
Mechanism of barrier river reaches in the middle and lower reaches of the Yangtze River
YOUXingying
收稿日期:2016-08-15
修回日期:2016-12-7
网络出版日期:2017-07-12
版权声明:2017《地理学报》编辑部本文是开放获取期刊文献,在以下情况下可以自由使用:学术研究、学术交流、科研教学等,但不允许用于商业目的.
基金资助:
作者简介:
-->
展开
摘要
关键词:
Abstract
Keywords:
-->0
PDF (3075KB)元数据多维度评价相关文章收藏文章
本文引用格式导出EndNoteRisBibtex收藏本文-->
1 引言
长江中下游干流河道素有“黄金水道”之美誉,是“长江经济带”主轴,其河势稳定不仅关系长江中下游防洪安全、航运畅通、水土资源利用等诸多方面,更事关沿江两岸经济社会发展,稳定中下游河势的意义十分重大。长江中下游沙市—大通河段全长约991 km,单一河道与分汊河道相间分布,长度分别为504.8 km和486.2 km,占河段总长的50.9%和49.1%。分汊河道河岸抗冲性较差,河道宽浅,河势变化相对剧烈,其中,鹅头型汊道一般遵循“边滩切割、心滩形成→心滩发展壮大、逐渐平移→归并消亡”的演变规律,如陆溪口、罗湖洲汊道[1]等,顺直和微弯型汊道演变规律主要表现为主支汊交替,如新堤夹、天兴洲、戴家洲汊道[2]等;单一顺直段一般遵循“交错边滩此消彼长、过渡段上提下移”的演变规律[3],但主流摆动幅度不如分汊河道大;单一微弯段凹岸大多已被守护,“凹冲凸淤”演变规律已不甚明显。正因为分汊河道的河势变化剧烈,对防洪、航运等影响较大,水利、交通等部门对分汊河道进行了大量研究[1-6]。由于分汊河道与单一河道交错分布的特点,大量实测资料分析表明,有些汊道段,河势调整通过单一河段后会继续向下游传递,如马鞍山河段[5]、天兴洲河段[6],这使得单一河段以下河道演变也受到上游河势调整的影响,无疑增加了下游河道演变趋势预测及整治工程布置的难度;而有些汊道段,河势调整通过单一河段后不再向下游传递,如龙口河段[2],河道演变影响因素相对单一,整治工程布置相对简单。因此,对单一河道,尤其是能阻隔上游河势调整向下游传递的单一河道进行研究,是十分必要的。文献[7]在系统总结分析长江中下游长河段长时段河道演变规律基础上,提出能够阻止河势调整向下游传递的阻隔性河段,并分析了阻隔性河段的基本特征,但文献[7]在阻隔性河段的控制要素方面研究不足,使阻隔性河段判别经验性较强;同时对阻隔河势传递的内在机理研究不够深入,限制了其在长江中下游阻隔性河段的塑造及维护等方面的应用。本文在文献[7]的基础上,进一步分析阻隔性河段形成的控制要素,通过推导河湾水流动力轴线弯曲半径理论公式,明确各控制要素对形成阻隔性河段的作用,剖析阻隔性河段作用机理,为长江中下游河道治理提供重要参考。
2 研究方法和数据来源
2.1 研究方法
本文研究对象为长江中下游沙市—大通河段,河床组成主要为沙质,两岸大多为二元结构,部分岸段存在抗冲性较强的节点。沙市—城陵矶河段右岸有松滋、太平、藕池三口分荆江水沙入洞庭湖,三口和湘、资、沅、澧四水的水沙经洞庭湖调蓄后于城陵矶汇入长江,分布有沙市、监利水文站。城陵矶—武汉河段左岸有汉江入汇,分布有螺山、汉口水文站;武汉—大通河段右岸有鄱阳湖入汇,分布有湖口、大通水文站。本文研究方法有两种:一是利用实测水文地形地质及遥感资料统计分析法,获取控制要素值,具体为:利用最新航空遥感测图,直接量取各河段的河湾曲率半径、节点位置及突出岸线长度等要素;采用2011年、2013年等年份实测河道地形断面数据计算各河段的典型断面河相系数、平均纵比降等要素;根据2003-2009年床沙资料统计各河段床沙中值粒径作为河底抗冲性要素,总结各河段平滩水位至深泓范围内凹岸岸坡地质结构组成,并根据物理力学性质试验成果统计各土层的黏粒含量,再按各土层厚度进行加权平均计算,得到河岸抗冲性要素。通过上述方法,从平面、横断面、纵剖面以及河岸河床抗冲性等方面对比分析阻隔性河段和非阻隔性河段的异同,剖析阻隔性控制要素。
二是利用数学方法,从Navier-Stokes方程出发,推导河湾水流动力轴线弯曲半径的半经验半理论公式,延伸出主流摆动力与边界约束力对比关系,验证上述各控制要素对阻隔性河段的作用,结合实测水文地形地质资料,剖析阻隔性河段形成机理。
2.2 数据来源
航空遥感测图来源于USGS提供的Landsat卫星数字产品中2016年最新测图(https://www.usgs.gov/中文镜像网站为http://www.gscloud.cn/);实测河道断面地形数据由武汉大学提供;岸坡地质结构及黏粒含量来源于湖北省水利水电规划勘测设计院在1998-2002年编制的长江中游各堤段的地质勘察报告;床沙资料由交通运输部天津水运工程科学研究所提供。选取的34个单一河段在沙市—城陵矶、城陵矶—武汉、武汉—湖口、湖口—大通河段均有分布,既有单一顺直河段,又有单一微弯河段,具有较强的代表性,其基本情况及阻隔性控制要素值如表1所示。Tab. 1
表1
表1研究河段基本情况及阻隔性控制要素
Tab. 1The basic conditions and the control factors of the barrier properties of the study river reaches
序号 | 河段名称 | 河段长度(km) | 距宜昌里程(km) | 河道形态 | 是否有节点 | 河相系数 | 是否有阻隔性 | |
---|---|---|---|---|---|---|---|---|
沙市— 城陵矶 | 1 | 斗湖堤 | 9.9 | 175 | 单一微弯 | 无 | 2.55 | 是 |
2 | 石首 | 8 | 234 | 单一微弯 | 中间有 | 2.86 | 否 | |
3 | 碾子湾 | 15 | 242 | 单一微弯 | 无 | 4.76 | 否 | |
4 | 河口 | 7 | 257 | 单一微弯 | 无 | 3.19 | 否 | |
5 | 调关 | 13 | 264 | 单一微弯 | 无 | 2.61 | 是 | |
6 | 莱家铺 | 12 | 277 | 单一微弯 | 无 | 3.32 | 否 | |
7 | 塔市驿 | 14 | 289 | 单一微弯 | 无 | 2.98 | 是 | |
8 | 大马洲 | 10.5 | 330 | 单一微弯 | 进口有 | 6.68 | 否 | |
9 | 砖桥 | 9 | 338 | 单一微弯 | 无 | 3.66 | 是 | |
10 | 铁铺 | 12 | 347 | 单一顺直 | 无 | 4.31 | 否 | |
11 | 反咀 | 6.5 | 356 | 单一微弯 | 无 | 3.11 | 是 | |
12 | 七公岭 | 7.8 | 380 | 单一微弯 | 中下有 | 3.29 | 否 | |
城陵矶 —武汉 | 13 | 螺山 | 11 | 419 | 单一顺直 | 进口有 | 6.25 | 否 |
14 | 石头关 | 9 | 456 | 单一微弯 | 出口有 | 5.08 | 否 | |
15 | 龙口 | 9.6 | 483 | 单一微弯 | 出口有 | 3.42 | 是 | |
16 | 汉金关 | 10.9 | 519 | 单一微弯 | 无 | 3.25 | 是 | |
17 | 簰洲湾 | 15 | 542 | 单一微弯 | 无 | 2.14 | 否 | |
18 | 沌口 | 12 | 610 | 单一顺直 | 中间有 | 5.59 | 否 | |
19 | 武桥 | 13 | 628 | 单一顺直 | 进口有 | 4.47 | 否 | |
武汉— 湖口 | 20 | 阳逻 | 15 | 658 | 单一微弯 | 进口有 | 3.46 | 否 |
21 | 湖广 | 10 | 679 | 单一微弯 | 进口有 | 3.93 | 否 | |
22 | 巴河 | 9.4 | 723 | 单一顺直 | 进口有 | 4.52 | 否 | |
23 | 黄石 | 15.5 | 753 | 单一微弯 | 出口有 | 2.70 | 是 | |
24 | 牯牛沙 | 17 | 773 | 单一微弯 | 进口有 | 4.07 | 否 | |
25 | 搁排矶 | 15 | 802 | 单一微弯 | 两岸沿程有 | 0.79 | 是 | |
26 | 武穴 | 13 | 830 | 单一微弯 | 中上有 | 4.87 | 否 | |
27 | 九江 | 16 | 853 | 单一微弯 | 无 | 3.17 | 否 | |
湖口— 大通 | 28 | 上下三号—马垱过渡段 | 6 | 938 | 单一微弯 | 出口有 | 2.05 | 是 |
29 | 马垱—东流过渡段 | 8 | 972 | 单一微弯 | 无 | 2.96 | 是 | |
30 | 东流—官洲过渡段 | 9 | 995 | 单一微弯 | 中间有 | 3.47 | 否 | |
31 | 官洲—安庆过渡段 | 16 | 1023 | 单一微弯 | 中间有 | 2.71 | 否 | |
32 | 安庆—太子矶过渡段 | 8.4 | 1054 | 单一微弯 | 出口有 | 1.71 | 是 | |
33 | 太子矶—贵池过渡段 | 10.5 | 1078 | 单一微弯 | 无 | 4.16 | 否 | |
34 | 大通顺直段 | 16 | 1101 | 单一顺直 | 进口有 | 4.29 | 否 |
新窗口打开
3 阻隔性控制要素分析
3.1 平面控制要素
铁铺、螺山、沌口、武桥、巴河、大通等6个河段为单一顺直河道,由于河道较宽,难以约束上游河势调整导致的入流方向变化,伴随着交错边滩的复归性演变,本河段动力轴线大幅度上提下移[3];而调关、塔市驿、反咀等河段的河湾弯曲度较大,河湾出流方向因入流方向不同或流量不同而改变的幅度可以限制在较小范围内[8],从而使弯道具有良好的导流作用。表1中的34个单一河段,只有单一微弯河段才可能具有阻隔性,而单一顺直河段均不具有阻隔性,可见单一弯曲的平面形态是形成阻隔性河段、阻隔河势调整向下游传递的控制要素之一。长江中下游节点包括突出于岸线的山岩、胶泥咀、沉积年份较久的黏土层等。当单侧节点分布于河段中上部时,破坏了本河段平、断面形态的连续性,引起水流动力轴线弯曲半径大小的突变;上游河势调整后,入流动力轴线的角度、与节点贴靠程度发生变化[1],节点挑流强度随之变化,导致出流方向大幅度改变。东岳山、阳逻矶、白浒山、西塞山、狗头矶、吉阳矶、小闸口等节点分别位于石首、阳逻、湖广、牯牛沙、武穴、东流—官洲、官洲—安庆等河段的中上部,上游河势调整后,节点挑流强度变化,致使出流动力轴线方向的改变程度明显不同,进而导致本河段河势调整向下游传递。可见,阻隔性河段中上部不应具有起挑流作用的单侧节点。
长江中下游也存在大量对峙节点,作为抗冲性较强的河道边界,对峙节点有利于限制河宽、控制河道总体走向[4, 8]。但由于对峙节点挑流强度不同,一旦上游河势变化,节点交替挑流且有强弱之分,加之节点下游河岸地质组成也存在差异,将引起主流摆动及河势调整。例如杨林矶、龙头山对峙分布于螺山河段进口,由于两节点挑流强度不同[9],龙头山以下主流摆动频繁,加之下游界牌河段河岸抗冲性差、河道放宽,主流摆动幅度进一步加大[3],不具有阻隔性;再例如龟、蛇山节点对峙分布于武桥河段进口,也有类似特征。也有些对峙节点河段的河势相对稳定,如白螺矶、道人矶对峙分布于南阳洲河段,沙帽山、铁板矶对峙分布于铁板洲河段,其稳定成因在于上游的观音洲弯道、煤炭洲弯道的河势长期保持稳定,从而为下游提供了稳定的入流平面位置,使对峙节点挑流强度始终变化不大,因此其稳定性依赖于上游河势,一旦人为或自然因素改变上游河势,本河段河势也将随之调整。可见,具有对峙节点的河段,由于两节点挑流强度不同[9]以及节点两岸下游地质地貌条件的差异[10],难以在上游河势变化后,仍维持窄深型断面来约束主流摆动,因而不具有阻隔性。
3.2 横断面控制要素
河宽过大的河道难以有效约束水流动力轴线摆动,且往往有宽广河漫滩发育[11-12],当水流漫上河漫滩后,河宽骤然增加,河相系数显著增大[13],为动力轴线提供了充足的摆动空间;此时,若上游河势调整,势必引起本河段的动力轴线摆动,进而向下游传递。图1为各河段典型横断面图,为明显起见,将窄深型河段的深泓绘制于图中分界线左侧,将其他河段的深泓绘制于分界线右侧。可见,位于分界线左侧的斗湖堤、调关、塔市驿、砖桥、反咀、龙口、汉金关、黄石、搁排矶、上下三号—马垱、马垱—东流、安庆—太子矶等12个阻隔性河段为窄深型断面,不同流量级下的平均河相系数
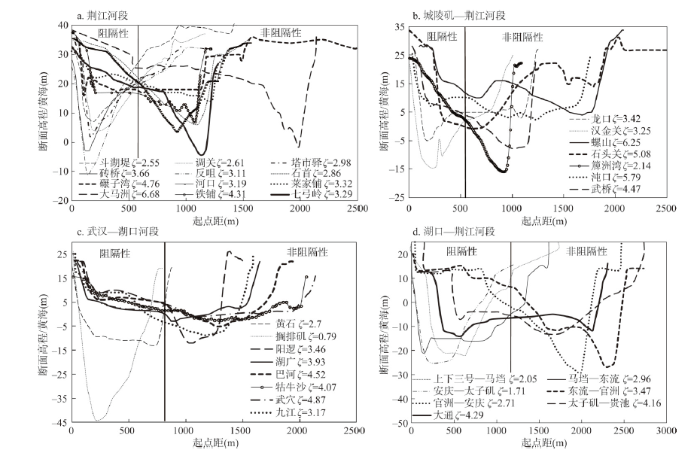
图1长江中下游单一河段的典型横断面
-->Fig. 1The typical transverse configurations of the typical cross-sections of the single-thread reaches in the middle and lower reaches of the Yangtze River
-->
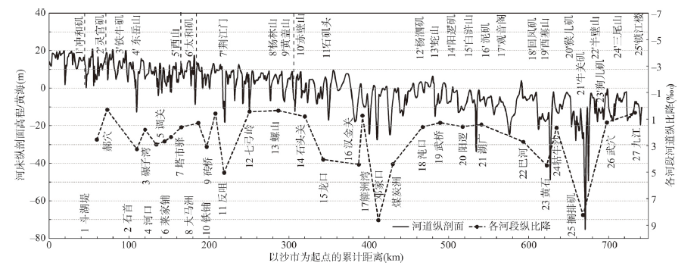
图2长江中游单一河段河床纵剖面及河道纵比降
-->Fig. 2The longitudinal gradients of the single-thread reaches and the longitudinal profile of the middle reaches of the Yangtze River
-->
3.3 纵剖面控制要素
对于进口深泓高程高于出口的顺坡河段,纵剖面越陡、纵比降越大[14],河段出口具有较强的吸流作用,有利于集中上游不同方向的来流而约束水流动力轴线摆动,主要有两种表现形式:一是河段出口由节点挑流形成的冲刷坑,如龙口、黄石、搁排矶河段;二是弯顶凹岸下游由弯道环流形成的冲刷坑,如调关、反咀、汉金关河段。对于逆坡或出口有支流汇入壅水顶托的河段,往往不具有阻隔性。统计表明,除顺直、有节点、3.4 河岸及河床控制要素
Julian等[15]研究表明,河岸抗冲性正比于其黏粒含量。图3为长江中游单一河段的凹岸黏粒含量及床沙中值粒径,上述剩余的14个河段中,仅有河口、莱家铺河段的凹岸黏粒含量低于9.5%而不具有阻隔性,其余12个河段均高于9.5%;仅有河口河段的床沙中值粒径小于0.158 mm而不具有阻隔性,其余12个河段均大于0.158 mm。可见,只有河岸抗冲性较强,才能防止崩岸的发生及河道的展宽[16-17],本河段方可保持自身河势稳定,从而维持微弯窄深的断面形态,约束水流动力轴线摆动;只有床沙较粗、抗冲性较强、动床阻力较大,本河段的河床形态才难以改变[18],水流动力轴线难以大幅度摆动。因此,凹岸黏粒含量高于9.5%、床沙中径大于0.158 mm,也是形成阻隔性河段的控制要素之一。值得指出的是,阻隔性河段的护岸方量平均值为189.1 m3/m,非阻隔性河段仅有126.9 m3/m,阻隔性河段中河岸天然地质条件相对薄弱的调关、塔市驿、砖桥、反咀等河段,护岸方量均较大,从而保证了阻隔性河段的河岸具有较强抗冲性。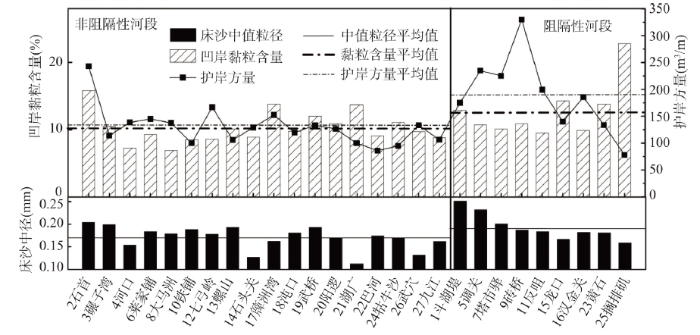
图3长江中游单一河段凹岸黏粒含量、床沙中值粒径
-->Fig. 3The riverbank clay content and the median diameter of the bed sediment of the single-thread reaches in the middle reaches of the Yangtze River
-->
4 阻隔性河段作用机理分析
研究表明[7],河势调整向下游传递的实质是主流平面位置的变化向下游传递。上述分析表明,只有单一微弯河段才可能具有阻隔性,而水流动力轴线弯曲半径能较好反应天然河湾的主流平面位置,主流摆动可通过弯曲半径变化反映出来,因此,本节从推导水流动力轴线弯曲半径入手,分析上述各控制要素对阻隔性的作用,剖析阻隔性河段作用机理。4.1 河湾水流动力轴线弯曲半径公式推导
(1)公式推导三维水流运动方程Navier-Stokes方程可表示为:
忽略紊动扩散项及非恒定项,底部床面阻力项采用
式中:φ、R分别为河湾的弯曲度(以弧度表示)和垂线所在位置的弯曲半径;u、v分别为(φ、R)处的垂线平均流速;Jφ为河道纵向比降;h为垂线水深;p为动水压强;g为重力加速度;ρ为水体的密度。将曼宁公式和谢才系数C带入底部床面阻力项之中得到:
考虑到横向流速远小于纵向流速,忽略带有v的项,同时动水压强是由岸壁切应力产生,因此p可表示为岸壁切应力τ(单位为
Yin等[19]研究得出河床糙率与粗化层的下限粒径d关系为:
对式(5)按一阶常微分方程求解得到
式中:考虑在同一断面中,水流动力轴线所在处的流速、河道纵比降、水深最大,
根据式(7)可求解出水流动力轴线弯曲半径R0表达式:
长江中下游为大型冲积河道,沿程有洞庭四水、鄱阳五河、汉江等支流入汇,年内年际间流量变幅较大[23],因此在式(8)的基础上增设
式中:
(2)公式合理性分析
已有众多****[8, 24-25]对河湾水流动力轴线弯曲半径公式进行了大量研究,并提出了诸多半经验半理论或者经验性公式,这些公式均认为水流动力轴线弯曲半径与流量大小呈正相关,这与河湾“大水趋直、小水坐弯”的一般规律性认识是一致的。从式(9)可以看出,本次推导的水流动力轴线弯曲半径公式也与流量大小呈正相关,与已有研究成果及认识一致。
同时,已有研究[1-2, 4, 7, 9-10]也逐渐认识到进口节点挑流在河床演变中的重要作用,基于此,式(9)考虑了节点挑流作用,这是与已有研究成果主要的不同之处。为验证式(9)的合理性,采用式(9)及已有公式,计算牯牛沙河段不同流量不同断面的水动力轴线弯曲半径,并与实测值进行比较(图4,表2)。可以看出,式(9)与实测值更符合,表明式(9)更适用于牯牛沙这类进口存在挑流节点的河段的水流动力轴线弯曲半径计算。
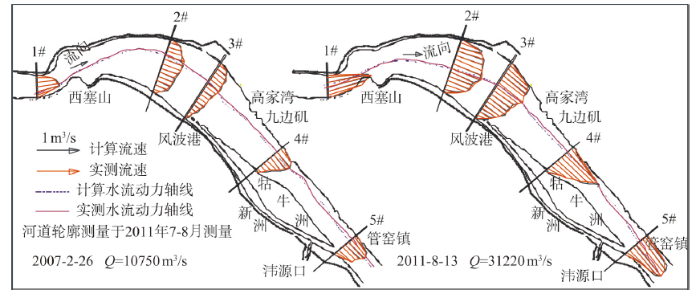
图4式(9)计算的牯牛沙河段水流动力轴线验证
-->Fig. 4The verification of the flow dynamic axis calculated by Eq.9 in the Guniusha Reach
-->
Tab. 2
表2
表2牯牛沙河段公式计算结果与实测值对比表
Tab. 2The comparison of the formula results with the measured values in the Guniusha Reach
弯曲半径计算公式(m) | 流量(m3/s) | ||||||
---|---|---|---|---|---|---|---|
1# Q=10750 | 1# Q=31220 | 2# Q=10750 | 2# Q=31220 | 2'# Q=10750 | 2'# Q=31220 | ||
实测值 | 2530 | 2900 | 2820 | 3940 | 3090 | 3750 | |
欧阳履泰[7] | 2390 | 3771 | 2390 | 3771 | 2390 | 3771 | |
张敬笃[23] | 851 | 890 | 1549 | 1919 | 1814 | 2246 | |
张植堂[24] | 2204 | 3597 | 2056 | 3531 | 2163 | 3446 | |
本研究 | 式(9) | 2498 | 2976 | 2782 | 3997 | 3133 | 3771 |
新窗口打开
4.2 阻隔性河段作用机理分析
(1)各控制要素对阻隔性河段作用机理式(9)可以写成:
式中:
若流量过程恒定,水流动力轴线平面位置将不会发生时空变化,也就不会出现河势调整,流量过程变化是促进动力轴线摆动的动力因子。3.1节分析表明,河段中上部存在挑流节点将加剧不同流量下动力轴线的摆动幅度;河湾弯曲度过小的顺直河段,河湾曲率半径与动力轴线弯曲半径差别较大而削弱了河湾对动力轴线的归顺作用[8];河相系数过大的宽浅断面,为动力轴线提供了较大摆动空间,滩槽高差较小也利于主流切滩。因此,流量过程、节点挑流、河湾弯曲度、河相系数均是促进动力轴线摆动的因素,式(10)中右边分子项能够表征水流动力轴线摆动力的作用。
3.1节分析表明,河道纵比降大,深槽处水流功率大,有利于刷深深槽[26];河岸物质组成中黏粒含量较多时,抗冲性较强而有利于塑造窄深断面,减小动力轴线的横向摆动空间;床沙较粗时,河床对水流侵蚀的抵抗力较强,也一定程度上减小动力轴线的摆幅。因此,河道纵比降、河岸及河床抗冲性等均是约束动力轴线摆动的因素,在式(10)右边分母项能够表征河道边界约束力的作用。
综上分析,式(10)右边项近似反映了水流动力轴线摆动力与河道边界条件约束力之间的对比关系,宏观上则表现为水流动力轴线弯曲半径与河湾曲率半径的对比,可见上述公式结构是基本合理的,第三节分析的各控制要素对水流动力轴线摆动的促进或者约束作用也是合理的。
为进一步分析不同控制要素对水流动力轴线摆动的影响程度,整理各控制要素变化范围如下:流量在4000~80000 m3/s之间,河湾曲率半径约在2000~16000 m之间,河相系数在0.8~6.7之间,节点突出岸线的相对长度在0.67~1.0之间,河段纵比降在0.40/000~8.20/000之间,床沙中值粒径在0.11~0.25 mm之间,凹岸岸坡黏粒含量在6.9%~22.8%之间。令任一控制要素在上述范围内相对变化,其他控制要素保持该范围的平均值不变,其余参数取各河段平均值,分析
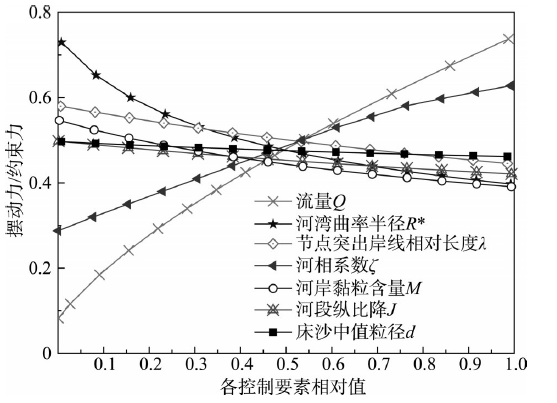
图5各控制要素对河段阻隔性影响的敏感度分析
-->Fig. 5Sensitivity analysis of the influences of various control factors on the barrier river reaches
-->
(2)主流摆动力与边界约束力的比值决定河段阻隔性
根据式(10),计算表1中34个河段
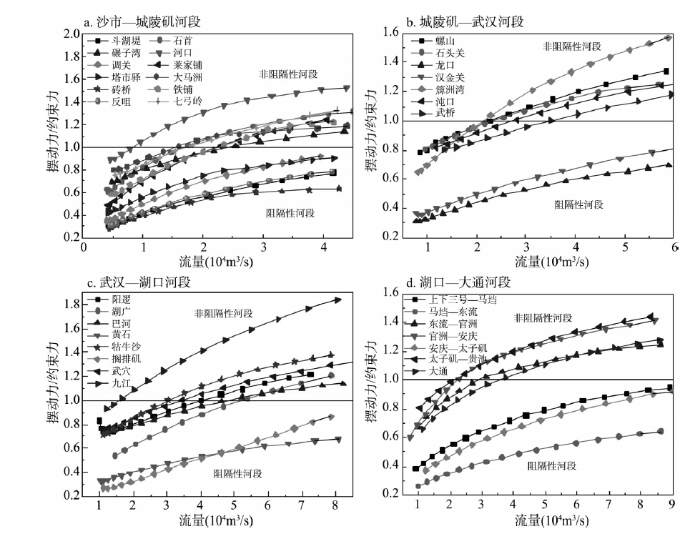
图6长江中下游单一河段水流动力轴线摆动力与河道边界约束力的比值
-->Fig. 6The ratio of the migration force of the flow dynamic axis to the confining force of the channel boundaryin the middle and lower reaches of the Yangtze River
-->
综上,阻隔性河段作用机理在于:在不同流量级下,河道约束力始终大于主流摆动力,即便上游河势发生明显调整,入流方向发生大幅度变化,但本河段的河道边界始终能够约束动力轴线的平面位置,从而削弱上游河势变化后主流摆动幅度,归顺上游不同河势条件下的主流平面位置,为下游河道提供了相对稳定的入流条件。第3节分析的阻隔性河段在平面、横断面、纵剖面、河岸及河床抗冲性方面的要素,正是河道约束力大于主流摆动力的必要条件。不满足上述一个或多个条件的河道,随着入流方向或流量变化,河道约束力未能始终大于主流摆动力,主流平面位置将发生较大变化,河势随之调整,也就不具有阻隔性。
5 结论
以长江中下游34个单一河段为研究对象,在判别各河段是否具有阻隔性的基础上,对比分析了阻隔性控制要素,推导了水流动力轴线弯曲半径计算式,分析了各控制要素对阻隔性河段的作用机理,主要结论如下:(1)阻隔性河段形成的控制要素包括:河道具有单一微弯的平面形态、中上部无挑流节点;河相系数小于4;河道纵比降大于1.2?;凹岸黏粒含量高于9.5%、床沙中值粒径大于0.158 mm等。
(2)阻隔性河段的作用机理在于,不同流量级下,河道边界约束力始终大于水流动力轴线摆动力,即便上游河势发生调整,本河段的河道边界始终能约束主流的摆动幅度,从而归顺上游不同流量级及不同河势条件下的主流平面位置,为下游河道提供了相对稳定的入流条件。
The authors have declared that no competing interests exist.
参考文献 原文顺序
文献年度倒序
文中引用次数倒序
被引期刊影响因子
[1] | . |
[2] | |
[3] | . |
[4] | . |
[5] | 基于历年实测资料,详细分析了马鞍山河段演变规律及其对上游陈家洲汊道段演变的响应机理,进而揭示了马鞍山河段演变趋势。结果表明,马鞍山河段多年来保持分汊河势格局,右汊河势相对稳定,但左汊河势变化剧烈,左汊深泓平面位置与上游陈家洲汊道河势演变密切相关,当陈家洲左汊发展、西梁山挑流作用较强时,江心洲左汊深泓呈二次过渡,当陈家洲右汊发展、西梁山挑流作用较弱时,江心洲左汊深泓呈一次过渡。近期,陈家洲左汊淤积、右汊冲刷,陈家洲头低滩切割趋势明显,这些变化将会导致西梁山挑流作用逐渐减弱,马鞍山河段左汊主流可能逐渐调整成一次过渡形式,左汊内河势将发生较大调整。 . 基于历年实测资料,详细分析了马鞍山河段演变规律及其对上游陈家洲汊道段演变的响应机理,进而揭示了马鞍山河段演变趋势。结果表明,马鞍山河段多年来保持分汊河势格局,右汊河势相对稳定,但左汊河势变化剧烈,左汊深泓平面位置与上游陈家洲汊道河势演变密切相关,当陈家洲左汊发展、西梁山挑流作用较强时,江心洲左汊深泓呈二次过渡,当陈家洲右汊发展、西梁山挑流作用较弱时,江心洲左汊深泓呈一次过渡。近期,陈家洲左汊淤积、右汊冲刷,陈家洲头低滩切割趋势明显,这些变化将会导致西梁山挑流作用逐渐减弱,马鞍山河段左汊主流可能逐渐调整成一次过渡形式,左汊内河势将发生较大调整。 |
[6] | 借助于天兴洲河段较为系统的年内与年际水沙输移、河床变形等方面原型观测资料,分析了顺直和分汊两种河型交界位置的洲滩和浅滩动态特征.近20年来洲滩形 态比较表明,上游顺直河道内汉口边滩上低矮成型淤积体具有以5-6年为周期缓慢下移和突然上提的特性,从而导致汊道进口低滩冲淤具有周期性;近5年来断面 流速、含沙量和床沙组成资料的统计表明,汊道进口主流位置在汛枯期左右摆动、漫滩横流强度不断转换,洲头低滩的冲淤强度与年际之间来流条件具有相关性.以 上两种因素迭加作用之下,汊道进口的低滩形态不仅随年际之间来水来沙变化而波动,还会因上游顺直河段内成型淤积体上提下移的影响而周期性地淤长萎缩.这种 复杂的动态调整特点,使航道条件以若干年为周期,交替呈现出优劣转换.在此结论基础上,可以推测三峡水库蓄水后水沙条件下,该类型汊道进口的航道条件可能 趋于恶化. . 借助于天兴洲河段较为系统的年内与年际水沙输移、河床变形等方面原型观测资料,分析了顺直和分汊两种河型交界位置的洲滩和浅滩动态特征.近20年来洲滩形 态比较表明,上游顺直河道内汉口边滩上低矮成型淤积体具有以5-6年为周期缓慢下移和突然上提的特性,从而导致汊道进口低滩冲淤具有周期性;近5年来断面 流速、含沙量和床沙组成资料的统计表明,汊道进口主流位置在汛枯期左右摆动、漫滩横流强度不断转换,洲头低滩的冲淤强度与年际之间来流条件具有相关性.以 上两种因素迭加作用之下,汊道进口的低滩形态不仅随年际之间来水来沙变化而波动,还会因上游顺直河段内成型淤积体上提下移的影响而周期性地淤长萎缩.这种 复杂的动态调整特点,使航道条件以若干年为周期,交替呈现出优劣转换.在此结论基础上,可以推测三峡水库蓄水后水沙条件下,该类型汊道进口的航道条件可能 趋于恶化. |
[7] | 长江中下游长河段长时段的演变资料分析表明河势调整往往会向下游传递,但是部分河段却能阻隔这种河势调整的传递效应,称之为阻隔性河段.统计表明,阻隔性河段应同时具有单一微弯的平面形态、凹岸抗冲性较强且中上段无挑流节点、河宽随水位变化率不超过45等三个特征.进一步分析表明,这种河段具有阻隔性的根本原因是其能够归顺不同流量级的主流平面位置,使得无论上游河势如何调整,其出流的主流平面位置基本稳定,为下游河段提供相对稳定的入流条件.河道整治过程中,宜抓住有利时机塑造阻隔性河段效果,无阻隔性影响的长河段宜自上而下系统规划整治,对大幅度崩岸、滩体大幅萎缩等可能破坏阻隔性的变化应及时整治,防止阻隔性丧失. . 长江中下游长河段长时段的演变资料分析表明河势调整往往会向下游传递,但是部分河段却能阻隔这种河势调整的传递效应,称之为阻隔性河段.统计表明,阻隔性河段应同时具有单一微弯的平面形态、凹岸抗冲性较强且中上段无挑流节点、河宽随水位变化率不超过45等三个特征.进一步分析表明,这种河段具有阻隔性的根本原因是其能够归顺不同流量级的主流平面位置,使得无论上游河势如何调整,其出流的主流平面位置基本稳定,为下游河段提供相对稳定的入流条件.河道整治过程中,宜抓住有利时机塑造阻隔性河段效果,无阻隔性影响的长河段宜自上而下系统规划整治,对大幅度崩岸、滩体大幅萎缩等可能破坏阻隔性的变化应及时整治,防止阻隔性丧失. |
[8] | |
[9] | . 长江中游城螺河段河道顺直分汊,其演变受江湖关系影响较大。河床演变分析表明:自五十年代以来,本河段河势稳定,河道横断面形态杨林山以上为左侧淤积、右侧无明显变化。多年来南阳洲未见有累积性的淤积或冲刷。仙峰洲则因河道左淤右冲而并于左岸成为边滩。江湖关系调整及来水来沙条件的改变使本河段枯水河槽出现了一定的淤积。受城陵矶、白螺矶 ̄道人矶、杨林山 ̄龙头山等节点的控制,南阳洲以上段深泓摆动很小,主流一直靠右岸沿 . 长江中游城螺河段河道顺直分汊,其演变受江湖关系影响较大。河床演变分析表明:自五十年代以来,本河段河势稳定,河道横断面形态杨林山以上为左侧淤积、右侧无明显变化。多年来南阳洲未见有累积性的淤积或冲刷。仙峰洲则因河道左淤右冲而并于左岸成为边滩。江湖关系调整及来水来沙条件的改变使本河段枯水河槽出现了一定的淤积。受城陵矶、白螺矶 ̄道人矶、杨林山 ̄龙头山等节点的控制,南阳洲以上段深泓摆动很小,主流一直靠右岸沿 |
[10] | . |
[11] | |
[12] | During 2008 and 2009 heavy rainfall took place around the Mazatlan County in the Sinaloa state, Mexico, with a return period (Tr) between 50 and 100 years. As a result, the region and its infrastructure, such as the railways and highways (designed for a Tr = 20 years) were severely exposed to floods and, as a consequence damage caused by debris and sediments dragged into the channel. One of the highest levels of damage to the infrastructure was observed in the columns of Quelite River railway bridge. This is catastrophic as the railway is very important for trade within the state and also among other states in Mexico and in the USA. In order to understand the impact of the flooding and to avoid the rail system being damaged it is necessary to analyse how significant the changes in the river channel have been. This analysis looks at the definition of the main channel and its floodplain as a result of the sediment variability, not only at the bridge area, but also upstream and downstream. The Quelite River study considers the integration of Geographic Information Systems (GIS) and remote sensing data to map, recognise and assess the spatio-temporal change channel morphology. This increases the effectiveness of using different types of geospatial data with in situ measurements such as hydrological data. Thus, this paper is an assessment of a 20 years study period carried out using historical Landsat images and aerial photographs as well as recent Spot images. A Digital Elevation Model (DEM) of local topography and flow volumes were also used. The results show the Quelite River is an active river with a high suspended sediment load and migration of meanders associated to heavy rainfall. The river also has several deep alluvial floodplain channels which modified the geometry and other morphological characteristics of the channel in the downstream direction. After the identification of the channel changes, their causes and solutions to control, the channel migration and the dynamics structure, a river management plan was projected not only to protect the bridge but also to provide a flood risk awareness in order to reduce the social揺conomical impact during a flood event. |
[13] | In contrast with the results of other studies, the most recent evolutionary trend of the Taro River shows substantial morphological stability with possible slight narrowing. The identification of the variations along the channel, facilitated by the analysis of the parameter curves and supported by the historical documentation, reveals that these variations can be substantially attributed to human activities. In particular, the continuous narrowing is largely due to the recurrent subtraction of riverbed areas to be used for agricultural and industrial purposes, as well as to the construction of 10 bridges with the relevant bank protections. The intense mining between 1950s and 1980s seems to have caused a sharp incision and partial narrowing only. The morphological changes due to the reduction in the flow regime, which seem to emerge from the sporadic and discontinuous hydrological data, are expected to be negligible and, in any case, are immaterial when compared with the remarkable changes due to human activities. |
[14] | 61Channel profiles and erosion rates constrain processes of knickzone formation.61Knickzones in Abukuma massif are localized along an uplift rate boundary.61Models of channel profile evolution place constraints on subsurface fault geometry. |
[15] | This study identifies and assesses the controls on hydraulic erosion of cohesive riverbanks on a 600-m reach of an urban ephemeral stream with active bank erosion. We examined hydraulic bank erosion by separating estimated bank shear stress into four properties: magnitude, duration, event peak, and variability. The values of these independent variables were used as a bank erosion context at three transects. Stepwise regression showed that the event peak (maximum peak) of excess shear stress best predicts cohesive bank erosion at the two transects with moderate critical shear stresses (1.93–4.08 N/m 2 ), while the variability (all peaks) of excess shear stress best predicts erosion at the transect with low critical shear stress (0.95 N/m 2 ). These results suggest that the amount of hydraulic erosion of cohesive riverbanks is dictated by flow peak intensities. Finally, the results of this study were combined with results from previous bank erosion studies to produce a conceptual model for estimating bank erosion rates based on their silt–clay content. |
[16] | By integrating the individual values of those six parameters out of those eight parameters (the first two parameters remained constant for the particular study area), a bank erosion vulnerability zonation map of the River Haora, Tripura, India (23°37′–23°53′ N. and 91°15′–91°37′ E.) has been prepared. The values have been compared with the existing BEHI-NBS method of 60 spots and also with field data of 30 cross sections (covering the 60 spots) taken along a 51-km stretch of the river within Indian Territory, and we found that the estimated values are matching with the existing method as well as with field data. The whole stretch has been divided into five hazard zones, i.e. very high, high, moderate, low and very low hazard zones; and they are cover 5.66, 16.81, 40.82, 29.67, and 9.0402km, respectively. |
[17] | The flow and sediment transport processes near steep streambanks, which are commonly found in meandering, braided, and anastomosing stream systems, exhibit complex patterns that produce intricate interactions between bed and bank morphologic adjustment. Increasingly, multi-dimensional computer models of riverine morphodynamics are used to aid in the study of these processes. A number of depth-averaged two-dimensional models are available to simulate morphologic adjustment of both bed and banks. Unfortunately, these models use overly simplified conceptual models of riverbank erosion, are limited by inflexible structured mesh systems, or are unable to accurately account for the flow and sediment transport adjacent to streambanks of arbitrary geometry. A new, nonlinear model is introduced that resolves these limitations. The model combines the river morphodynamics computer models TELEMAC-2D and SISYPHE of the open source TELEMAC-MASCARET suite of solvers with the bank erosion modules of the CONCEPTS channel evolution computer model. The performance of the new model is evaluated for meander-planform initiation and development. The most important findings are: (1) the model is able to simulate a much greater variety and complexity in meander wavelengths; (2) simulated meander development agrees closely with the unified bar-bend theory of Tubino and Seminara (1990); and (3) the rate of meander planform adjustment is greatly reduced if the wavelength of alternate bars is similar to that of meanders. |
[18] | Particle dynamics refers to production, erosion, transport, and storage of particulate material including mineral sediment and organic matter. Particle dynamics differ significantly between the end members of bedrock and alluvial river segments and between alluvial river segments with different grain-size distributions. Bedrock segments are supply limited and resistant to change, with relatively slow, linear adjustments and predominantly erosion and transport. Particle dynamics in alluvial segments, in contrast, are transport limited and dominated by storage of mineral sediment and production of organic matter. Alluvial segments are resilient to change, with relatively rapid, multidirectional adjustments and stronger internal influences because of feedbacks between particles and biota. Bedrock segments are the governors of erosion within a river network, whereas alluvial segments are the biogeochemical reactors. Fundamental research questions for both types of river segments center on particle dynamics, which limit network-scale incision in response to base level fall (bedrock segments) and habitat, biogeochemical reactions, and biomass production (alluvial segments). These characterizations illuminate how the spatial arrangement of bedrock and alluvial segments within a river network influence network-scale resistance and resilience to external changes in relative base level, climate, and human activities. |
[19] | |
[20] | The concept of sedimentation zone is applied to the study of spatial and temporal variations of mid-channel bars in the middle Hanjiang River, a large sand-bed braided river in China. The river channel can be divided into alternating sedimentation zones and transport zones, the formation of which is basically controlled by local channel boundary conditions such as natural and artificial bank controls (bedrock outcrops, local hill spurs, terraces, artificial dikes and influence of tributaries). A systematic comparison between sedimentation and transport zones is made in this study, including the flow shear stress, stream power, mid-channel bar features and channel stability. The results obtained indicate that different channel boundary conditions in sedimentation and transport zones control channel width and width-depth ratio, which in turn control the reach's hydraulic and sediment transport characteristics. The storage and removal of sediment in sedimentation zones correspond to the increase and decrease of the degree to which mid-channel bars develop. When the sediment input of the channel system is altered by reservoir construction, the bed-load sediment historically accumulated is released by clear water scour, thus a macroscopic bed-load `wave' forms and moves gradually downstream. Associated with this is the complex response phenomenon of mid-channel bar evolution. |
[21] | |
[22] | . |
[23] | Significant channel adjustments have occurred in the Jingjiang Reach of the Middle Yangtze River, because of the operation of the Three Gorges Project (TGP). The Jingjiang Reach is selected as the study area, covering the Upper Jingjiang Reach (UJR) and Lower Jingjiang Reach (LJR). The reach-scale bankfull channel dimensions in the study reach were calculated annually from 2002 to 2013 by means of a reach-averaged approach and surveyed post-flood profiles at 171 sections. We find from the calculated results that: the reach-scale bankfull widths changed slightly in the UJR and LJR, with the corresponding depths increasing by 1.6塵 and 1.0塵; the channel adjustments occurred mainly with respect to bankfull depth because of the construction of large-scale bank revetment works, although there were significant bank erosion processes in local regions without the bank protection engineering. The reach-scale bankfull dimensions in the UJR and LJR generally responded to the previous five-year average fluvial erosion intensity during flood seasons, with higher correlations being obtained for the depth and cross-sectional area. It is concluded that these dynamic adjustments of the channel geometry are a direct result of recent human activities such as the TGP operation. |
[24] | . |
[25] | |
[26] | Understanding the controls on the morphological variability of river systems constitutes one of the fundamental questions in geomorphic investigation. Channel morphology is an important indicator of river processes and is of significance for mapping the hydrology-ecologic connectivity in a river system and for predicting the future trajectory of river health in response to external forcings. This paper documents the spatial morphological variability and its natural and anthropogenic controls for the Yamuna River, a major tributary of the Ganga River, India. The Yamuna River runs through a major urban centre i.e. Delhi National Capital Region. The Yamuna River was divided into eight geomorphically distinct reaches on the basis of the assemblages of geomorphic units and the association of landscape, valley and floodplain settings. The morphological variability was analysed through stream power distribution and sediment load data at various stations. Stream power distribution of the Yamuna River basin is characterised by a non-linear pattern that was used to distinguish (a) high energy ‘natural’ upstream reaches, (b) ‘anthropogenically altered’, low energy middle stream reaches, and (c) ‘rejuvenated’ downstream reaches again with higher stream power. The relationship between stream power and channel morphology in these reaches was integrated with sediment load data to define the maximum flow efficiency (MFE) as the threshold for geomorphic transition. This analysis supports the continuity of river processes and the significance of a holistic, basin-scale approach rather than isolated local scale analysis in river studies. |