

Stable nitrogen isotope in tree rings: Progresses, problems and prospects
WANG Keyi

通讯作者:
收稿日期:2020-05-18修回日期:2021-03-12网络出版日期:2021-05-25
基金资助: |
Received:2020-05-18Revised:2021-03-12Online:2021-05-25
Fund supported: |
作者简介 About authors
王可逸(1995-), 女, 内蒙古呼和浩特人, 硕士生, 主要从事树轮稳定氮同位素方面的研究。E-mail:

摘要
关键词:
Abstract
Keywords:
PDF (2209KB)元数据多维度评价相关文章导出EndNote|Ris|Bibtex收藏本文
本文引用格式
王可逸, 刘晓宏, 曾小敏, 徐国保, 张凌楠, 李春越. 树轮稳定氮同位素记录的进展与展望. 地理学报[J], 2021, 76(5): 1193-1205 doi:10.11821/dlxb202105011
WANG Keyi, LIU Xiaohong, ZENG Xiaomin, XU Guobao, ZHANG Lingnan, LI Chunyue.
1 引言
全球气候和环境变化给人类的生活环境和生存方式带来深远影响,导致人类赖以生存的森林生态系统结构、功能和健康发生了显著变化。同时,人类活动造成全球活性氮总量翻倍,增加了大气到森林生态系统的氮沉降。从生态系统尺度来看,氮对植物的供应水平控制着生态系统的生产力[1],氮循环特征的变化也显著影响和干扰了森林生态系统功能的各个方面[2,3,4]。在氮为限制性因子的森林生态系统中,氮沉降增加的开始阶段会促进叶面积、光合速率和植物生长量的提高;随着生态系统的发展演化,氮沉降和磷素等会共同影响森林树木生长[5]。然而,氮沉降对植物氮同位素分馏的影响机理以及在长时间尺度生态系统对氮沉降、气候变化和大气CO2浓度升高的响应特征也需进一步明确[5]。尽管氮沉降变化对氮循环很多过程的影响难以准确测定,但植物和土壤稳定氮同位素比率(δ15N)变化在一定程度上可指示氮循环关键过程的变化特征[4]。因此,土壤和植物δ15N长期变化特征可用来研究难以直接测定的氮循环各个过程及其综合影响[6,7]。树木年轮以其定年准确连续性强、分辨率高、对气候环境变化敏感及复本量好等特点成为研究过去气候环境变化的首选生物代用资料,在揭示长时间尺度环境演变规律和机理方面发挥了重要作用[8,9]。保存在树轮中的氮化合物能提供十年到千年时间尺度上的植物氮吸收利用信号,成为陆地生态系统氮循环特征的可靠综合度量指标。树轮中15N与14N的相对比值(δ15N)可以作为生态系统氮循环历史变化的生物代用记录。基于树轮 δ15N重建森林生态系统氮循环的优势在于:① 以高分辨率覆盖较长的时间尺度,② 揭示良好的地理空间相关信息,③ 反映植物直接吸收氮源的同位素信号变化[10]。到目前为止,有关树轮稳定氮同位素的研究大多数集中在国外,研究区域广泛分布于北美洲、西欧、东亚、大洋洲、以及赤道附近的热带地区。关注点集中在以下4个方面:基于自然丰度变化研究、肥料施用与示踪研究、污染物排放研究以及海洋氮源追踪研究。其中,对树轮氮同位素自然丰度的研究最为集中。然而,国内基于树轮δ15N记录的相关研究成果相对较少,处于起步阶段,有必要对树轮δ15N研究方法和需考虑的问题给予关注,拓展树轮稳定同位素指标的应用范围。本文综述了基于树轮δ15N进行气候和环境演变方面的相关研究成果及其发展趋势,系统阐述了树轮δ15N的分馏机理、测试方法及需要注意的问题,并指出未来树轮δ15N研究需要着重发展的方向。
2 树轮稳定氮同位素研究方法
2.1 氮循环过程中树轮稳定氮同位素分馏
在自然界中,氮原子的稳定同位素有2种能够存在的形式:14N和15N,空气中14N和15N的相对丰度为99.6337%和0.3663%,通常以大气氮作为同位素测定的标准物质。一般稳定氮同位素的比率用千分偏差δ值来描述,其定义公式如下:式中:R为15N和14N的比值。
氮稳定同位素的分馏发生在生态系统氮循环各个过程中(图1),生态系统不同含氮化合物的氮同位素组成(15N/14N)呈现动态变化,可被用来指示生态系统中氮的来源和周转过程。利用氮稳定同位素研究氮循环过程,必须考虑氮循环各个过程中同位素分馏效应(即2种物质间同位素分馏的程度):通常以2种物质中同位素比率之商,即同位素分馏系数(αp/s);以及富集系数(εP/S)来表示,以判断分馏过程中产物与底物的同位素比值变化,定义式如下[11]:
式中:Rp为产物的氮同位素比率,Rs为底物的氮同位素比率。ε > 0表示分馏过程使产物同位素比值变高;ε < 0表示分馏过程使产物同位素比值变低,ε 一般写成‰的形式。
图1
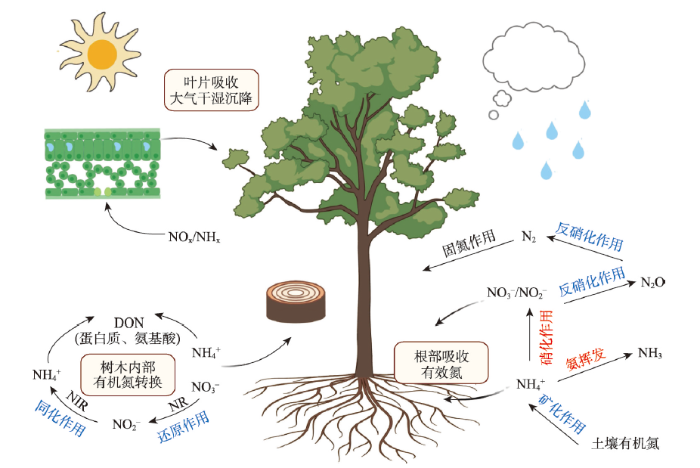
图1氮循环过程中树轮稳定氮同位素相关分馏机理
注:蓝色字体表示分馏过程中,产物氮同位素比值降低;红色字体表示分馏过程中产物氮同位素比值升高;黑色字体表示在分馏过程中,产物氮同位素比值变化不显著。
Fig. 1The fractionation mechanism of δ15N in tree rings
在氮循环过程中,生物固氮是通过专门的固氮酶来实现的,由于全球整体大气中N2的δ15N几乎没有变化[12],普遍认为自然界中生物固氮过程中氮同位素分馏作用很小[13]。通过生物固氮进入到土壤中的有机氮,在土壤中可以通过矿化和硝化等作用发生转化。许多研究揭示了根际土壤无机氮δ15N值(NO3-和NH4+)在大多数情况下比非根际土壤的δ15N值低[14,15,16],这是由于土壤微生物吸收和排泄氮的过程中,发生的同位素分馏作用造成的。生态系统氮输出主要通过气态形式(如反硝化作用、氨挥发)和液态形式(如淋溶作用、侵蚀)。相比于水文形式的氮输出,气态形式的氮输出会更大程度伴随着氮同位素分馏[17]。当NH3挥发,扩散到大气中的过程伴随着很高的分馏系数(17.9‰),相对于残留在土壤或植物中的NH4+,15N的消耗量高达40‰[18]。尽管经过反硝化作用排放到大气中的δ15N值尚未有精准的测量,但是通过生物化学分馏因子来评估N2O的同位素效应,结果显示N2O的δ15N值通常在-40‰~0之间[19,20,21],这表明反硝化过程中15N损耗很大。
植物在吸收利用土壤中的无机氮时,不同组织发生不同程度的分馏,其分馏程度取决于地面根部表层的氮浓度;当土壤氮浓度较高时,分馏作用明显(图1)。其中,矿化作用对氮同位素的分馏作用比较小,仅约2‰左右[18, 22-23],而硝化作用对氮同位素的分馏作用介于-35‰~-5‰之间[22]。此外,NO3–通过硝酸盐还原酶(NR)还原成NO2–的过程、亚硝酸盐还原酶(NIR)将NO2–还原为NH4+的过程和谷氨酰胺(Gln)合成过程也存在氮同位素的分馏,分馏作用对15N值的歧视效应(即优先吸收14N的分馏机制)在 15‰~17‰之间变化[24],这些分馏作用使未被吸收的NO3-相比植物有机氮更为富集15N [25]。
叶片可以通过气孔吸收大气中的可溶性无机氮。气态氮化合物(NOx、NHx)通过气孔进入到植物中,被转运到细胞内部,以蛋白质、氨基酸、酶等有机氮形式在植物内部迁移(图1)。Lindberg等认为干沉降是大气沉降氮输入树冠的主要形式,大气沉降中50%~70%的氮被树木冠层吸收;同时,叶片也会吸收被雨水捕获或沉降到叶表面的湿沉降氮源[26]。叶片在吸收干湿氮沉降物的过程中会发生分馏效应,通过气孔或叶表皮直接从大气中吸收气态NH3或湿沉降中的NH4+会降低叶片氮同位素组成,而分馏的程度与氮源类型直接关联,比如针叶δ15N值偏高的直接原因是叶片气孔吸收较高δ15N值的NO2[10, 27]。
此外,植物的菌根真菌对植物δ15N值也有显著影响。与非菌根植物相比,杜鹃类菌根真菌植物和外生菌根(EM)植物叶片δ15N的消耗量更大(分别为3.2‰和5.9‰)。丛枝菌根(AM)植物的同位素值处于中等水平,与非菌根植物相比平均耗竭2‰[10]。
虽然分馏作用引起δ15N值的变化会因发生器官(如根、茎、叶等)而不同,也会受物种、环境和氮源的影响[28],但树木年轮对自植物根部、冠层进入到树木内部进行循环的总有机氮均能有效记录,可作为呈现植物内部与外部氮循环总体长期变化的一个综合指标(图1),对区域尺度的森林生态系统氮循环开放程度亦有一定指示作用。
2.2 树轮稳定氮同位素比率测定方法
随着稳定同位素分析技术的进步,基于连续流分析的稳定同位素质谱仪实现了无机化合物中多种元素(C、H、O、N)的自动分析,获取可靠δ15N值所需的样品氮总量降低到约20~25 μg。树轮δ15N测定一般采用固体全木样品,借助元素分析仪—气体稳定同位素比质谱联用(EA-IRMS)系统来进行测量,不仅可以将样品自动分离与纯化,缩短样品分析时间,而且精度也接近传统的双路进样同位素质谱仪。在木材样品中氮含量较低,一般为0.05%~0.3%之间。根据氮含量的变化范围,测定自然丰度的树轮δ15N,需要更多的样品来达到气体稳定同位素比质谱仪对氮同位素比率检测信号的要求,一般所需木材干重的样品量约为10~30 mg。根据不同树种的木质特征,可以对树轮样品采取化学前处理或者不进行化学处理的方式,测定其原木样品的氮同位素比值(图2)。图2

图2树轮稳定氮同位素比例测定样品处理流程图
Fig. 2Flow-chart of pre-treatment of tree-ring material for stable nitrogen isotope analysis
在样品制备阶段,将剥离混合好的样品进行干燥、粉碎和研磨,使其均质化,有助于降低大样品量导致的不完全燃烧风险。其次,通过固体自动进样器将样品送入元素分析仪,样品中含氮物质依次经过元素分析仪的氧化炉和还原炉反应生成N2,生成的N2 通过万用接口进入到气体同位素比质谱仪进行测定,得到15N/14N比值。此外,大量样品燃烧产生的其他含碳产物(例如CO、CO2等)需要在进入质谱仪前被捕获并完全去除。一般采用大容量的CO2捕捉收集管进行,以避免频繁更换捕捉剂对质谱本底值产生影响。
在测得树轮δ15N之后,需要用一个普遍接受的有机氮同位素分析的木材标准进行校准。美国地质调查局(USGS)制备了3种粉末状的全木参考标准:来源于美国黑松(Pinus contorta)的USGS 54((-2.42±0.32)‰)、墨西哥破布木(Cordia cf.dodecandra)的USGS 55((-0.27±0.36)‰)和南非红象牙木(Berchemia cf.zeyheri)的USGS 56 ((1.85±0.37)‰)[29,30]。由于3种标准材料制备量有限,且价格较高,无法满足长期大量的校准工作,有必要在实验室制备一定量的木材实验室工作标准,并通过通用标准的校准使测得的树轮δ15N值与其他序列得到真正的比较。树轮δ15N样品准备和测试流程见图2。
2.3 树轮稳定氮同位素比率测定问题考虑
2.3.1 不同区域树种木质影响 树轮稳定氮同位素相关文章涉及到硬质木与软质木。有研究表明,单一木质特征树种的生长生理特征会对森林氮循环有个体影响[31]。因此,在树轮样芯采集时,应尽可能对林分内混合分布的多个木质特征的树种进行采样,避免在进行氮循环空间尺度分析时,单一树种个体生理趋势的影响。对不同木质特征的多个树种在洲际尺度上进行研究,其树轮稳定氮同位素比值所呈现的长期变化趋势整体一致,但区域样点间存在差异。北美洲地区研究主要集中于美国和加拿大的温带森林中,树木质地多为软木,多个树种树轮δ15N在该区域内呈现下降趋势,指示该区域较为封闭的氮循环特征[31,32,33,34,35,36](图3a);东亚地区的相关研究也皆为软木,但是不同树种在不同站点的树轮 δ15N变化趋势差异较大[7, 37-40],在一定程度上可能反映样点局地环境与人为活动的影响(图3b);欧洲地区的相关研究集中分布在地中海沿岸,包含软木与硬木,两种木质类型的树种树轮δ15N均表现出下降趋势[41,42,43](图3c);热带地区广泛研究了赤道附近分布的硬木树种,涉及北半球与南半球的8个树种,绝大多数树轮δ15N呈现为上升趋势[44,45,46](图3d),表明热带地区相对其他研究区域,氮循环系统更为开放。此外,在热带森林中,由于异养土壤微生物和豆科植物根瘤菌的高固氮率,天然氮的有效性比大多数温带森林要高,将豆科植物作为树轮δ15N的研究对象,有助于理解热带地区氮循环特征的变化[45]。不同区域的树种和木质特征存在差异,含氮化合物尤其是抽提物含量上存在差异,在验证树轮δ15N对空间氮循环的指示作用时,应针对不同树种的木材特征,采取不同的样品前处理方式或进行对比分析。图3
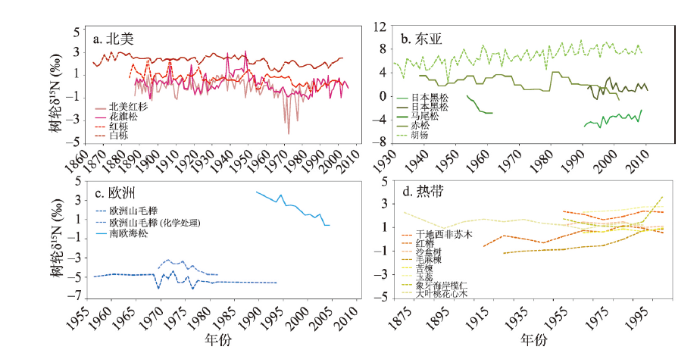
图3全球多树种树轮稳定氮同位素比率变化趋势比较
注:a图数据来源[32, 50]、b图数据来源[7, 37-40]、c图数据来源[42-43]、d图数据来源[44-46];图中虚线表示硬质木,实线表示软质木。
Fig. 3Comparison in the regional trends of global tree-ring δ15N investigations
2.3.2 氮在年轮中的径向迁移 研究表明,年轮氮含量相对较低,从心材到边材树轮氮含量都较为恒定,在最新形成的年轮中达到高值[34]。也有研究表明,树轮氮含量在边材和心材的交界处有明显的上升趋势[46],这种变化在很多类型的森林生态系统研究中均有报道。针对多样化的研究结果,****们从不同角度做出解释,但较为普遍的认知是:树轮中细胞壁的碳基结构上所包含的结构性蛋白质,是树轮氮的主要研究对象[47]。氮含量较为恒定的趋势是树木生理迁移过程的结果,不会受到样品处理方法和生态系统氮有效性的影响。因此,认为树轮氮含量记录的是在树木成熟过程中有机氮的再分配利用,作为环境中氮可利用性的代用指标时具有局限性[48]。
然而,研究发现树轮心材和边材边界处的δ15N并没有明显的变化[27, 40],也不存在明显分馏作用[48]。主要由于树轮稳定氮同位素记录了生长环境中氮源的变化,不会直接记录生理迁移作用,是环境氮有效性变化的可信指标[49,50,51]。为更好的反映树轮结构性氮同位素的记录作用,排除非结构性氮的影响,建议利用树轮稳定氮同位素探索环境影响的长期作用时,需判定高氮含量的非结构性潜在影响,若存在一定影响则需移除非结构性氮[39]。此外,为验证不同氮含量树种的迁移作用,建议将样芯分为心材、边材、心边材交界3个部分,并将每部分的年轮细分为更高分辨率的季节年轮来验证其科学性。
2.3.3 样品混合与化学前处理 对生长在高海拔或高纬地区的年轮相对较窄的树木来说,在树轮δ15N测定时需要采取多样芯混合的方式来获取足够的样本量进行δ15N分析。常见的混合方式有3种:① 将同一树木不同方向相同生长年份的样品进行混合[50]。由于受到树种个体生理特征以及树木微生境等的影响,这种混合方式对于研究区域环境氮循环代表性较弱。② 根据具体实验目的,将同一树轮样芯的多个相邻年轮进行样品混合[34, 48, 51]。此方式混合既不利于对氮径向迁移的研究,也掩盖了树轮作为氮循环综合指标的高分辨率(年)优势。③ 将同一树种多棵树木相同生长年份的样品进行混合[52,53,54]。不同混合方式对获取的树轮δ15N序列的环境代表性和时间分辨率的影响不同,在对年轮δ15N时空变化进行分析时需要考虑潜在偏差。研究森林生态系统氮循环长期动态变化,高分辨率的δ15N不是必需的,但是年分辨率的δ15N可以在一定程度解译高频气候变化或者其他干扰事件的影响[52]。因此,建议在条件允许时,尽量将相同采样点同一树种的年轮样品按高分辨率进行混合。
树轮中不存在易于识别和纯化的含氮化合物,也尚未有专门的研究测试木材中哪些氮化合物可以从树轮中去除或保留。研究表明,储存在结构化合物(细胞壁中的纤维素和木质素)上的蛋白质中的氮将被保留,而非结构化合物(单宁、多酚、脂肪、蜡、树脂,生物碱)通过萃取去除[53,54]。对于树轮δ15N研究来说,仅结构性氮代表生态系统的氮有效性水平,但会受到不稳定的迁移氮的潜在影响。因此,移除年轮样品中不稳定性氮后得到的年轮δ15N序列可以真实反映森林生态系统氮有效性的变化历史[54]。Sheppard等最早提出的树轮δ15N分析前处理方法可有效去除可迁移的不稳定氮的影响,保留结构性氮,但该处理流程大大降低了样品中氮含量水平,需要更多的样品来满足仪器检测需要的氮同位素信号强度[53]。进一步的研究对Sheppard等提出方法进行修正和简化来提高样品处理效率[39]。改进的前处理方法表明在氮输入较高的生态系统,对不稳定的迁移性氮的去除是必须的。也有研究表明,对树脂含量高的树种也需要进行前处理移除树脂的影响[48]。因此,对于不同氮水平的生态系统来说,受树种影响,边材和心材之间的氮迁移结果存在差异,需要采取不同的前处理方法[31]。在未来研究中,需要根据研究树种、样点环境和研究目的来确定是否和如何对样品进行前处理。建议在不明确化学处理是否可以去除生理驱动模式时,对树木年轮样品不进行化学处理,或者是用极性较大的去离子水做简单去除。
3 树轮稳定氮同位素记录研究进展
3.1 树轮δ15N与气候变化
随着植物和土壤δ15N数据的积累,对δ15N变化模式的解释随着时间推移而发生了变化,使得人们对氮循环参数如何沿气候梯度变化产生了一些困惑。Handley等首次尝试广泛地探究了气候与植物δ15N之间的综合关系,发现97个站点的叶片δ15N随降水的增加呈线性下降;虽然他们发现纬度对叶面δ15N没有影响,但是热带森林叶片平均δ15N比温带叶片平均高6.5‰[55]。有****认为这是因为相对于其他生态系统的氮循环,热带森林的氮循环更开放,氮的输入和输出都很大[56];也有****认为树轮δ15N在热带森林系统有更高的值是因为湿热的环境下,氮沉降和氮损失量更大[44]。这些研究将植物δ15N的升高与湿热的气候条件建立起良好关系。尽管如此,气候变量对树轮δ15N值的具体影响尚不一致或不明确[57,58]。研究表明,生长季降水和春夏季温度对年轮δ15N的影响作用是相反的[45]。也有研究表明,树轮δ15N与生长季降水量呈负或正相关,与生长季温度也呈负相关,但这种相关关系并未存在于所有研究样点[52, 59],这意味着气温、降水等气候因素对树轮δ15N的影响并未形成明晰且一致的认识,可能主要是通过影响土壤氮矿化过程和植物可利用氮水平来改变树轮中的δ15N 信号[55,56]。此外,在一些区域,树轮δ15N与研究区域的土壤生产力显著相关,表明研究地点生境特性对树轮δ15N的驱动力大于气候作用。
普遍认为,气候变化或升高的大气CO2浓度会通过影响菌根活动和改变土壤氮循环等改变植物δ15N值[59],气候变化导致的土壤温度和pH值、菌根活动、土壤氮转化(氨化、硝化)相对速率的变化共同影响了树轮δ15N值。但是,气候变量仅是通过影响氮循环分馏过程来影响平均值,还是会影响年轮δ15N的时间变化趋势,有待进一步验证[59]。要阐明哪些气候变量显著影响年轮δ15N,其他因素如何交互影响这些关系,尚需进一步研究。对以上问题的回答对理解长时间尺度上气候变化如何影响森林生态系统氮循环特征及制定科学森林管理政策至关重要。
3.2 树轮δ15N与人类活动氮排放
20世纪树轮δ15N长时间变化趋势与人类活动有关。人为活动显著影响了森林生态系统的氮循环过程。在全球范围内,人为氮沉降量等于自然氮固定率[60],但是在区域范围内,氮沉降总量差异很大,导致区域氮污染物的δ15N值变化也很大,变化范围约达20‰。不同的氮源产生的氮氧化物δ15N值变化范围不同,车辆废气排放物的δ15N在-13‰~-2‰之间,燃煤锅炉排放物的在6‰~13‰之间[61],农业生产活动产生的硝态氮化合物排放物在-5‰~10‰之间[62]。车辆尾气中氮排放量增加会增加δ15N排放量,从而导致树轮δ15N的升高[63,64],而树轮δ15N的降低也是由于排气中氮氧化物具有较低的δ15N导致[31,32]。有研究提供了分析时间段内研究区域直接排放氮氧化物的δ15N[63],以说明区域氮氧化物排放水平与树轮δ15N的直接关系。Savard等[32]和Doucet等[31]的研究给出了区域氮氧化物排放量,但没有氮沉积物的δ15N值,缺乏直接的氮沉降同位素特征以区别于生物地球化学系统内部循环特征[64],也缺少区域NOx排放或氮沉积数据来验证树轮δ15N的直接来源。此外,将靠近交通要道的树轮δ15N值与增加的汽车数量建立相关性,可作为树轮δ15N指示车辆排放污染物的证据,但仍然需要通过获取汽车尾气NOx排放的同位素信号值加以验证[31]。尽管这些研究都可能准确地报告树轮δ15N记录的污染效应,但仅Saurer等[64]提供了污染物的δ15N数据,明确支持他们的结论。为了将树轮δ15N变化趋势归因于特定外部污染源的影响,有必要获取关于当地污染源δ15N值的变化数据。仅仅对排放源或当地大气浓度的一般分析不足以得出污染沉积对树轮δ15N趋势的直接影响[10]。值得注意的是,虽然大气氮源和树轮δ15N的详细测量可能无法准确反映整个树轮生长期间的大气排放趋势[34],但是在近期的短时间尺度上,树轮δ15N的时间序列和人为排放的氮沉降之间存在潜在关系。
农业生产活动是另一种重要的人为干扰生态系统氮循环的模式。在农业活动中,长期施用氮肥,使土壤中氮素含量较高,植物从土壤中吸收和利用铵态氮和硝态氮,使得植物体内的含氮化合物增加,从而影响植物(树轮)δ15N。在中国森林地区通过示踪的方式进行同位素研究,发现δ15N有记录农业肥料施用的能力[65]。也有研究通过施用大量氮肥,量化生态系统中不同氮库之间的氮转移,并增强我们对可利用氮较高的生态系统中氮循环过程的理解。通过肥料示踪的方式模拟高氮肥输入的农业活动,有助于解释树木吸收的无机氮是如何沉积在木材组织中的,特别是含氮化合物跨年轮边界的径向迁移的去向与分配[66]。氮在年轮之间的显著迁移可能掩盖或消除环境有效性氮的年际信号,因此氮在年轮中的迁移是所有树轮δ15N研究的一个关键问题。研究表明,在进行高δ15N标记的氮施加时,尽管该峰值在施肥处理后可能会延迟1~2年[67],但与氮添加年限相对应的树轮中通常会显示出一个清晰而显著的峰值[54]。这表明树轮δ15N值的变化并不直接来源于高δ15N标记的氮施加,而是通过吸收利用土壤有效氮,并在氮进入植物的过程中发生分馏。
通过示踪的方式测定树轮δ15N值有利于了解氮循环中分馏过程对树轮δ15N的影响机制,但是会掩盖树轮在百年尺度甚至更长尺度上对于农业活动影响氮循环的记录。中国是历史悠久、人口众多的农业大国,国内树轮氮同位素研究可以结合自然丰度法与示踪方式,多维度地了解生态系统氮循环过程的变化。
4 问题和展望
树轮稳定氮同位素比率在反映多时间尺度生态系统氮循环信息方面具有巨大潜力。有助于评估全球或区域环境变化,特别是不同人类活动强度影响下森林生态系统的氮循环变化。现有研究主要集中于报道树轮δ15N对气候变化、污染物排放的记录作用,但在跨区域研究中如何对比树轮δ15N的指示效应、深化耦合多个树轮同位素指标等问题还有待进一步挖掘。此外,优化和综合利用多树种δ15N指标,将树轮δ15N应用于火灾、间伐等长时间尺度森林动态研究中,有利于理解干扰事件对森林生态系统氮循环的影响。在未来研究中,应从以下几个方面着手解决与加强。4.1 加强不同树种氮同位素样品的前处理方法研究
目前,不同木质特征树种的氮同位素特征研究广泛存在,对于不同树芯样品的前处理方式也进行了多种尝试,但是仍未得出一致或统一的样品处理方式与结论。问题在于① 受实验测试仪器限制,样品处理的混合模式易掩盖树轮年际与个体生理特征;② 对树轮中非结构性含氮化合物认知的缺乏;③ 对相邻树轮之间迁移和不同组织之间氮同位素分馏的具体机制了解较少。因此,针对不同树种和不同研究目的与要求,应加强对树轮非结构氮化合物的了解,探究氮同位素记录在植物组织之间的分馏与内部径向迁移机制。基于树种木材组成成分特征,采取相对应的样品混合与化学前处理方法。4.2 深化多稳定同位素指标耦合的探究
树轮δ13C和δ18O已用来评估树木生长对CO2浓度变化、气候因子变化的响应。不同类型树轮的δ13C、δ18O和δD反映的生理与生态环境信息也不同[68,69,70]。考虑到不同指标记录气候环境信息的互补性,在树轮稳定同位素气候和环境研究中,综合多种同位素代用指标已成为一种趋势[68, 71-73]。因此,需结合多个树轮稳定同位素代用指标,相互补充,获得更丰富的、详尽的生物地球化学循环变化资料,提高评估全球变化对森林树木生长、碳氮耦合循环以及污染物来源的准确性(图4)。图4
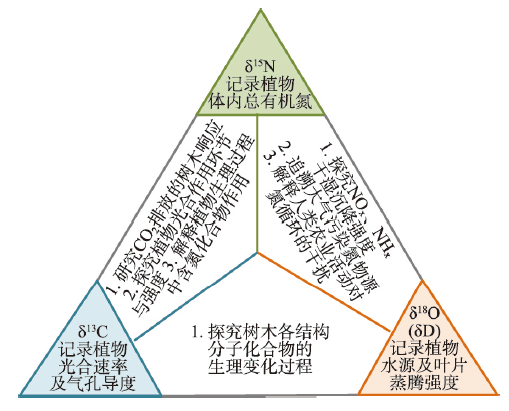
图4树轮多指标同位素耦合分析框架图
Fig. 4The concept diagram of coupling of multiple stable isotope proxies in tree rings
4.3 拓展多来源氮同位素的对比研究
全球树轮稳定氮同位素的研究集中分布在具有独特特征的森林生态系统,代表着特定区域氮循环的变化,借助大气排放的氮同位素值、土壤沉积的氮同位素比率、河流海洋传输的氮同位素比率等其他指标,也可以直接验证树轮稳定氮同位素记录信息的直接性与准确性。中国****对于氮同位素的研究已经深入到各个圈层的多个载体中,比如大气、降水、植物叶片、土壤、海洋沉积物、冰芯以及地下水等[63, 74-78],这类研究主要集中于中国东部以及华北等人类活动较频繁的地区。这些研究丰富了氮同位素记录的氮循环相关变化信息,反映了中国的氮循环系统相对较为开放;然而利用树轮稳定氮同位素来探究区域氮循环过程的研究较少。加强区域不同载体氮同位素信息的对比,可以从不同角度剖析全球变化和人类活动对氮可利用性的影响机制和过程。
参考文献 原文顺序
文献年度倒序
文中引用次数倒序
被引期刊影响因子
DOI:10.1111/gcb.12281PMID:23744637 [本文引用: 1]

Projections of future changes in land carbon (C) storage using biogeochemical models depend on accurately modeling the interactions between the C and nitrogen (N) cycles. Here, we present a framework for analyzing N limitation in global biogeochemical models to explore how C-N interactions of current models compare to field observations, identify the processes causing model divergence, and identify future observation and experiment needs. We used a set of N-fertilization simulations from two global biogeochemical models (CLM-CN and O-CN) that use different approaches to modeling C-N interactions. On the global scale, net primary productivity (NPP) in the CLM-CN model was substantially more responsive to N fertilization than in the O-CN model. The most striking difference between the two models occurred for humid tropical forests, where the CLM-CN simulated a 62% increase in NPP at high N addition levels (30 g N m(-2) yr(-1)), while the O-CN predicted a 2% decrease in NPP due to N fertilization increasing plant respiration more than photosynthesis. Across 35 temperate and boreal forest sites with field N-fertilization experiments, we show that the CLM-CN simulated a 46% increase in aboveground NPP in response to N, which exceeded the observed increase of 25%. In contrast, the O-CN only simulated a 6% increase in aboveground NPP at the N-fertilization sites. Despite the small response of NPP to N fertilization, the O-CN model accurately simulated ecosystem retention of N and the fate of added N to vegetation when compared to empirical (15) N tracer application studies. In contrast, the CLM-CN predicted lower total ecosystem N retention and partitioned more losses to volatilization than estimated from observed N budgets of small catchments. These results point to the need for model improvements in both models in order to enhance the accuracy with which global C-N cycle feedbacks are simulated.? 2013 John Wiley & Sons Ltd.
[本文引用: 1]
[本文引用: 1]
DOI:http://www.geog.com.cn/article/2021/0375-5444/10.1098/rstb.2013.0119URL [本文引用: 1]
[本文引用: 2]
URL [本文引用: 2]
DOI:10.1111/nph.2009.183.issue-4URL [本文引用: 1]
DOI:10.1007/s11270-005-2253-yURL [本文引用: 3]
DOI:10.1038/nature04743URL [本文引用: 1]
DOI:10.1016/j.quascirev.2010.05.005URL [本文引用: 1]
DOI:http://www.geog.com.cn/article/2021/0375-5444/10.1007/s10533-014-9988-8URL [本文引用: 4]
[本文引用: 1]
DOI:10.1104/pp.69.4.880URL [本文引用: 1]
DOI:10.1111/nph.2012.196.issue-2URL [本文引用: 1]
DOI:10.1080/03067319208027017URL [本文引用: 1]
DOI:10.2136/sssaj1985.03615995004900020034xURL [本文引用: 1]
DOI:10.1002/rcm.4648PMID:20740523 [本文引用: 1]

We investigated the delta(15)N profile of N (extractable NH(4)(+), NO(3)(-), and organic N (EON)) in the soil of a N-saturated subtropical forest. The order of delta(15)N in the soil was EON > NH(4)(+) > NO(3)(-). Although the delta(15)N of EON had been expected to be similar to that of bulk soil N, it was higher than that of bulk soil N by 5 per thousand. The difference in delta(15)N between bulk soil N and EON (Delta(15)N(bulk-EON)) was correlated significantly with the soil C/N ratio. This correlation implies that carbon availability, which determines the balance between N assimilation and dissimilation of soil microbes, is responsible for the high delta(15)N of EON, as in the case of soil microbial biomass delta(15)N. A thorough delta(15)N survey of available N (NH(4)(+), NO(3)(-), and EON) in the soil profiles from the organic layer to 100 cm depth revealed that the delta(15)N of the available N forms did not fully overlap with the delta(15)N of plants. This mismatch in delta(15)N between that of available N and that of plants reflects apparent isotopic fractionation during N uptake by plants, emphasizing the high N availability in this N-saturated forest.Copyright 2010 John Wiley & Sons, Ltd.
DOI:10.1073/pnas.0912111106URL [本文引用: 1]
DOI:10.1046/j.1469-8137.1997.00808.xURL [本文引用: 2]
URL [本文引用: 1]
DOI:10.1007/s11104-007-9264-yURL [本文引用: 1]
DOI:10.1002/(ISSN)1097-0231URL [本文引用: 1]
DOI:10.1016/j.scitotenv.2017.10.086URL [本文引用: 2]
DOI:10.1016/j.gca.2012.11.048URL [本文引用: 1]
DOI:10.1111/jpy.2004.40.issue-3URL [本文引用: 1]
DOI:10.1093/jxb/49.320.521URL [本文引用: 1]
DOI:10.1126/science.231.4734.141URL [本文引用: 1]
DOI:http://www.geog.com.cn/article/2021/0375-5444/10.1038/s41598-017-08156-xURL [本文引用: 2]
DOI:10.1007/s004250050336URL [本文引用: 1]
DOI:10.1016/j.chemgeo.2016.07.017URL [本文引用: 1]
DOI:10.1016/j.chemgeo.2012.05.011URL [本文引用: 1]
DOI:10.1023/B:BIOG.0000015786.65466.f5URL [本文引用: 6]
DOI:10.1021/es802437kURL [本文引用: 4]
DOI:10.5194/bg-9-867-2012URL [本文引用: 1]
DOI:10.1021/es050733pURL [本文引用: 4]
DOI:10.1007/s10021-013-9692-xURL [本文引用: 1]
DOI:10.1016/j.foreco.2011.09.033URL [本文引用: 1]
DOI:10.1016/j.apgeochem.2010.08.017URL [本文引用: 2]
DOI:10.1002/rcm.5227PMID:22006393 [本文引用: 2]

Temporal changes in the acquisition of nitrogen (N) are recorded in tree-rings together with unique N isotopic values. Some debate continues regarding the importance of wood pre-treatment in isotope analysis and, thus, this study focuses on the removal of labile components to determine the intrinsic nature of N in tree-rings. The total concentration and stable isotopic value of N in annual tree-rings were determined for two cores from Japanese black pine (Pinus thunbergii) from areas colonized by black cormorant (Phalacrocorax carbo). One core sample was also collected from a control site, without cormorants. Sharp increases in tree-ring δ(15)N values associated with migration of the cormorant population indicate positive incorporation of N from soils, whereas a less pronounced trend was observed for ring samples for periods without or substantially less migration, and for those obtained from the control site. All labile N components were removed by repeated extraction with toluene/ethanol (1:1) solution. Radial translocation of labile N is limited in tree-rings from Japanese black pine, providing intrinsic records on N acquisition. The difference in N isotopic values (up to 7.0‰) following pre-treatment was statistically significant for trees affected by the avian colony, whereas the pre-treatment of the control samples did not influence N values. The implication is that in agreement with previous studies pre-treatment is not necessary when trees are exposed to natural N concentrations in the soil but the removal of enriched δ(15)N labile components is necessary when woody plants are exposed to unusually high inputs of N into soils. However, the temporal trend in tree-ring δ(15)N series of the avian N affected trees did not change. Thus, if the priority is not the value but the trend then pre-treatment is not necessary.Copyright ? 2011 John Wiley & Sons, Ltd.
DOI:http://www.geog.com.cn/article/2021/0375-5444/10.1016/j.chemgeo.2020.119666URL [本文引用: 3]
DOI:10.1007/s00468-011-0539-9URL [本文引用: 1]
DOI:10.1093/treephys/25.11.1437URL [本文引用: 2]
DOI:10.1007/s00468-010-0420-2URL [本文引用: 2]
DOI:10.1021/es901383gURL [本文引用: 3]
DOI:10.1126/science.1211979URL [本文引用: 3]
DOI:10.1890/0012-9658(2007)88[443:DATGAC]2.0.CO;2URL [本文引用: 3]
DOI:10.1073/pnas.89.14.6604URL [本文引用: 1]
DOI:10.1002/rcm.4876URL [本文引用: 4]
URL [本文引用: 1]
DOI:10.1021/es061414gURL [本文引用: 3]
DOI:10.1002/2014JG002865URL [本文引用: 2]
DOI:10.1007/s11258-012-0141-2URL [本文引用: 3]
[本文引用: 3]
DOI:10.1051/forest:2003043URL [本文引用: 4]
PMID:10407318 [本文引用: 2]

It has long been evident that plant (15)N chiefly reflects the processes which fractionate (15)N/(14)N rather than the (15)N of plant N source(s). It has emerged recently that one of the most important fractionating processes contributing to the whole plant (15)N is the presence/absence, type or species of mycorrhiza, especially when interacting with nutrient deficiency. Ecto- and ericoid mycorrhizas are frequently associated with (15)N-depleted foliar (15)N, commonly as low as -12 per thousand. As shown by the present study, plants having no mycorrhiza, or those infected with various species of arbuscular mycorrhiza (AM)-forming fungi, interact with varying concentrations of soil nitrogen [N] and moisture to enrich plant (15)N by as much as 3.5 per thousand. Hence the lack of a mycorrhiza, or variation in the species of AM-forming fungal associations, can account for about 25% of the usually reported variations of foliar (15)N found in field situations and do so by (15)N enrichment rather than depletion. Copyright 1999 John Wiley & Sons, Ltd.
[本文引用: 2]
DOI:10.1007/s12665-014-3127-7URL [本文引用: 1]
DOI:10.1016/j.advwatres.2017.02.013URL [本文引用: 1]
DOI:10.1046/j.1365-2486.2003.00679.xURL [本文引用: 3]
DOI:10.1126/science.1136674URL [本文引用: 1]
DOI:10.1007/s004420050122PMID:28307345 [本文引用: 1]

Isotopic studies of nitrogen and sulphur inputs to plant/soil systems commonly rely on limited published data for the N/N and S/S ratios of nitrate, ammonium and sulphate in rainfall. For systems with well-developed plant canopies, however, inputs of these ions from dry deposition or particulates may be more important than rainfall. The manner in which isotopic fractionation between ions and gases may lead to dry deposition and particulates having N/N or S/S ratios different from those of rainfall is considered. Data for rainfall and throughfall in coniferous plantations are then discussed, and suggest that: (1) in line with expectations, nitrate washed from the canopy has N/N ratios higher than those in rainfall; (2) the N/N ratios of ammonium washed from the canopy are variable, with high ratios being found for canopies of higher pH?in conditions of elevated ambient ammonia gas concentrations; and (3) in accord with expectations and previous work, S/S ratios of sulphate washed from the canopy are not substantially different from those in rainfall. The study suggests that if atmospheric inputs are relevant to isotopic studies of the sources of nitrogen for canopied systems, then confident interpretation will require analysis of these inputs.
DOI:http://www.geog.com.cn/article/2021/0375-5444/10.1016/j.scitotenv.2020.137242URL [本文引用: 1]
DOI:10.1016/j.atmosenv.2008.08.042URL [本文引用: 3]
DOI:10.1016/j.atmosenv.2004.02.037URL [本文引用: 3]
[本文引用: 1]
[本文引用: 1]
DOI:10.1080/1025601031000102206URL [本文引用: 1]
DOI:10.1093/treephys/28.10.1525URL [本文引用: 1]
[本文引用: 2]
[本文引用: 2]
DOI:http://www.geog.com.cn/article/2021/0375-5444/10.1007/s10584-006-9206-3URL [本文引用: 1]
DOI:10.1016/j.gloplacha.2013.02.005URL [本文引用: 1]
DOI:10.1093/treephys/tpu067URL [本文引用: 1]
DOI:10.1016/j.chemgeo.2008.01.006URL
[本文引用: 1]
[本文引用: 1]
[本文引用: 1]
[本文引用: 1]
DOI:10.1016/j.scitotenv.2018.03.261URL
DOI:10.1016/j.atmosenv.2019.06.031URL
DOI:10.1016/j.scitotenv.2017.06.088URL [本文引用: 1]