
Contribution of terrestrial carbon sink to future warming in China, the United States, Russia and Canada
CUI Yaoping
收稿日期:2019-04-2修回日期:2020-10-7网络出版日期:2021-01-25
基金资助: |
Received:2019-04-2Revised:2020-10-7Online:2021-01-25
Fund supported: |
作者简介 About authors
崔耀平(1984-), 男, 河南周口人, 教授, 博导, 研究方向为自然资源学。E-mail:

摘要
关键词:
Abstract
Keywords:
PDF (3574KB)元数据多维度评价相关文章导出EndNote|Ris|Bibtex收藏本文
本文引用格式
崔耀平, 李楠, 付一鸣, 陈良雨. 中美俄加陆域碳汇对人为增温的消减贡献. 地理学报[J], 2021, 76(1): 167-177 doi:10.11821/dlxb202101013
CUI Yaoping, LI Nan, FU Yiming, CHEN Liangyu.
1 引言
由于人类活动和自然变化的共同影响,气候变暖已成为全球不得不面对的事实。政府间气候变化专门委员会(IPCC)发布的评估报告指出,自20世纪50年代以来,人为活动所引发的温室气体(GHGs)排放在全球气温变暖过程中发挥着重要作用,其可信度超过了95%[1]。CO2作为一种最重要的温室气体,其引起的辐射强迫约占长寿命GHGs所引发辐射强迫的65%[2]。研究人为和自然因素引起的大气CO2变化对气温的影响,一方面可以评估人为和自然因素在调节气候中的重要作用,另一方面可以为分析国别间的“碳中和”、建立公平合理的碳减排分配方案提供科学依据。人为温室气体排放和陆地生态系统碳循环均受到人类社会经济活动和自然因素的共同影响。人类活动导致GHG的异常升高将导致全球变暖持续数千年[3,4]。陆地生态系统的碳源汇功能对人为活动释放的CO2具有重要的调节功能[5]。在21世纪初,全球范围内化石燃料排放的CO2约9.0 Pg C a-1 [6],而陆地生态系统年均碳汇达到1.5±0.9 Pg C a-1,高于20世纪90年代的1.1±0.9 Pg C a-1 [1, 7]。但是,不同国家或地区、不同的发展阶段、不同的人类活动强度和陆地生态系统,对全球变化的影响也会存在很大差异。
辐射强迫(Radiative Forcing, RF)是对某气候强迫因子改变“地球—大气”系统能量收支平衡影响程度的度量,同时其也是反映强迫因子作用于气温变化的一个指标[8]。IPCC也采用RF来定量评估不同自然和人为强迫因子在引起气候变化方面的强度[9]。无论是解释过去的气候变化,还是预测未来的气候变化,都有必要研究大气CO2变化引起的辐射强迫。因此,很多研究在长时间尺度下进行了RF的模拟研究[10,11,12,13]。但是Joos等研究证实,CO2在排放到大气中的头20 a对气温的扰动最为明显,随后进入稳定阶段[14]。一些研究还显示,工业革命以来CO2的辐射强迫为1.82±0.19 W m-2,但在21世纪前10年,辐射强迫就提升了0.2 W m-2 [1, 15]。因此,开展短时间尺度的对应分析亟待加强,尤其是21世纪以来,可以利用更为详实的数据来开展对应时间段的研究。
中国、美国、俄罗斯和加拿大4个国家的人为CO2排放在全球具有重要占比。2014年,4个国家化石燃料燃烧排放的CO2全球占比接近一半[16]。从历史上看,美国长期以来一直是世界上最大的人为CO2排放国[16],2006年中国的CO2排放量首次超过美国[17]。2001—2006年间,全球54%的CO2排放来自中国[18],但是作为全球主要的CO2排放国,美国、俄罗斯和加拿大的人均CO2排放量均高于中国[19]。因此,研究世界主要排放国的CO2排放的变化及其对气温的影响,对促进各国加大减排力度和实现全球应对气候变化的长期目标均具有重要意义。
本文使用CO2同化数据集研究中国、美国、俄罗斯和加拿大4个国家的人为碳排放时空分布及其对应的RF;并进一步分析陆域生物圈对人为碳排放增温的消减作用。本文关注的核心是4个国家2000—2016年间,人为和自然因素共同作用下的CO2浓度变化对全球变化的贡献。
2 数据与方法
2.1 研究区域
本文选取全球面积最大的4个国家:俄罗斯、加拿大、中国及美国为研究区,总面积占全球陆地面积的31.2%。4个国家经济发展程度和发展阶段各有不同,根据世界银行的《全球经济展望》报告,北美的美国和加拿大国家为发达经济体,而亚洲的俄罗斯和中国为新兴市场和发展中经济体[20]。2000年以来,各国经济发展速率依次为:中国>俄罗斯>加拿大>美国[21],且这个次序在本文研究时段内基本保持不变。2.2 研究数据
本文CO2数据来自美国国家海洋与大气管理局地球系统研究实验室提供的2017年版本的碳源追踪同化数据集(CT2017),“碳追踪”是一个全球大气CO2模型,旨在跟踪地球表面CO2的吸收和释放情况,其原数据空间分辨率为1°×1°,时间分辨率为月,本文处理成年进行计算和分析。本文所用的CO2通量数据包括:陆域生物圈的净生态系统碳交换量(NEE)(不包括野火)、野火燃烧以及化石燃料排放的CO2 。其中,人为碳通量(AF)主要是化石燃料燃烧释放的CO2,可以反映人类普遍的社会经济活动,而自然碳通量(NF)包括了陆地生态系统和野火燃烧的CO2通量。本文联立人为和自然CO2通量分析其对应的RF。本文所用全球月平均气温数据来自美国国家航空航天局戈达德航天研究所GISS分析的表面气温。时间跨度为2000—2016年,数据被处理成年平均温度进行计算和分析。
2.3 研究方法
单一的气候模式得到的结果具有一定的不确定性,而多模式模拟结果间往往也存在着很大的差异性。并且复杂的气候模式参数众多和各因素影响交织,不利于直接探讨碳量变化对应的气候敏感性。本文为了量化2000—2016年自然和人为因素对气候的影响,分别计算了研究区陆地生态系统、野火及化石燃料燃烧产生的CO2各自的RF;同时也计算了净RF,并将陆地生态系统和野火的RF一同归属于自然RF,而化石燃料的RF归为人为RF。设置了2种计算RF的情景:① 当年吸收或释放的CO2造成的RF(ARF),用来研究自然因素对人为碳排放增温效应的消减作用;② CO2释放到大气中不断衰减累积造成的RF(CRF),即时间积分累积的RF,用来研究人为碳排放的增温贡献。(1)年辐射强迫。本文使用Mythre等[22]提出的一种参数化方法来计算人为或自然CO2的RF:
式中:ΔARF(t)为时间t时的RF;ΔC(t)为大气CO2当年的浓度变化;C0为背景CO2浓度,5.35是关联摩尔CO2变化与辐射强迫的一个常数[23]。
需要注意的是,当陆地生态系统的NEE小于零即表现为碳汇起到降温效果时:
式中:abs为绝对值函数。
(2)累积辐射强迫。与大多数其他物质不同,CO2不会被大气中的化学反应破坏或沉积在地球表面,而是在主要的碳库如大气、海洋、陆地生态系统重新分布,涉及多个时间尺度,释放的CO2引起的初始扰动有相当一部分在大气和海洋中存在了数千年[12, 23]。大气中CO2的长期性和累积性意味着它与气候反应是耦合的,因此即使排放停止,以前排放的CO2也会继续影响气候[23,24]。
考虑到每个向大气中释放的CO2脉冲在全球碳循环的碳汇中部分消失,脉冲响应函数(IRF)可以用来描述这种变化[25]:
式中:
如果考虑气候系统关于平衡参考状态的微小扰动,可以用线性响应模型来描述气候系统对扰动强迫的响应[23, 26]。在本文中,强迫为陆地生态系统碳源、化石燃料燃烧或野火的CO2排放量,相应的气候变量是CO2浓度,因此,时间t时的大气CO2浓度可以表示为:
考虑到全球变化模式必须能够计算主要碳库大气、陆地生物圈和海洋之间人为碳的重新分布,且能模拟气候变化对CO2辐射强迫的响应,同时还应包括相关的碳—气候循环,本文采用Joos等[14]利用包括3个综合地球系统模式(HADGEM2-ES、MPI-ESM和NCAR CSM1.4)、8个中等复杂程度地球系统模式和4个箱式模式(ACC2、Bern-SAR、MAGICC和TOTEM)等集合模拟后的结果来拟合脉冲响应函数
Tab. 1
表1
表1脉冲响应函数的拟合系数
Tab. 1
i | 0 | 1 | 2 | 3 |
---|---|---|---|---|
ai | 0.2173 | 0.2240 | 0.2824 | 0.2763 |
τi | ∞ | 394.4 | 36.54 | 4.304 |
新窗口打开|下载CSV
具体计算过程中,CRF可用公式(1)表示。式(1)分别以1750年的全球CO2参考浓度278.00 ppm和2000年的369.18 ppm为背景浓度进行计算。1750年的CO2浓度可以更好地与其他****的研究进行衔接和对比;而2000年的背景浓度则可以分析在21世纪初的CO2浓度水准下,新CO2排放带来的增温作用。需要说明的是,当陆地生态系统的NEE小于零即表现为碳汇时,则不考虑衰减过程,只计算第一个年度的浓度值[23]。
最后,本文分析了自然因素对人为升温效应的消减贡献率(D):
3 结果
3.1 2000—2016年CO2通量的时空变化
CO2通量由自然和人为的通量值共同决定,4个国家在2000—2016年间碳源汇及CO2浓度趋势的空间分布如图1~图3所示。4个国家研究时段内总的CO2排放量为34.98 Pg C,陆地生态系统共吸收了28.51 Pg C。由于各国人为碳排放和生态系统均存在不同,其陆域总的碳源汇在空间上也存在较大差异。其中,中国和美国的人为碳排放量要高于加拿大、俄罗斯,分别为33.01 Pg C和22.34 Pg C,陆地生态系统分别能消减人为排放量的22.92%和20.19%。加拿大和俄罗斯人为碳排放量分别为1.55 Pg C和6.59 Pg C,而陆地生态系统所吸收的碳量要超过人为排放量,达到了3.20 Pg C和13.23 Pg C,陆域整体表现为碳汇。图1
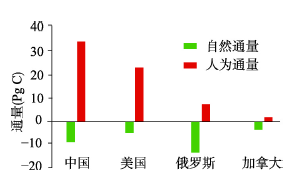
图12000—2016年中美俄加CO2总排放量
Fig.1Total CO2 emissions in China, the United States, Russia, and Canada from 2000 to 2016
图2
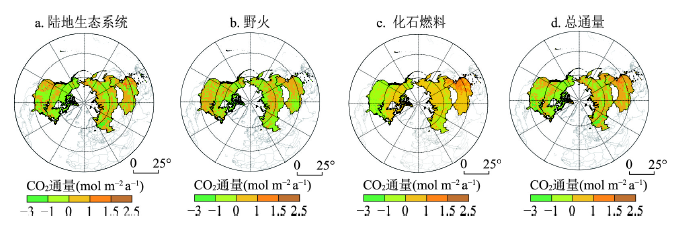
图22000—2016年中美俄加CO2通量的变化趋势
Fig. 2Trends of CO2 flux in China, the United States, Russia, and Canada from 2000 to 2016
图3
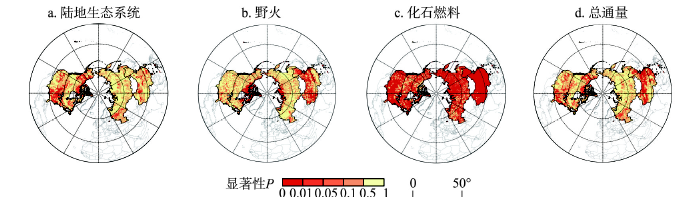
图32000—2016年中美俄加CO2通量变化趋势的显著性检验
Fig. 3Significance of the trends for CO2 flux in China, the United States, Russia, and Canada from 2000 to 2016
就变化趋势而言,2000年4个国家总CO2排放量为0.78 Pg C,2016年达2.58 Pg C。俄罗斯和中国的AF年均增速分别为0.003 Pg C a-1和0.131 Pg C a-1,增加最显著的地方出现在中国东南沿海及中部地区,最高排放量达33.81 mol m-2 a-1,而美国大部分地区呈显著下降趋势)。空间上,在加拿大北部、俄罗斯东部、中国东部及西藏等区域,虽然人为排放量不断增加,但是由于陆地生态系统吸收CO2能力在不断增强,使得该区域总排放量的增加趋势有所减缓。其中,人为化石燃料燃烧的排放具有显著的增加趋势,速率为0.125 Pg C a-1,和总的排放速率接近(0.124 Pg C a-1)(图4)。AF在研究时间段内均为负值,说明陆地生态系统总体为碳汇。其中,陆地生态系统的NEE多年平均值为-1.67 Pg C,虽有波动但仍呈现下降趋势,下降均值为0.003 Pg C a-1,说明其吸收CO2的能力在不断增强,吸收能力最强的年份为2009年,达到-2.10 Pg C。自然野火产生的CO2年际波动相对较小,但以0.002 Pg C a-1的趋势增加。
图4
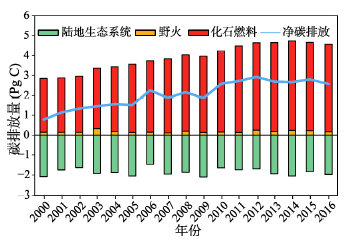
图42000—2016年中美俄加碳排放年总量
Fig. 4Annual carbon emissions in China, the United States, Russia, and Canada from 2000 to 2016
3.2 年辐射强迫的变化
考虑到CO2的气体特性及其研究区CO2的分布和CO2产生的区位分布无法对应,因此本文用浓度均值计算其辐射强迫,图5给出了辐射强迫对当年CO2通量变化的响应结果。2000—2016年间,研究区的陆地生态系统以(12.72±1.18)×10-3 W m-2 a-1的速率减缓了增温效应,同为自然因素的野火和人为因素化石燃料燃烧则分别以(1.25±0.37)×10-3 W m-2 a-1和(25.50±4.27)×10-3 W m-2 a-1的速率正向作用于变暖趋势。2000—2016年,4个国家ARF的平均值为(14.17±4.38)×10-3 W m-2(图5a)。图5
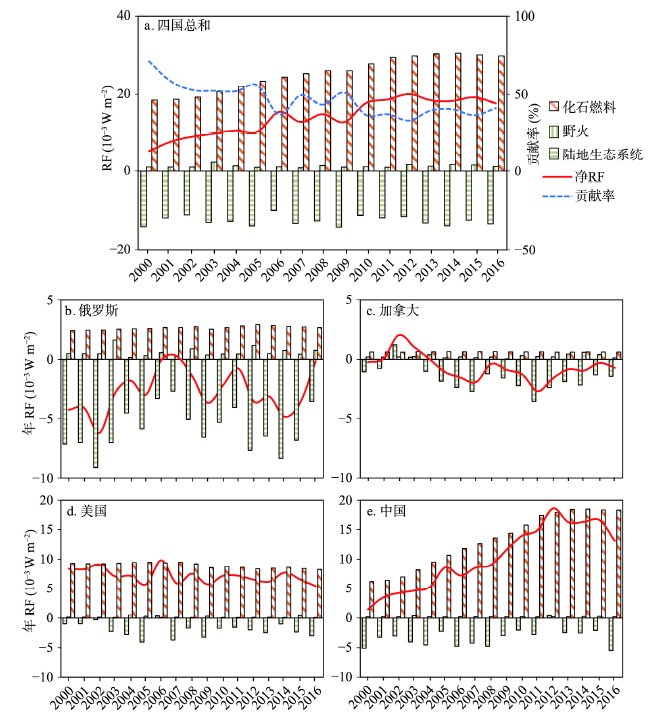
图52000—2016年四国总和及各国年辐射强迫
Fig. 5Annual dynamics of total and national radiation forcing China, the United States, Russia, and Canada, 2000-2016
俄罗斯和加拿大NF的ARF多年平均值分别为(-5.32±1.72)×10-3 W m-2和(-1.19±1.07)×10-3 W m-2,NF最小值(即陆地生态系统对CO2吸收最强)分别出现在2002年(-9.11×10-3 W m-2)和2011年(-3.54×10-3 W m-2)。两国在研究时段内NF的ARF总量高于AF,说明自然因素降温作用超过了人为碳排放的增温作用。2000年美国AF的ARF为9.28×10-3 W m-2,高于中国的6.21×10-3 W m-2。2016年,美国下降至8.32×10-3 W m-2,而中国增加到18.36×10-3 W m-2。2002—2016年,美国AF的ARF以-0.068×10-3 W m-2 a-1的速率减少,而中国则快速增加至2014年,达到峰值18.53×10-3 W m-2后下降。中国和美国的NF虽然表现为负强迫,但考虑到化石燃料燃烧,中美两国的净ARF仍以增温作用为主(图5d~5e)。而且,相较于美国净ARF较为平缓的变化速率(-0.130×10-3 W m-2 a-1),2000—2011年中国净ARF增加迅速达1.14×10-3 W m-2 a-1,之后速度减缓,2013年达到最大值。
计算结果还表明,如果仅考虑人为的化石燃料燃烧排放的CO2,则对应的辐射强迫值在2016年达到0.030 W m-2,由于陆地生态系统的碳汇功能,致使其净辐射强迫响应值为0.018 W m-2,即自然因素对大气中CO2的吸收起到了-0.012 W m-2的降温作用,消减了化石燃料燃烧41.13%。其中,陆地生态系统的RF为-0.013 W m-2,贡献率45.06%,自然野火为正强迫起增温作用,贡献率为3.93%(图5a)。
3.3 累积辐射强迫变化
依据不同的CO2大气背景浓度计算得到的CRF差异是很大的。在2000—2016年间,以2000年为本底的人为CRF和净CRF均小于以1750年为本底值,2016年,不同本底之间的人为和净CRF差值分别为0.10 W m-2和0.06 W m-2。在不考虑额外的CO2加入的情况下,2016—2036年以2000为本底的人为CRF和净CRF分别为0.24 W m-2和0.13 W m-2,较2016年分别减少了25.27%和26.32%,而以1750年为本底则分别减少了25.09%和26.22%;到2100年不同本底下的人为CRF和净CRF仍有54%~56%左右存留在大气中。但是,如果单纯考虑人为的排放,则会明显高估CO2的增温效应。如以1750年为本底时,2000—2016年间的净CRF均小于人为因素造成的CRF,2016年净CRF比人为CRF少0.18 W m-2,2036年少0.13 W m-2,2100年少0.11 W m-2(图6)。图6
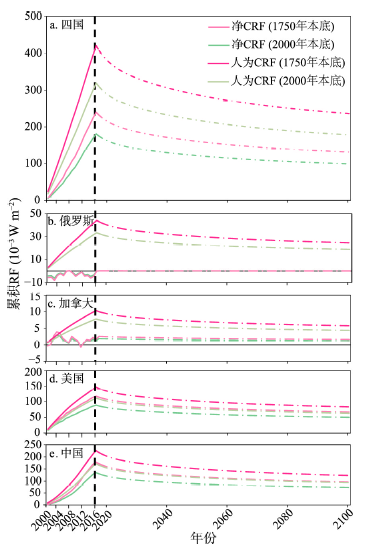
图6四国陆域的累积辐射强迫(CRF)
Fig. 6CRF of land area for China, the United States, Russia, and Canada
2000—2016年间,俄罗斯和加拿大的净CRF波动较大,俄罗斯人为CRF一直高于加拿大,但俄罗斯自然因素造成的降温效应要强于加拿大,到2016年,以2000年和1750年为本底的俄罗斯净CRF分别为0.04×10-3 W m-2和0.05×10-3 W m-2,均小于加拿大的1.28×10-3 W m-2和1.71×10-3 W m-2(图6b和图6c);而中国和美国在此研究时段内的人为CRF和净CRF均保持持续增加的趋势(图6d和图6e)。2016—2036年,在不考虑额外的CO2排放的情况下,各国在研究时段累积的辐射强迫开始呈现快速减少,然后继续缓慢下降,到2100年,还存在超过一半的辐射强迫留在大气中。
4 讨论
本文探讨全球年平均温度与CO2的净CRF、人为CRF,及自然CRF的对应关系。结果表明,人为因素即化石燃料燃烧与自然因素相比,其CRF与气温的对应关系更为密切(R = 0.54, P = 0.02)(图7b),但是,如果仅考虑陆域生态系统碳循环则无法解释对应温度(R = 0.26, P = 0.30)(图7c),而综合考虑人为和自然因素,温度和净CRF则有更大的正相关性(R = 0.55, P = 0.02)(图7a)。虽然CO2为增温的主要因子之一,但只考虑CO2的辐射强迫并不够,其他诸如CH4、N2O等温室气体[27,28,29]和生物地球物理因子如反照率、蒸散率、粗糙度长度[10, 30-33]等影响也需要一并纳入。此外,还有气溶胶、ENSO等的气候效应[34,35]也未在本文的研究范畴。尽管如此,本文得到的CO2净CRF年际波动还是与温度变化本身存在很强的相关性,对同时段气温变化的直接可解释度达到了30.3%。图7
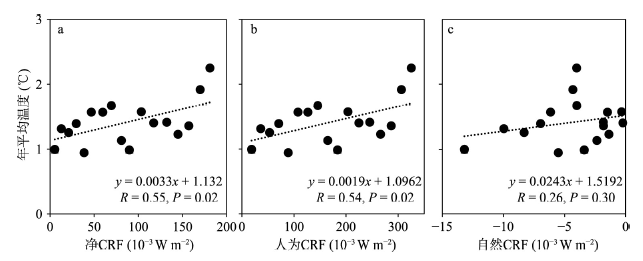
图7全球年平均温度与累计辐射强迫(CRF)的相关性
Fig. 7Relationship between global average temperature and CRF
在温室效应的研究中,大气CO2浓度加倍所产生的辐射强迫是一个十分重要的方面,其产生的辐射强迫是研究人类活动对气候影响的一个最基本的数据[36,37,38]。IPCC第二次评估报告(SAR)使用简化的表达式算得的CO2加倍产生的辐射强迫为4.37 W m-2,而IPCC第五次评估报告推荐的参数化方案考虑了不同温室气体吸收带之间的重叠效应、平流层温度的调节,以及CO2的短波辐射强迫[22, 39],最终算得CO2加倍产生的辐射强迫与SAR的结果相比减小了15%。因此,关于CO2浓度加倍产生的辐射强迫敏感性,可能会在整个气候变化研究中造成很大的不确定性。
陆地生态系统是人类社会赖以生存和发展的基础,可在各种尺度上对气候产生作用,是影响气候变化的重要因素。陆地生态系统具有碳源汇特征,例如在中国,稻田、自然湿地和旱地是造成全球变暖的重要因素;而森林、林地和草地在缓解气候变暖方面则发挥了重要作用[40]。有研究表明,中国和北美的森林面积在不断扩张[41],俄罗斯森林则是世界上最大的毗连林带,占全球森林覆盖面积的17%,在控制全球碳循环方面发挥了关键作用[42]。尽管如此,气候变暖有可能转变其碳源汇功能[43,44]。就本文的结果来看,纵然有野火的燃烧排放,陆地生态系统仍然为一个CO2的汇,且这种汇的作用还有一定的增加趋势,这可能与近年来的植被作用增强[45,46,47],及CO2浓度升高对生态系统光合作用的施肥效应有关[48]。同时,各国CO2的排放时间不同带来的气候效应不同,美国在历史上的碳排放总量一直高居榜首,中国则在近十余年才开始超过美国[17,18,19]。本文分析了4个国家陆域CO2通量的时空变化及其辐射强迫,但仅含2000—2016年,2000年以前的CO2遗留影响尚未纳入本文,可能会影响到长期积累CO2通量的增温效应[27]。
此外,本文是以各国陆域为分析单元开展的人为和自然CO2的RF效应研究,并未考虑国与国之间的碳流动及各国之间的CO2进出口贸易;也未包括大气—海洋的碳交换过程,而海洋具有很强的“汇”的功能[23, 49-50],这也是后续研究所需关注的方向。
5 结论
利用CO2同化数据集,本文分析了中国、美国、俄罗斯和加拿大在2000—2016年的自然和人为CO2通量的时空变化,分别模拟其辐射强迫,并探讨了研究时段内4个国家CO2排放总量对未来(2100年)的累积增温效应。本文有助于进一步了解人为和自然因素对气候的影响,特别是量化了陆地生态系统碳汇对人为碳排放增温的消减作用。本文结果证实了,单纯考虑人为碳排放而不考虑陆地生态系统的碳汇作用,将会明显高估人为CO2排放的增温效应。同时,考虑了人为和自然因素的净累计辐射强迫与对应时段的温度变化具有显著的相关性。并且到本世纪末,研究时段内CO2排放的辐射强迫仍有超过一半存留在大气中。
参考文献 原文顺序
文献年度倒序
文中引用次数倒序
被引期刊影响因子
[本文引用: 3]
URL [本文引用: 1]
[本文引用: 1]
[本文引用: 1]
[本文引用: 1]
[本文引用: 1]
[本文引用: 1]
URLPMID:18202646 [本文引用: 1]
[本文引用: 1]
[本文引用: 1]
[本文引用: 2]
DOI:10.1038/nclimate1346URL [本文引用: 1]
[本文引用: 2]
[本文引用: 1]
[本文引用: 3]
URLPMID:25731165 [本文引用: 1]
[本文引用: 2]
[本文引用: 2]
[本文引用: 2]
[本文引用: 2]
[本文引用: 1]
URL [本文引用: 1]
[本文引用: 2]
[D].
[本文引用: 7]
[D].
[本文引用: 7]
DOI:10.1016/j.atmosenv.2009.06.005URLPMID:32288556 [本文引用: 1]

Aviation alters the composition of the atmosphere globally and can thus drive climate change and ozone depletion. The last major international assessment of these impacts was made by the Intergovernmental Panel on Climate Change (IPCC) in 1999. Here, a comprehensive updated assessment of aviation is provided. Scientific advances since the 1999 assessment have reduced key uncertainties, sharpening the quantitative evaluation, yet the basic conclusions remain the same. The climate impact of aviation is driven by long-term impacts from CO2 emissions and shorter-term impacts from non-CO2 emissions and effects, which include the emissions of water vapour, particles and nitrogen oxides (NO x ). The present-day radiative forcing from aviation (2005) is estimated to be 55 mW m(-2) (excluding cirrus cloud enhancement), which represents some 3.5% (range 1.3-10%, 90% likelihood range) of current anthropogenic forcing, or 78 mW m(-2) including cirrus cloud enhancement, representing 4.9% of current forcing (range 2-14%, 90% likelihood range). According to two SRES-compatible scenarios, future forcings may increase by factors of 3-4 over 2000 levels, in 2050. The effects of aviation emissions of CO2 on global mean surface temperature last for many hundreds of years (in common with other sources), whilst its non-CO2 effects on temperature last for decades. Much progress has been made in the last ten years on characterizing emissions, although major uncertainties remain over the nature of particles. Emissions of NO x result in production of ozone, a climate warming gas, and the reduction of ambient methane (a cooling effect) although the overall balance is warming, based upon current understanding. These NO x emissions from current subsonic aviation do not appear to deplete stratospheric ozone. Despite the progress made on modelling aviation's impacts on tropospheric chemistry, there remains a significant spread in model results. The knowledge of aviation's impacts on cloudiness has also improved: a limited number of studies have demonstrated an increase in cirrus cloud attributable to aviation although the magnitude varies: however, these trend analyses may be impacted by satellite artefacts. The effect of aviation particles on clouds (with and without contrails) may give rise to either a positive forcing or a negative forcing: the modelling and the underlying processes are highly uncertain, although the overall effect of contrails and enhanced cloudiness is considered to be a positive forcing and could be substantial, compared with other effects. The debate over quantification of aviation impacts has also progressed towards studying potential mitigation and the technological and atmospheric tradeoffs. Current studies are still relatively immature and more work is required to determine optimal technological development paths, which is an aspect that atmospheric science has much to contribute. In terms of alternative fuels, liquid hydrogen represents a possibility and may reduce some of aviation's impacts on climate if the fuel is produced in a carbon-neutral way: such fuel is unlikely to be utilized until a 'hydrogen economy' develops. The introduction of biofuels as a means of reducing CO2 impacts represents a future possibility. However, even over and above land-use concerns and greenhouse gas budget issues, aviation fuels require strict adherence to safety standards and thus require extra processing compared with biofuels destined for other sectors, where the uptake of such fuel may be more beneficial in the first instance.
[本文引用: 1]
[本文引用: 1]
[本文引用: 2]
[本文引用: 1]
[本文引用: 1]
[本文引用: 1]
DOI:10.5194/bg-12-467-2015URL
DOI:10.1126/science.1118160URLPMID:16339443

Adding the effects of changes in land cover to the A2 and B1 transient climate simulations described in the Special Report on Emissions Scenarios (SRES) by the Intergovernmental Panel on Climate Change leads to significantly different regional climates in 2100 as compared with climates resulting from atmospheric SRES forcings alone. Agricultural expansion in the A2 scenario results in significant additional warming over the Amazon and cooling of the upper air column and nearby oceans. These and other influences on the Hadley and monsoon circulations affect extratropical climates. Agricultural expansion in the mid-latitudes produces cooling and decreases in the mean daily temperature range over many areas. The A2 scenario results in more significant change, often of opposite sign, than does the B1 scenario.
DOI:10.1175/2009JCLI3102.1URL [本文引用: 1]
[本文引用: 1]
[本文引用: 1]
DOI:10.1126/science.1144735URLPMID:17962560 [本文引用: 1]

Uncertainties in projections of future climate change have not lessened substantially in past decades. Both models and observations yield broad probability distributions for long-term increases in global mean temperature expected from the doubling of atmospheric carbon dioxide, with small but finite probabilities of very large increases. We show that the shape of these probability distributions is an inevitable and general consequence of the nature of the climate system, and we derive a simple analytic form for the shape that fits recent published distributions very well. We show that the breadth of the distribution and, in particular, the probability of large temperature increases are relatively insensitive to decreases in uncertainties associated with the underlying climate processes.
[本文引用: 1]
[本文引用: 1]
URLPMID:33340110 [本文引用: 1]

Brown adipose tissue (BAT) is an endocrine organ that contributes to thermogenesis and energy consumption. We investigated the effects of salt loading and surgical removal of whitened interscapular BAT (iBAT) on cardiac and adipose tissue pathology in DahlS.Z-Lepr(fa) /Lepr(fa) (DS/obese) rats, an animal model of metabolic syndrome (MetS). DS/obese rats were subjected to surgical removal of iBAT or sham surgery at 8 weeks of age and were provided with drinking water containing or not containing 0.3% NaCl for 4 weeks beginning at 9 weeks of age. Removal of iBAT suppressed the salt-induced exacerbation of left ventricular inflammation, fibrosis, and diastolic dysfunction, but not that of hypertension development, in DS/obese rats. Salt loading attenuated adipocyte hypertrophy but enhanced inflammation in both visceral white adipose tissue (WAT) and iBAT. Although iBAT removal did not affect visceral WAT pathology in salt-loaded DS/obese rats, it attenuated the elevation of circulating interleukin-6 levels in these animals. Downregulation of uncoupling protein-1 expression in iBAT of DS/obese rats was not affected by salt loading. Our results suggest that the conversion of iBAT to WAT-like tissue contributes to a salt-induced elevation of circulating proinflammatory cytokine levels that leads to exacerbation of cardiac pathology in this model of MetS.
[本文引用: 1]
[本文引用: 1]
DOI:10.1111/gcb.13349URLPMID:27167728 [本文引用: 1]

Forest stand age plays a major role in regulating carbon fluxes in boreal and temperate ecosystems. Young boreal forests represent a relatively small but persistent source of carbon to the atmosphere over 30 years after disturbance, while temperate forests switch from a substantial source over the first 10 years to a notable sink until they reach maturity. Russian forests are the largest contiguous forest belt in the world that accounts for 17% of the global forest cover; however, despite its critical role in controlling global carbon cycle, little is known about spatial patterns of young forest distribution across Russia as a whole, particularly before the year 2000. Here, we present a map of young (0-27 years of age) forests, where 12- to 27-year-old forests were modeled from the single-date 500 m satellite record and augmented with the 0- to 11-year-old forest map aggregated from the 30 m resolution contemporary record between 2001 and 2012. The map captures the distribution of forests with the overall accuracy exceeding 85% within three largest bioclimatic vegetation zones (northern, middle, and southern taiga), although mapping accuracy for disturbed classes was generally low (the highest of 31% for user's and producer's accuracy for the 12-27 age class and the maximum of 74% for user's and 32% for producer's accuracy for the 0-11 age class). The results show that 75.5 +/- 17.6 Mha (roughly 9%) of Russian forests were younger than 30 years of age at the end of 2012. The majority of these 47 +/- 4.7 Mha (62%) were distributed across the middle taiga bioclimatic zone. Based on the published estimates of net ecosystem production (NEP) and the produced map of young forests, this study estimates that young Russian forests represent a total sink of carbon at the rate of 1.26 Tg C yr(-1) .
[本文引用: 1]
[本文引用: 1]
[本文引用: 1]
[本文引用: 1]
URLPMID:29097731 [本文引用: 1]

The gross primary production (GPP) of vegetation in urban areas plays an important role in the study of urban ecology. It is difficult however, to accurately estimate GPP in urban areas, mostly due to the complexity of impervious land surfaces, buildings, vegetation, and management. Recently, we used the Vegetation Photosynthesis Model (VPM), climate data, and satellite images to estimate the GPP of terrestrial ecosystems including urban areas. Here, we report VPM-based GPP (GPPvpm) estimates for the world's ten most populous megacities during 2000-2014. The seasonal dynamics of GPPvpm during 2007-2014 in the ten megacities track well that of the solar-induced chlorophyll fluorescence (SIF) data from GOME-2 at 0.5 degrees x 0.5 degrees resolution. Annual GPPvpm during 2000-2014 also shows substantial variation among the ten megacities, and year-to-year trends show increases, no change, and decreases. Urban expansion and vegetation collectively impact GPP variations in these megacities. The results of this study demonstrate the potential of a satellite-based vegetation photosynthesis model for diagnostic studies of GPP and the terrestrial carbon cycle in urban areas.
[本文引用: 1]
URLPMID:29064464 [本文引用: 1]
DOI:10.3354/cr028011URL [本文引用: 1]
[本文引用: 1]
[本文引用: 1]