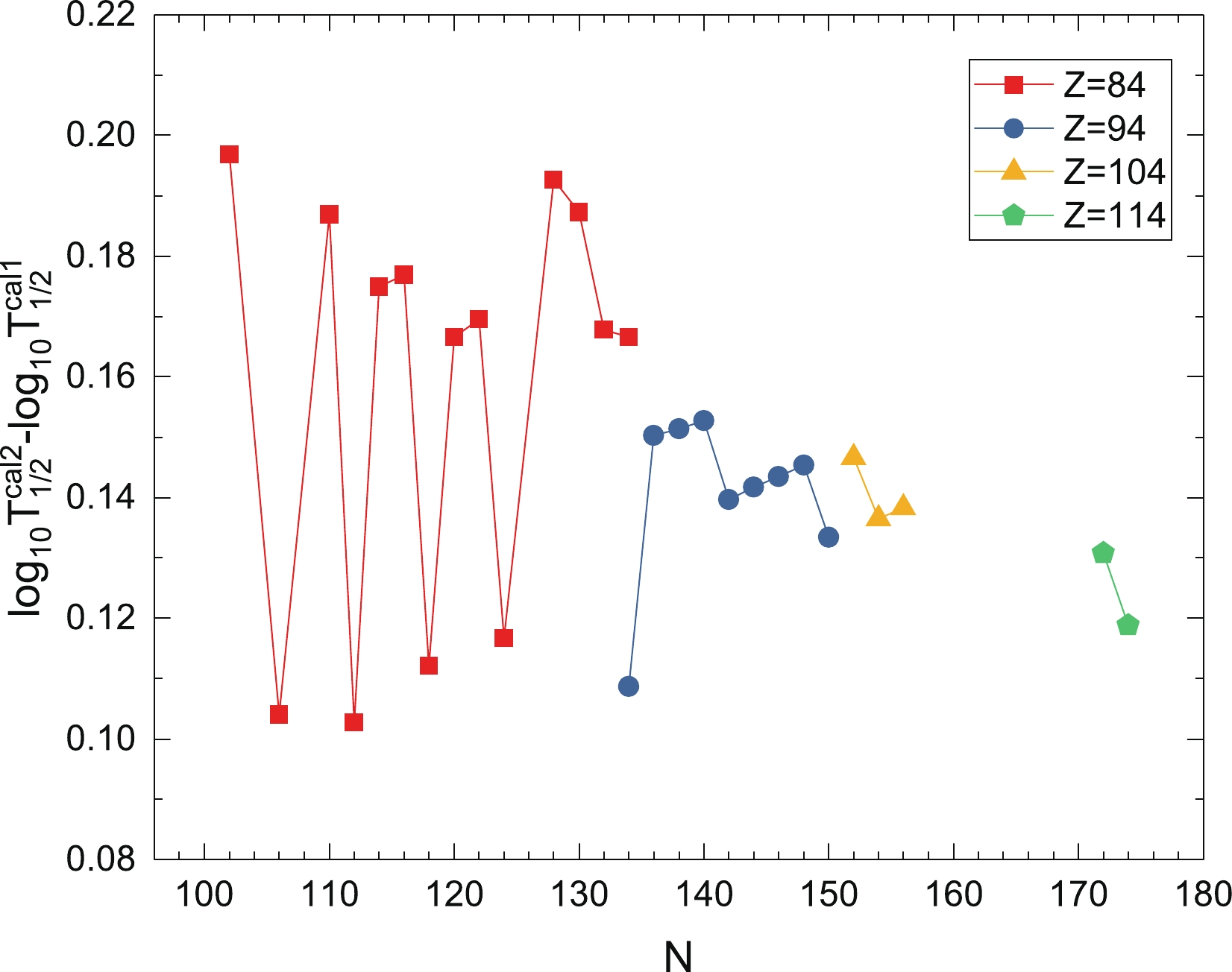
Encouraged by the good precision of the calculated α decay half-lives for known nuclei, the α decay half-lives of even-even superheavy nuclei with
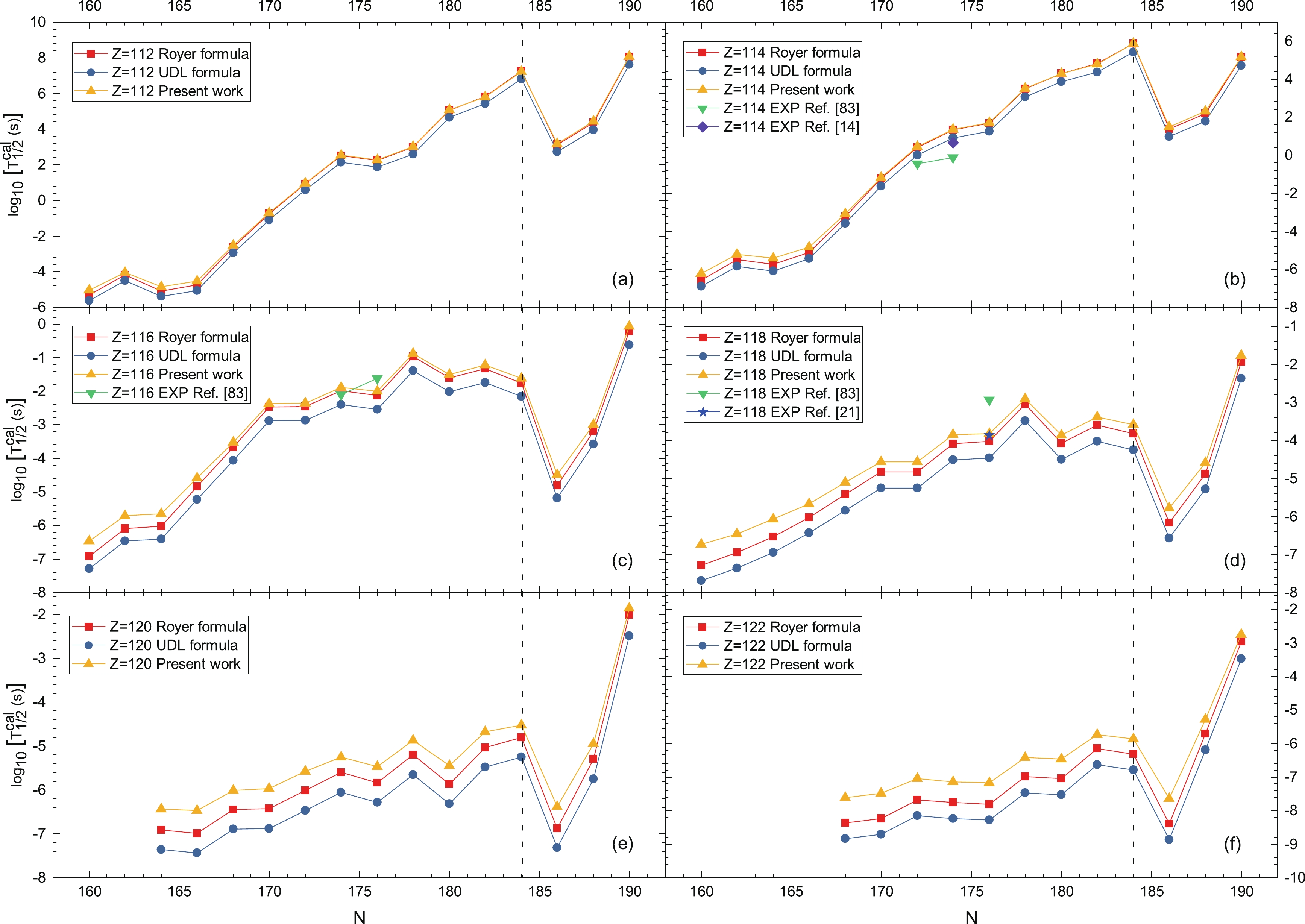
α transition | ![]() ![]() | ![]() ![]() | ![]() ![]() | ![]() ![]() | ![]() ![]() | α transition | ![]() ![]() | ![]() ![]() | ![]() ![]() | ![]() ![]() | ![]() ![]() | ||
Nucsth Z = 112 | |||||||||||||
272Cn | 268Ds | 12.05 | 0 | ?5.32 | ?5.62 | ?5.04 | 274Cn | 270Ds | 11.52 | 0 | ?4.18 | ?4.5 | ?4.04 |
276Cn | 272Ds | 11.9 | 0 | ?5.08 | ?5.38 | ?4.85 | 278Cn | 274Ds | 11.74 | 0 | ?4.75 | ?5.06 | ?4.52 |
280Cn | 276Ds | 10.83 | 0 | ?2.63 | ?2.96 | ?2.53 | 282Cn | 278Ds | 10.11 | 0 | ?0.76 | ?1.11 | ?0.69 |
284Cn | 280Ds | 9.52 | 0 | 0.94 | 0.57 | 0.97 | 286Cn | 282Ds | 9.01 | 0 | 2.51 | 2.13 | 2.53 |
288Cn | 284Ds | 9.09 | 0 | 2.24 | 1.86 | 2.27 | 290Cn | 286Ds | 8.85 | 0 | 2.98 | 2.59 | 3.01 |
292Cn | 288Ds | 8.27 | 0 | 5.06 | 4.65 | 5.08 | 294Cn | 290Ds | 8.06 | 0 | 5.83 | 5.41 | 5.81 |
296Cn | 292Ds | 7.7 | 0 | 7.24 | 6.81 | 7.21 | 298Cn | 294Ds | 8.77 | 0 | 3.12 | 2.73 | 3.18 |
300Cn | 296Ds | 8.42 | 0 | 4.36 | 3.96 | 4.42 | 302Cn | 298Ds | 7.49 | 0 | 8.05 | 7.61 | 8.08 |
Nuclei with Z = 114 | |||||||||||||
274Fl | 270Cn | 12.95 | 0 | ?6.58 | ?6.91 | ?6.21 | 276Fl | 272Cn | 12.41 | 0 | ?5.5 | ?5.84 | ?5.22 |
278Fl | 274Cn | 12.51 | 0 | ?5.75 | ?6.09 | ?5.43 | 280Fl | 276Cn | 12.19 | 0 | ?5.11 | ?5.45 | ?4.84 |
282Fl | 278Cn | 11.34 | 0 | ?3.23 | ?3.59 | ?3.08 | 284Fl | 280Cn | 10.54 | 0 | ?1.24 | ?1.62 | ?1.19 |
286Fl | 282Cn | 9.94 | 0 | 0.4 | ?0.002 | 0.45 | 288Fl | 284Cn | 9.62 | 0 | 1.33 | 0.92 | 1.35 |
290Fl | 286Cn | 9.5 | 0 | 1.68 | 1.26 | 1.71 | 292Fl | 288Cn | 8.93 | 0 | 3.49 | 3.06 | 3.52 |
294Fl | 290Cn | 8.69 | 0 | 4.3 | 3.86 | 4.29 | 296Fl | 292Cn | 8.54 | 0 | 4.81 | 4.37 | 4.8 |
298Fl | 294Cn | 8.25 | 0 | 5.87 | 5.41 | 5.87 | 300Fl | 296Cn | 9.54 | 0 | 1.37 | 0.97 | 1.48 |
302Fl | 298Cn | 9.27 | 0 | 2.19 | 1.78 | 2.31 | 304Fl | 300Cn | 8.41 | 0 | 5.15 | 4.71 | 5.17 |
Nuclei with Z = 116 | |||||||||||||
276Lv | 272Fl | 13.43 | 0 | ?6.92 | ?7.28 | ?6.47 | 278Lv | 274Fl | 12.99 | 0 | ?6.09 | ?6.47 | ?5.71 |
280Lv | 276Fl | 12.94 | 0 | ?6.03 | ?6.4 | ?5.66 | 282Lv | 278Fl | 12.35 | 0 | ?4.84 | ?5.23 | ?4.58 |
284Lv | 280Fl | 11.8 | 0 | ?3.67 | ?4.06 | ?3.52 | 286Lv | 282Fl | 11.28 | 0 | ?2.47 | ?2.88 | ?2.36 |
288Lv | 284Fl | 11.26 | 0 | ?2.45 | ?2.86 | ?2.36 | 290Lv | 286Fl | 11.06 | 0 | ?1.98 | ?2.4 | ?1.89 |
292Lv | 288Fl | 11.1 | 0 | ?2.13 | ?2.54 | ?2.01 | 294Lv | 290Fl | 10.64 | 0 | ?0.97 | ?1.39 | ?0.88 |
296Lv | 292Fl | 10.87 | 0 | ?1.61 | ?2.02 | ?1.51 | 298Lv | 294Fl | 10.75 | 0 | ?1.33 | ?1.74 | ?1.22 |
300Lv | 296Fl | 10.9 | 0 | ?1.76 | ?2.16 | ?1.61 | 302Lv | 298Fl | 12.17 | 0 | ?4.81 | ?5.18 | ?4.49 |
304Lv | 300Fl | 11.45 | 0 | ?3.19 | ?3.58 | ?3 | 306Lv | 302Fl | 10.29 | 0 | ?0.2 | ?0.62 | ?0.07 |
Nuclei with Z = 118 | |||||||||||||
278Og | 274Lv | 13.93 | 0 | ?7.28 | ?7.69 | ?6.74 | 280Og | 276Lv | 13.73 | 0 | ?6.95 | ?7.35 | ?6.45 |
282Og | 278Lv | 13.49 | 0 | ?6.54 | ?6.95 | ?6.07 | 284Og | 280Lv | 13.21 | 0 | ?6.02 | ?6.44 | ?5.66 |
286Og | 282Lv | 12.89 | 0 | ?5.42 | ?5.84 | ?5.1 | 288Og | 284Lv | 12.59 | 0 | ?4.83 | ?5.25 | ?4.57 |
290Og | 286Lv | 12.57 | 0 | ?4.83 | ?5.25 | ?4.56 | 292Og | 288Lv | 12.21 | 0 | ?4.08 | ?4.51 | ?3.84 |
294Og | 290Lv | 12.17 | 0 | ?4.03 | ?4.46 | ?3.82 | 296Og | 292Lv | 11.73 | 0 | ?3.04 | ?3.48 | ?2.91 |
298Og | 294Lv | 12.16 | 0 | ?4.07 | ?4.5 | ?3.86 | 300Og | 296Lv | 11.93 | 0 | ?3.59 | ?4.02 | ?3.38 |
302Og | 298Lv | 12.02 | 0 | ?3.83 | ?4.25 | ?3.59 | 304Og | 300Lv | 13.1 | 0 | ?6.17 | ?6.57 | ?5.77 |
306Og | 302Lv | 12.46 | 0 | ?4.87 | ?5.28 | ?4.59 | 308Og | 304Lv | 11.18 | 0 | ?1.93 | ?2.37 | ?1.77 |
Nuclei with Z = 120 | |||||||||||||
284120 | 280Og | 13.99 | 0 | ?6.91 | ?7.35 | ?6.44 | 286120 | 282Og | 14.01 | 0 | ?6.99 | ?7.43 | ?6.46 |
288120 | 284Og | 13.71 | 0 | ?6.45 | ?6.9 | ?6.01 | 290120 | 286Og | 13.68 | 0 | ?6.43 | ?6.88 | ?5.97 |
292120 | 288Og | 13.44 | 0 | ?6.01 | ?6.46 | ?5.57 | 294120 | 290Og | 13.22 | 0 | ?5.6 | ?6.05 | ?5.25 |
Continued on next page |
Table7.Predicted α decay half-lives of even-even nuclei with
Table 7-continued from previous page | |||||||||||||
α transition | ![]() ![]() | ![]() ![]() | ![]() ![]() | ![]() ![]() | ![]() ![]() | α transition | ![]() ![]() | ![]() ![]() | ![]() ![]() | ![]() ![]() | ![]() ![]() | ||
296120 | 292Og | 13.32 | 0 | ?5.84 | ?6.29 | ?5.46 | 298120 | 294Og | 12.98 | 0 | ?5.2 | ?5.65 | ?4.87 |
300120 | 296Og | 13.29 | 0 | ?5.87 | ?6.31 | ?5.45 | 302120 | 298Og | 12.87 | 0 | ?5.03 | ?5.48 | ?4.67 |
304120 | 300Og | 12.74 | 0 | ?4.8 | ?5.25 | ?4.53 | 306120 | 302Og | 13.77 | 0 | ?6.88 | ?7.31 | ?6.38 |
308120 | 304Og | 12.95 | 0 | ?5.3 | ?5.75 | ?4.95 | 310120 | 306Og | 11.48 | 0 | ?2.01 | ?2.49 | ?1.86 |
Nuclei with Z = 122 | |||||||||||||
290122 | 286120 | 15.09 | 0 | ?8.37 | ?8.84 | ?7.62 | 292122 | 288120 | 14.99 | 0 | ?8.24 | ?8.71 | ?7.48 |
294122 | 290120 | 14.64 | 0 | ?7.68 | ?8.15 | ?7.05 | 296122 | 292120 | 14.67 | 0 | ?7.76 | ?8.23 | ?7.14 |
298122 | 294120 | 14.68 | 0 | ?7.81 | ?8.28 | ?7.17 | 300122 | 296120 | 14.2 | 0 | ?6.99 | ?7.46 | ?6.42 |
302122 | 298120 | 14.21 | 0 | ?7.05 | ?7.52 | ?6.46 | 304122 | 300120 | 13.71 | 0 | ?6.15 | ?6.63 | ?5.73 |
306122 | 302120 | 13.78 | 0 | ?6.31 | ?6.79 | ?5.87 | 308122 | 304120 | 14.92 | 0 | ?8.4 | ?8.86 | ?7.64 |
310122 | 306120 | 13.44 | 0 | ?5.71 | ?6.19 | ?5.28 | 312122 | 308120 | 12.14 | 0 | ?2.97 | ?3.48 | ?2.75 |
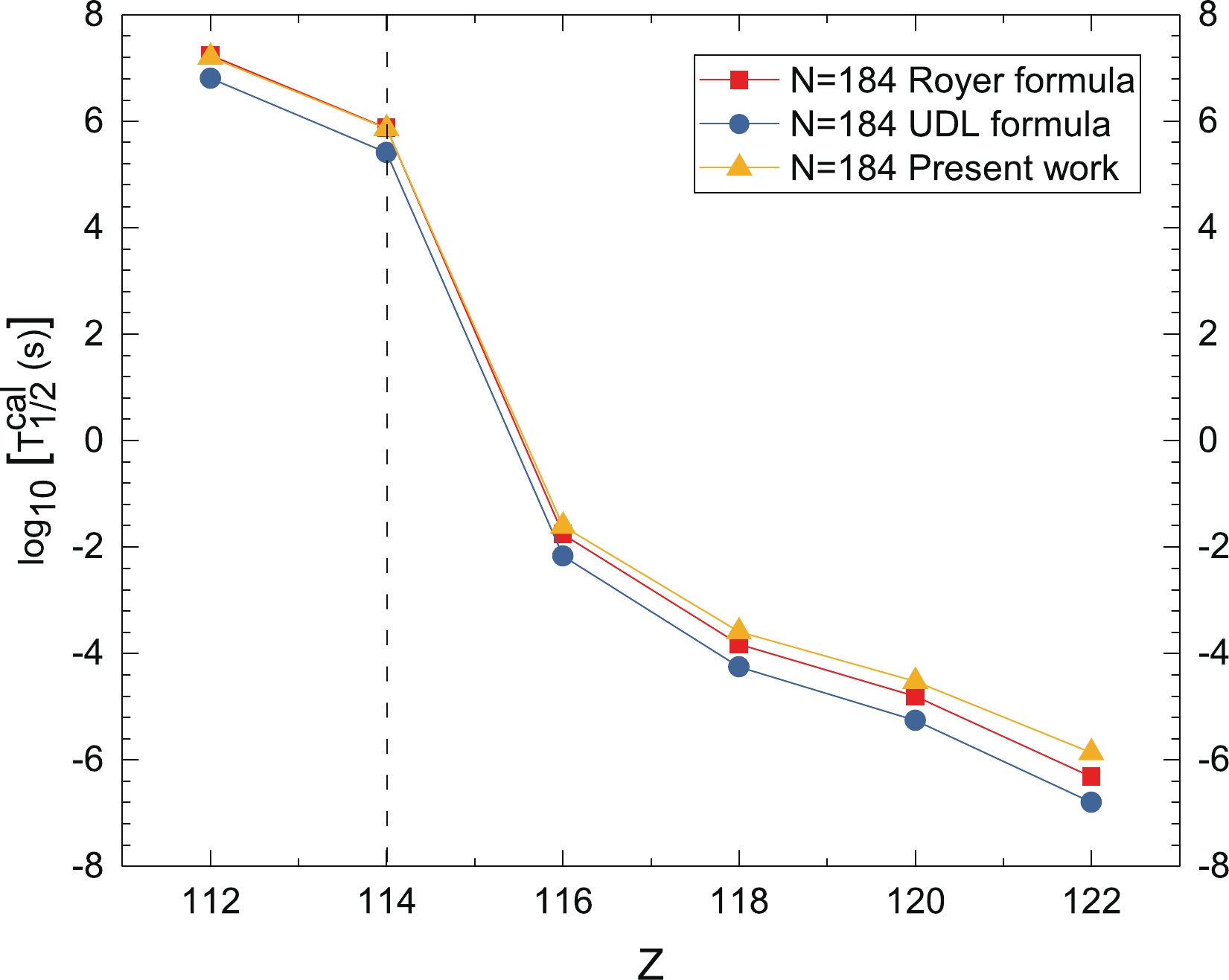