

2
3
A review of the calibration methods for measuring the carbon and oxygen isotopes in CO2 based on isotope ratio infrared spectroscopy
PANG Jia-Ping1,2, WEN Xue-Fa

2
3
通讯作者:
基金资助: |
Online:2018-04-19
Supported by: | SupportedbytheNationalKeyResearchandDevelopmentProjectofChina(2016YFC0500102)( |

摘要
关键词:
Abstract
Keywords:
PDF (937KB)摘要页面多维度评价相关文章导出EndNote|Ris|Bibtex收藏本文
引用本文
庞家平, 温学发. 稳定同位素红外光谱技术测定CO2同位素校正方法的研究进展. 植物生态学报, 2018, 42(2): 143-152 doi:10.17521/cjpe.2017.0206
PANG Jia-Ping, WEN Xue-Fa.
稳定同位素观测仪器和方法的进步使稳定同位素技术成为解决生态、大气和水文等研究热点与难点问题的重要工具(Yakir & Sternberg, 2000; Bowling et al., 2003; Griffis, 2013; 林光辉, 2013; Chen et al., 2017)。稳定同位素红外光谱(IRIS)技术的发展使得大气CO2的碳同位素组成(δ13C)和氧同位素组成(δ18O)的高时间分辨率和高精度的原位连续观测成为可能(McManus et al., 2010; Tanaka et al., 2013; Wen et al., 2013)。商业化的仪器包括调制式半导体激光吸收光谱(TDLAS)、波长扫描光腔衰荡光谱(WS-CRDS)、离轴综合腔输出光谱(OA-ICOS)、量子级联激光吸收光谱(QCLAS)和差频激光光谱(DFG)等(Griffis, 2013; Wen et al., 2013)。
IRIS技术突破了传统大气CO2气瓶采样-稳定同位素质谱(IRMS)技术的分辨率低且费时耗力的局限性, 能够实现CO2碳氧同位素比值的原位连续观测(Bowling et al., 2003, 2005; Schaeffer et al., 2008; Bickford et al., 2010; Wingate et al., 2010; Shim et al., 2011)。所有IRIS仪器的观测结果都需要能够高精度地测定自然条件下大气CO2 δ13C和δ18O的变异特征, 并准确地追溯到国际标准(Bowling et al., 2005; Schaeffer et al., 2008; Griffis, 2013)。基于IRIS技术测量CO2 δ13C和δ18O的主要误差来源包括δ13C和δ18O测量值对CO2浓度变化的非线性响应(浓度依赖性)以及对环境条件变化的敏感性导致的漂移(时间漂移)(Griffith et al., 2012; Guillon et al., 2012; Vogel et al., 2013)。例如, Guillon等(2015)研究表明, 在300-17 800 μmol·mol-1 CO2浓度范围内, 利用OAICOS技术的Los Gatos Research CCIA-EP测定的CO2 δ13C浓度依赖性达到19‰, 而在300-1 000 μmol·mol-1的浓度范围内达到6‰。Pang等(2016a)研究表明, 在368.1-550.1 μmol·mol-1浓度范围内, 利用WS-CRDS技术的Picarro G1101-i和G2201-i测定的CO2 δ13C浓度依赖性分别为1.75‰和0.47‰。仪器的长期漂移也是影响IRIS仪器测量结果准确性的重要因素(Vogel et al., 2013; Guillon et al., 2015)。例如, Vogel等(2013)研究表明, 利用WS-CRDS技术的Picarro G1101-i测定的CO2 δ13C在24 h的漂移为0.3‰。Guillon等(2015)利用OA-ICOS 技术的Los Gatos Research CCIA-EP测定的CO2 δ13C在24 h内变化为5‰。Pang等(2016a)在48 h的测量周期内, 利用WS-CRDS技术的Picarro G1101-i和G2201-i测定的CO2 δ13C时间漂移分别为0.92‰-1.09‰和0.19‰-0.37‰。
采用适当的浓度依赖性和时间漂移的校正方法是提升仪器的测量精度, 并获得可追溯到国际标准的准确测量结果的重要保证。为了确保所有IRIS仪器都能高精度地测定自然条件下δ值的变异特征, 并准确地溯源到国际标准, 需要对IRIS仪器的测量结果进行适当的校正, 以消除浓度依赖性和时间漂移带来的误差(Bowling et al., 2005; Schaeffer et al., 2008; Griffis, 2013)。目前已有的研究通常根据仪器性能和研究需要采用不同的理论或经验的校正方法进行浓度依赖性和时间漂移校正(Bowling et al., 2003; Tuzson et al., 2008; Vogel et al., 2013; Guillon et al., 2012, 2015)。尽管IRIS仪器在我国生态、大气和水文等研究中得到广泛应用, 但还缺乏IRIS技术的浓度依赖性和时间漂移等产生的原因及其校正方法的系统论述。
本文概述了稳定同位素比值和同位素通量比值的概念, 系统阐述了浓度依赖性的概念、校正原理、理论校正及经验校正的方法和应用, 阐述了时间漂移的概念、校正方法和应用, 综述了数据溯源至国际标准的原理、方法和应用, 推荐了浓度依赖性和时间漂移等的校正方法, 并指出了IRIS技术和方法应用研究的瓶颈问题和在生态学中的应用潜力。
1 同位素比值与同位素通量比值
稳定同位素是指不发生或极不易发生放射性衰变的具有相同质子数、不同中子数的同一元素的不同核素。稳定同位素组成可以用同位素丰度或原子百分比、绝对丰度、原子百分超或同位素比率表示(林光辉, 2013)。由于重同位素的自然丰度较低, CO2的碳氧同位素组成通常用同位素比率(R)来表示:\[{}^{13}R\text{(C}{{\text{O}}_{2}}\text{) }=\frac{{}^{13}\text{C}}{{}^{12}\text{C}}=\frac{{}^{13}\text{C}{{\text{O}}_{2}}}{{}^{12}\text{C}{{\text{O}}_{\text{2}}}} \ \ (1) \]
\[{}^{18}R(C{{O}_{2}}) =\frac{^{18}O}{^{16}O}=\frac{{{C}^{16}}{{O}^{18}}O}{2\times C{{O}_{2}}} \ \ (2) \]
为便于比较, CO2碳氧同位素组成通常用同位素比值(δ值)来表示, 其定义为:
\[{{\delta }_{sample}}=\left( \frac{{{R}_{sample}}}{{{R}_{VPDB}}}-1 \right)\times 1000 \ \ (3) \]
式中Rsample是所测定样品的同位素比率, RVPDB是CO2中碳或氧稳定同位素国际标准的同位素比率, 分别为0.011 179 7和0.002 088 349 077。δsample表示样品的同位素比率相对于标准物质同位素比率的相对千分差, 可以清晰地反映同位素组成的变化。δ值通常用千分数(‰)表示。
CO2通量的碳氧同位素组成通常用同位素通量比率(RF)来表示:
\[{}^{13}R{{(C{{O}_{2}})}_{F}}=\frac{F{}^{13}C}{F{}^{12}C}=\frac{F{}^{13}C{{O}_{2}}}{F{}^{12}C{{O}_{2}}} \ \ (4) \]
\[{}^{18}R{{(C{{O}_{2}})}_{F}}=\frac{F{}^{18}O}{F{}^{16}O}=\frac{FC{}^{16}O{}^{18}O}{2\times FC{}^{16}{{O}_{2}}} \ \ (5) \]
其中, F12C和F13C(F16O和F18O)分别为轻重同位素的通量。同位素通量比率可以由涡度协方差或者通量廓线技术的进行观测和计算(Santos et al., 2012; Sturm et al., 2012; Wehr et al., 2016)。同样, 为便于比较, CO2通量碳氧同位素组成也通常用同位素通量比值(δ值)来表示, 其定义为:
\[{{\delta }_{F}}=\left( \frac{{{R}_{F}}}{{{R}_{VPDB}}}-1 \right)\times 1000 \ \ (6) \]
式中RF是所测定通量的同位素通量比率, δ值通常用千分数(‰)表示。
CO2浓度是标量, 而CO2通量是矢量, 同样同位素比值(δ值)是标量而同位素通量比值(δ值)是矢量。
通常, IRIS仪器校正分为内部校正和外部校正。内部校正是指试验前由仪器制造商或者仪器使用者通过直接改变分析仪本身参数设置而达到校正目的(Guillon et al., 2012; Sturm et al., 2012)。外部校正则是指试验过程中仪器使用者通过设置不同的标准气体来校正仪器浓度依赖性和时间漂移(Wen et al., 2013)。在本文中, 所指的CO2碳氧同位素校正方法都是针对同位素比值(δ值)的外部校正方法。
2 浓度依赖性的校正原理、方法和应用
2.1 浓度依赖性产生的理论基础
浓度依赖性是指IRIS仪器测量具有相同δ13C (或δ18O)的不同浓度的CO2标准气体时表现出的δ测量值与CO2浓度的非线性关系(Wen et al., 2013)。在理想条件下, IRIS仪器应该没有浓度依赖性现象, 即具有相同δ13C (或δ18O)值的不同浓度CO2的δ测量值应该恒定不变。由于IRIS仪器直接测量每个核素的浓度, 根据测量温度和压力等将浓度转换为摩尔混合比率后计算δ值(Wehr et al., 2013), 因此, 单个核素浓度的测量值与真值之间的测量误差导致了IRIS仪器的浓度依赖性(Griffith et al., 2012)。IRIS仪器δ测量值对浓度变化的浓度依赖性是测量误差的主要来源。以CO2 δ13C为例, 当仪器对12CO2和13CO2浓度的响应完全线性时, 由于仪器的测量误差, 12CO2和13CO2测量值与真值之间会存在微小的差异, 可以通过线性回归方程进行计算。
\[{{C}_{12,T}}={{a}_{12}}\times {{C}_{12,M}}+{{b}_{12}} \ \ (7) \]
\[{{C}_{13,T}}={{a}_{13}}\times {{C}_{13,M}}+{{b}_{13}} \ \ (8) \]
式中C为CO2的浓度, 下标12、13分别代表12CO2和13CO2, T和M分别为真值和测量值; a12、b12和a13、b13分别为12CO2和13CO2的校正系数。如果不对12CO2和13CO2进行校正直接计算δ值, 那么δ值可以表示为:
\[{{\delta }_{\text{T}}}=\frac{{{a}_{13}}{{C}_{12}}}{{{a}_{12}}{{C}_{12}}+{{b}_{12}}}{{\delta }_{\text{M}}}+\frac{({{a}_{13}}-{{a}_{12}}){{C}_{12}}+{{b}_{13}}-{{b}_{12}}}{{{a}_{12}}{{C}_{12}}+{{b}_{12}}} \ \ (9) \]
根据上述方程可知, 如果b12和b13不为0, 那么方程中的斜率和截距将会随着CO2浓度的变化而变化, 从而导致δ值对CO2浓度的依赖性, 并且在一定CO2浓度范围内表现出非线性响应。因此, 当仪器对12CO2和13CO2浓度的响应为非完全线性时, 也必然会导致δ值的浓度依赖性(Wen et al., 2013)。
根据上述方程, 即使浓度依赖性的响应为非线性的, 在较小的浓度范围内也可近似地认为δ值的浓度依赖性是线性的。例如, Pang等(2016a)研究表明在368.1-550.1 μmol·mol-1浓度范围内基于WS- CRDS技术的G1101-i和G2201-i的δ13C浓度依赖性分别为每100 μmol·mol-1 0.46‰和每100 μmol·mol-1 0.09‰。Guillon等(2012)利用基于OA-ICOS技术的Los Gatos Research DLT-100碳同位素分析仪分别测定1 920 μmol·mol-1 (-43.99‰)和17 800 μmol·mol-1 (-39.37‰)标准气体的逐级稀释气体, 结果表明在300-2 000 μmol·mol-1存在非线性的δ13C浓度依赖性, 2个标准气体在1 000-2 000 μmol·mol-1范围内的浓度依赖性拟合曲线重叠得很好, 这表明在-44‰到-39‰范围内δ13C的浓度依赖性不受CO2同位素组成变化的影响。
理想的校正方法是利用不同δ值的CO2标准气体或固体物质产生多个浓度梯度系列涵盖待测气体CO2浓度和δ值的变化范围, 可有效校正仪器的浓度依赖性。通常CO2标准气体浓度涵盖待测气体CO2浓度的范围比其涵盖δ值的范围更为重要(Bowling et al., 2003; Griffis et al., 2005; Wen et al., 2013)。根据浓度依赖性的定义和产生原因, 可以将校正方法分为理论校正和经验校正方法。
2.2 浓度依赖性的理论校正方法与应用
IRIS仪器可以直接测定12CO2和13CO2或C16O2和C18O16O浓度, 根据浓度依赖性产生的理论原因, 只要分别准确校正12CO2和13CO2或C16O2和C18O16O浓度就可以直接校正仪器的浓度依赖性。因此, 首先需要获得CO2标准气体的12CO2和13CO2或C16O2和C18O16O浓度。对CO2标准气体来说, 从已知总[CO2]和δ13C真值可以获得CO2标准气体中的[12CO2]和[13CO2], 计算公式如下:
\[[C{{O}_{2}}]=[{}^{12}C{{O}_{2}}]+[{}^{13}C{{O}_{2}}]+f[C{{O}_{2}}] \ \ (10) \]
\[{{R}_{a}}={[{}^{13}C]}/{[{}^{12}C]}\;={[{}^{13}C{{O}_{2}}]}/{[{}^{13}C{{O}_{2}}]}\;\]
\[={{R}_{VPDB}}(1+{{\delta }_{a}}/1000) \ \ (11) \]
\[[{}^{12}C{{O}_{2}}]=[C{{O}_{2}}](1-f)/(1+{{R}_{VPDB}}(1+{{\delta }_{a}}/1000)) \ \ (12) \]
\[[{}^{13}C{{O}_{2}}]=[C{{O}_{2}}](1-f)-[{}^{12}C{{O}_{2}}] \ \ (13) \]
其中[CO2]是包括所有同位素的总CO2浓度; f是除了[12CO2]和[13CO2]外其他同位素含量的比例(0.004 74); Ra是CO2标准气体已知13C/12C; RVPDB是国际标准(VPDB)的13C/12C同位素比率(0.011 179 7); δa是CO2标准气体已知δ13C。
同样, 从已知总[CO2]和δ18O真值可以获得CO2标准气体中的[C16O2]和[C18O16O], 计算公式如下:
\[[C{{O}_{2}}]=[{{C}^{16}}{{O}_{2}}]+[{{C}^{18}}{{O}^{16}}O]+f[C{{O}_{2}}] \ \ (14) \]
\[{{R}_{a}}={[{}^{18}O]}/{[{}^{16}O]}\;=0.5\times {[{{C}^{18}}{{O}^{16}}O]}/{[{{C}^{16}}{{O}_{2}}]}\;\]
\[={{R}_{VPDB}}(1+{{\delta }_{a}}/1000) \ \ (15) \]
\[[{{C}^{16}}{{O}_{2}}]=[C{{O}_{2}}](1-f)/(1+2{{R}_{VPDB}}(1+{{\delta }_{a}}/1000)) \ \ (16) \]
\[[{{C}^{18}}{{O}^{16}}O]=[C{{O}_{2}}](1-f)-[{{C}^{16}}{{O}_{2}}] \ \ (17) \]
其中f是除了C16O2和C18O16O外其他同位素含量的比例(0.011 85); Ra是CO2标准气体已知18O/16O, 0.5是C18O16O中存在轻重2个氧原子的比例系数; RVPDB是国际标准的18O/16O同位素比率(0.002 088 349 077)。
通常, 至少需要2个或以上CO2标准气体的CO2浓度涵盖待测目标气体CO2浓度, 同时根据上述公式(10)-(13)计算所需12CO2和13CO2或公式(14)-(17)计算所需C16O2和C18O16O的浓度真值, 建立相应的真值与测量值的函数关系对目标气体12CO2和13CO2或C16O2和C18O16O进行校正。例如, 利用2个CO2标准气体可以分别建立轻重同位素(12CO2和13CO2或C16O2和C16O18O)浓度的真值和测量值的线性函数关系并对目标气体进行校正, 校正方程可以表示为:
\[X_{s,\text{T}}^{L}=\frac{X_{2,T}^{L}-X_{1,T}^{L}}{X_{2,M}^{L}-X_{1,M}^{L}}(X_{s,M}^{L}-X_{1,M}^{L})+X_{1,T}^{L} \ \ (18) \]
\[X_{s,T}^{H}=\frac{X_{2,T}^{H}-X_{1,T}^{H}}{X_{2,M}^{H}-X_{1,M}^{H}}(X_{s,M}^{H}-X_{1,M}^{H})+X_{1,M}^{H} \ \ (19) \]
其中Xs,T、X1,T和X2,T为目标气体、标准气体1和2的摩尔浓度真值; Xs,M、X1,M和X2,M为目标气体、标准气体1和2的摩尔浓度测量值; 上标L和H分别为轻和重同位素。
利用校正后的轻重同位素的摩尔浓度真值直接计算δa值:
\[{{\delta }_{a}}=\left( \frac{{{R}_{a}}}{{{R}_{VPDB}}}-1 \right)\times 1000 \ \ (20) \]
其中, Ra - 13C = [13CO2]/ [12CO2], Ra - 18O = 0.5 × [C18O16O]/ [C16O2]。
少量研究只利用2个CO2 δ13C (或δ18O)标准气体进行浓度依赖性的理论校正, 由于缺乏独立的质控气体, 无法对数据的校正质量进行评价。例如, Bowling等(2003)利用基于TDLAS技术的TGA100在美国犹他州灌溉农田生态系统中开展CO2 δ13C连续观测研究, 采用CO2为363.4 μmol·mol-1 (δ13C为-29.55‰)和1 049.0 μmol·mol-1 (δ13C为-40.58‰) 2个标准气体进行浓度依赖性的理论校正。每个测定循环包括2个样品气体和2个标准气体, 每个测量30 s, 测量循环为2 min。Pang等(2016b)利用基于WS-CRDS技术的Picarro G1101-i对北京城市生态系统大气CO2 δ13C进行测量, 采用CO2为379 μmol·mol-1 (δ13C为-29.36‰)和499 μmol·mol-1 (δ13C为-29.95‰) 2个标准气体进行浓度依赖性的理论校正, 每个测量循环320 min进行一次校正。Wehr等(2013)利用QCLAS技术在美国马萨诸塞州哈佛森林生态系统开展了12CO2、13CO2和C18O16O的4 Hz高频连续观测研究, 采用2个CO2分别为350 μmol·mol-1和450 μmol·mol-1的标准气体进行浓度依赖性的理论校正, 校正频率为40 min。
大部分研究利用3个或者3个以上CO2 δ13C (或δ18O)标准气体进行相互检验, 以探讨不同CO2 δ13C (或δ18O)标准气体校正结果的准确性。例如: Wen等(2013)利用基于WS-CRDS技术的Picarro G1101-i和基于OA-ICOS技术的Los Gatos DLT-100在北京进行城市大气CO2 δ13C测量, 采用3个CO2在361.25- 436.41 μmol·mol-1和δ13C在-10.134 - -8.652‰的标准气体进行浓度依赖性的理论校正, 每个标准气体测量10 min, 每个测量循环30 min。利用每个测量循环的3个标准气体进行互相校正并评价校正结果的准确性, 校正后数据的准确性均优于0.03‰。Pang等(2016a)利用基于WS-CRDS技术的Picarro G1101-i在北京进行城市大气CO2 δ13C测量, 采用CO2为368.1、451.7和550.1 μmol·mol-1, δ13C均为-20.38‰的3个标准气体进行浓度依赖性的理论校正, 每个标准气体测量40 min, 每个测量循环120 min, 同样每个测量循环的3个标准气体进行互相校正并评价校正结果的准确性, 校正后G1101-i和G2201-i的准确性分别为-0.04‰-0.09‰和-0.13‰-0.03‰。需要注意的是, 虽然理论上要求标准气体同位素组成涵盖或接近待测样品气体同位素组成, 但实践中在浓度依赖性的理论校正中标准气体同位素组成是否有差异并涵盖或接近待测样品气体同位素组成不是最重要的, 需要至少保证标准气体CO2浓度能够涵盖样品气体的CO2浓度变化范围。标准气体CO2浓度范围涵盖样品气体CO2浓度时, 校正的准确性更高(Bowling et al., 2003; Wen et al., 2013)。实际数据校正中相互检验后也可以将所有CO2标准气体用于数据校正, 不留专门的质控标准气体, 也可以提高校正精度。
部分相关研究中也专门设置了CO2 δ13C (或δ18O)质控标准气体, 用于评价校正结果的准确性。例如: Griffis等(2005)利用TGA100在美国明尼苏达大学农田生态系统开展大气CO2 δ18O连续观测研究, 采用CO2为348.61 μmol·mol-1 (-2.90‰)和568.44 μmol·mol-1 (-14.89‰) 2个标准气体进行浓度依赖性的理论校正。每2 min的测量循环校正一次。同时, 利用上述2个标准气体和1个质控标准气体进行连续11 h的循环测量, 利用2个标准气体对质控标准气体进行浓度依赖性的理论校正, 研究表明2 min C16O2和C18O16O和δ18O值的测量精度分别为0.09 μmol·
mol-1、0.000 4 μmol·mol-1和0.26‰, 而30 min的测量精度提升到0.02 μmol·mol-1、0.000 1 μmol·mol-1和0.07‰。Bowling等(2005)利用TGA100在美国科罗拉多州亚高山针叶林生态系统开展大气CO2 δ13C连续观测研究, 共用了6个CO2在353.3-477.4 μmol·
mol-1和δ13C在-10.02‰-8.17‰的标准气体, 利用其中2-4个标准气体进行浓度依赖性的理论校正, 校正频率为6 min。研究表明, 3点或者4点线性回归校正即增加回归方程中标准气体的数量能够降低标准气体测量值与真值间的残差。Schaeffer等(2008)利用TGA100对美国AmeriFlux的Niwot Ridge亚高山针叶林生态系统开展大气CO2 δ13C连续观测研究, 采用4个CO2浓度在350-500 μmol·mol-1和δ13C在-8.5‰- -14.5‰的标准气体通过拟合二阶多项式曲线进行浓度依赖性的理论校正, 校正频率为10 min。研究表明, 校正后2个质控标准气体CO2与真值的差异分别为(0.04 ± 0.18) μmol·mol-1和(0.02 ± 0.16) μmol·mol-1, δ13C与真值的差异分别为-0.17‰ ± 0.33‰和-0.14‰ ± 0.34‰。
2.3 浓度依赖性的经验校正方法与应用
在无法准确获得标准气体12CO2和13CO2或C16O2和C18O16O浓度真值而已知δ13C或δ18O真值的条件下, 可以考虑通过直接建立δ13C或δ18O测量值与CO2测定值关系的多项式拟合经验方程, 对浓度依赖性进行校正(Tuzson et al., 2008; Guillon et al., 2012; Sturm et al., 2012)。通常可以直接测定具有相同δ13C (或δ18O)的不同浓度的CO2标准气体进而建立δ测量值与CO2浓度的多项式拟合经验方程; 或者利用单个已知δ13C (或δ18O)真值的高浓度CO2稀释产生不同CO2浓度梯度序列, 然后测定具有相同δ13C (或δ18O)的不同浓度的CO2进而建立δ测量值与CO2浓度的多项式拟合经验方程。例如, 建立3次多项式经验方程的校正方程可以表示为:\[{{\delta }_{std, M}} =a {{\left( C{{O}_{2std, M}} \right)}^{3}}+b{{\left( C{{O}_{2std, M}} \right)}^{2}}+\] \[c\left( C{{O}_{2std, M}} \right)+d \ \ (21) \]
其中a、b、c和d分别为经验方程拟合系数, 下标中std表示CO2标准气体, M表示测量值。
通常建立上述多项式经验拟合方程后, 可以直接利用该方程和δ13C或δ18O真值直接进行经验浓度依赖性校正, 或利用该函数关系将所有测定数据标准化到某CO2浓度(如400 μmol·mol-1)进行经验浓度依赖性校正, 然后再建立该CO2浓度下CO2 δ13C (或δ18O)标准气体的测量值与真值的单点、两点或多点函数关系并进行δ值校正(Tuzson et al., 2008; Guillon et al., 2012; Sturm et al., 2012)。
例如, Tuzson等(2008)利用QCLAS技术在瑞士中部苏黎世草地生态系统测定大气CO2 δ13C和δ18O。采用4个CO2浓度在1 000-1 970 μmol·mol-1和δ13C在-37‰ - -4‰的标准气体分别进行稀释实验, 研究表明不同同位素组成的标准气体测试的浓度依赖性均表现出δ值与CO2浓度的线性相关, 不受标准气体同位素组成差异的影响。因此在校正过程中2个标准气体用于确定浓度依赖性的线性经验方程。Sturm等(2012)利用QCLAS技术在瑞士L?geren森林生态系统进行大气12CO2、13CO2和CO18O通量的连续原位测量, 利用稀释法产生δ值相同而CO2浓度在300-500 μmol·mol-1的标准气体进行浓度依赖性的经验方程校正。Wada等(2011)利用QCLAS技术对日本名古屋城市大气CO2 δ13C和δ18O进行测量, 首先利用1个CO2标准气体在实验前通过一次稀释实验进行仪器浓度依赖性的经验方程校正, 仪器表现出线性的浓度依赖性,CO2浓度每改变20 μmol·mol-1时, 相应的δ13C变化为-4‰。Sturm等(2013)利用QCLAS技术在瑞士阿尔卑斯山少女峰进行大气CO2δ13C和δ18O观测, 每30 min测定CO2δ13C和δ18O标准气体5 min以校正仪器的时间漂移; 随后每5天利用1个标准气体通过稀释试验进行浓度依赖性的经验方程校正。
3 时间漂移的校正原理、方法和应用
3.1 时间漂移产生的原因
时间漂移是指IRIS仪器持续测量具有相同δ13C (或δ18O)的同一浓度的CO2标准气体时表现出的δ测量值随时间的变化特征。仪器运行过程中, 激光频率和强度的改变、腔室内气体温度和压力波动以及激光老化等仪器自身原因都会造成δ测量值的时间漂移(Sturm et al., 2013), 外界温度、湿度等环境参数变化的影响也会造成δ测量值的时间漂移(Guillon et al., 2015)。通常, 为了提高仪器测量结果的稳定性, 减小仪器时间漂移的幅度, 仪器制造商会对仪器的温度、压力和激光等进行精确控制。同时, 在仪器的使用过程中, 仪器使用者需要尽可能控制仪器周围的环境参数稳定, 如有可能建议对CO2气体进行干燥, 以保持样品的水汽浓度维持在较低的水平(Guillon et al., 2015)。IRIS仪器对环境条件变化(如温度和压力等)的敏感性会导致仪器δ测量值的时间漂移, 即同一浓度和δ值的CO2标准气体在不同时间的测量结果会发生变化(Schaeffer et al., 2008; Pang et al., 2016a)。Vogel等(2013)利用2个CO2 δ13C标准气体持续48 h的切换测试(分别测量10 min和20 min), 发现基于WS-CRDS技术的Picarro G1101-i在一天内的漂移能够达到0.3‰。此外, 浓度依赖性特征也会随着时间发生变化和漂移。Guillon等(2015)研究表明, 基于OA-ICOS技术的Los Gatos Research CCIA-EP在0-12天的不同时间间隔重复进行CO2 δ13C浓度依赖性测试, 在日尺度上保持着相似的形状, 但是会表现出随机的时间漂移, 24 h的漂移可以达到5‰。
3.2 时间漂移的校正方法与应用
通过设置不同的仪器校正频率可以有效地校正仪器的时间漂移。校正频率是综合权衡仪器时间漂移、最大校正精度和最小校正时间消耗后的最优校正间隔(Aemisegger et al., 2012; Vogel et al., 2013)。为了消除仪器时间漂移的影响, 可以通过Allan方差测试对仪器性能的测试确定适当的校正频率, 也可以通过设置一定的时间间隔对CO2 δ13C (或δ18O)标准气体进行动态测量确定适当的校正频率(Bowling et al., 2003; Griffis et al., 2005; Vogel et al., 2013)。以往研究已经开展对IRIS仪器的稳定性和精度的各种测试研究, 基于这些仪器稳定性和精度的测试结果可以确定适当的时间漂移校正频率, 以获得符合试验精度需求的校正结果。例如, Guillon等(2012)对基于OA-ICOS技术的Los Gatos Research DLT-100碳同位素分析仪进行Allan方差测试, 研究表明仪器最优精度的平均时间为200 s, CO2浓度和δ13C精度分别为2 μmol·mol-1和0.04‰。Wen等(2013)对基于WS-CRDS技术的Picarro G1101-i和基于OA-ICOS技术的Los Gatos DLT100进行Allan方差测试, 研究表明12CO2的最优精度分别为0.013 μmol·
mol-1和 0.016 μmol·mol-1, 平均时间为1 800 s和500 s; δ13C的最优精度分别为0.08‰和0.04‰, 平均时间为2 000 s和1 000 s。Vogel等(2013)对基于WS-CRDS技术的Picarro G1101-i进行Allan方差测试, 研究表明60 min平均时间的测量精度能够达到0.1‰。同时, 利用2个CO2 δ13C标准气体分别测量 10 min和20 min的切换测试, 表明2个标准气体的漂移状况相似, 在一天内约为0.3‰。Pang等(2016a)对基于WS-CRDS技术的G1101-i和G2201-i的Allan方差测试, 研究表明G1101-i和G2201-i的δ13C最优精度分别为0.08‰-0.15‰和0.01‰-0.04‰, 平均时间为1 900-7 600 s和3 800-7 600 s。
前面阐述的浓度依赖性的理论校正和经验校正均指某个时刻的浓度依赖性校正而未涉及时间漂移问题。通常可以直接设置固定的校正频率, 定期测量CO2标准气体进行浓度依赖性的理论或经验动态校正, 这样在浓度依赖性校正的同时也对仪器的时间漂移进行了校正(Bowling et al., 2003, 2005; Griffis et al., 2005; Schaeffer et al., 2008; Pang et al., 2016b)。还有研究者为了提高时间漂移校正的准确性, 定期测量CO2标准气体的CO2浓度和δ13C, 利用相邻两个时间的测量结果分别拟合标准参考气体CO2浓度和δ13C随时间变化的线性回归方程, 通过时间线性内插以获得所需更高校正频率的CO2浓度和δ13C (或δ18O)时间序列数据, 利用内插后生成的标准气体数据对样品测量结果进行校正, 从而进一步减少时间漂移的校正误差(Wehr et al., 2013; Guillon et al., 2015)。为了减少校正误差, 在校正时也利用平滑样条对每个测量循环的校正系数进行平滑处理, 然后应用平滑后的校正系数对数据进行校正(Sturm et al., 2012, 2013)。
也有研究者单独设置CO2标准气体对仪器的时间漂移进行校正(Tuzson et al., 2008; Sturm et al., 2012, 2013)。例如, Tuzson等(2008)利用QCLAS技术在瑞士中部苏黎世草地生态系统测定大气CO2 δ13C和δ18O。校正过程中设置3个标准气体, 2个用于确定浓度依赖性的线性经验方程, 另外1个用于时间漂移校正, 校正频率为30 min。在整个观测时期内校正后δ13C和δ18O的精度和准确性都在0.2‰左右。Wada等(2011)利用QCLAS技术对日本名古屋城市大气CO2 δ13C和δ18O进行测量, 首先进行仪器浓度依赖性的经验方程校正, 随后每30 min采用2个参考气体(CO2 = 498.34 μmol·mol-1, δ13C = -12.68‰, δ18O = -9.71‰; CO2 = 413.28 μmol·mol-1, δ13C = -9.11‰; δ18O = -7.07‰)进行两点时间漂移校正。Sturm等(2012)利用QCLAS技术在瑞士L?geren森林生态系统进行大气12CO2、13CO2和CO18O通量的连续原位测量, 利用浓度依赖性的经验方程校正, 然后利用2个已知CO2浓度和δ13C (或δ18O)的校正气体进行两点时间漂移校正。校正频率为1 h, 其中5 min测量校正气体, 55 min测量样品气体。5 min的校正气体测量包括稀释校正120 s, 以及2个标准气体和1个质控气体分别测量60 s。在连续3周的野外观测中, 每1 h一次校正后, δ13C和δ18O测量结果的精度为0.15‰。Sturm等(2013)利用QCLAS技术在瑞士阿尔卑斯山少女峰进行大气CO2δ13C和δ18O观测, 每隔30 min测定CO2δ13C和δ18O标准气体5 min以校正仪器的时间漂移; 随后每5天利用1个标准气体通过稀释试验进行浓度依赖性的经验方程校正。在平均时间为10 min时, δ13C和δ18O的测量精度均可以达到0.02‰, 准确性约为0.1‰。
4 数据溯源的校正原理、方法和应用
IRMS是相对测量技术, 而IRIS是绝对测量技术。所有的IRMS和IRIS仪器测定的δ值均需要利用1个或者多个标准物质溯源到同位素国际标准, 这一过程称为数据溯源。IRIS技术实现了对空气CO2 δ13C和δ18O的连续测定, 但需要IRMS提供数据溯源至CO2碳或氧同位素国际标准(Vienna Pee Dee Belemnite)的CO2 δ13C和δ18O标准气体(Wen et al., 2013; Pang et al., 2016a; Wang et al., 2017)。数据溯源方法通常包括单点溯源和两点或多点溯源:
(1) 单点溯源: 利用单个已知δ值的标准物质(δstd,T)溯源, 只适用于标准物质与样品δ值较为接近的情况。样品的真值δs,T为:
式中下标T和M分别为真值和测定值, s和std分别为样品和标准物质。
(2)两点或多点溯源: 建立两个或多个涵盖样品δ值的标准物质真值(δstd,T)和测定值(δstd,M)的线性关系, 获得线性回归拟合参数并用于对样品进行数据溯源, 样品的真值δT,s为:
其中, a和b分别为线性回归方程的拟合参数。
在前面阐述的浓度依赖性和时间漂移的校正中, 如果采用的已知CO2浓度和δ13C (或δ18O)值的标准气体已经溯源国际标准, 则在浓度依赖性和时间漂移校正的同时就实现了数据溯源(Bowling et al., 2003, 2005; Griffis et al., 2005; Schaeffer et al., 2008; Wen et al., 2013; Pang et al., 2016a, 2016b)。如果上述浓度依赖性和时间漂移校正CO2标准气体未溯源至国际标准, 则需要利用已知δ13C (或δ18O)值的校正气体进行单点、两点或者多点数据溯源(Tuzson et al., 2008; Wada et al., 2011; Sturm et al., 2012, 2013)。例如, Sturm等(2013)利用QCLAS技术在瑞士阿尔卑斯山少女峰进行大气CO2δ13C和δ18O观测, 每6 h测量3个标准气体, 其中2个进行数据溯源, 另1个用于检查校正的稳定性。
5 研究展望
5.1 加强不同IRIS仪器和校正方法的比对和一致性研究
由于IRIS仪器直接测量每个核素的浓度, 单个核素浓度值的测定误差导致了IRIS仪器的浓度依赖性(Griffith et al., 2012)。此外, 由于仪器的时间漂移, 需要设置一定的校正频率进行定期校正以消除仪器漂移的影响。以往研究主要集中在单台IRIS仪器的方法论研究方面(Bowling et al., 2003; Tuzson et al., 2008; Griffith et al., 2012; Guillon et al., 2012; Vogel et al., 2013)。较少关注IRIS仪器之间和不同校正方法之间的对比研究(Griffis, 2013; Wen et al., 2013), 仅有Wen等(2013)对两个IRIS技术的CO2 δ13C分析仪的对比研究表明Los Gatos DLT-100和Picarro G1101-i对大气CO2δ13C测量结果标定后的一致性在-0.02‰ ± 0.18‰以内, 然而这种微小的差异由于存在一定的浓度依赖性会导致Keeling Plot的分析误差达到2.44‰。不同的IRIS仪器设备之间测量结果如果存在系统偏差, 会导致不同仪器测量结果不具有可比性(Flowers et al., 2012; Hammer et al., 2013; Griffis, 2013; Wen et al., 2013)。在今后的研究中, 需要重点关注不同IRIS仪器和不同校正方法间的对比研究, 加强数据质量控制, 确保不同测量结果间的准确性、一致性和可比性。推荐至少利用3个或3个以上已知CO2浓度和δ真值的标准气体涵盖待测CO2浓度校正浓度依赖性, 设置适当的校正频率校正时间漂移并溯源至国际标准。目前, IRIS技术的测量结果经过浓度依赖性和时间漂移校正后准确性能够达到0.01‰ ± 0.03‰ (Bowling et al., 2005), 与IRMS相当。5.2 H2O、CH4和N2O等IRIS技术面临同样的校正难题
随着IRIS技术的发展, 除了CO2外, 也可以实现H2O、CH4和N2O等多种同位素气体的原位连续测量, 在这些测量中同样需要考虑仪器的浓度依赖性和时间漂移等误差来源, 并通过适当的校正策略获得精度和准确度均满足研究需要的测量结果(Wen et al., 2008, 2012; Keppler et al., 2010; Mohn et al., 2012, 2014; Yamamoto et al., 2014), 例如: Sturm和 Knohl (2010)发现基于OA-ICOS技术的Los Gatos DLT-100在2 000-27 000 μmol·mol-1 H2O浓度范围内氘同位素组成(δD)和δ18O测量值存在着明显的浓度依赖性。Aemisegger等(2012)发现基于WS-CRDS技术的Picarro L1115-i测量中, 由于气体样品中水汽浓度的变化导致的δD和δ18O的偏差能够分别达到4‰和2‰。Yamamoto等(2014)发现基于QCLAS技术在1 000-2 500 nmol·mol-1的N2O浓度范围内δ15Nα和δ15Nβ存在明显的浓度依赖性。这些浓度依赖性可以通过理论校正或者经验方程校正予以消除(Wen et al., 2008; Aemisegger et al., 2012)。Kurita等(2012)发现基于OA-ICOS技术的Los Gatos DLT-100连续4个月重复测量水汽标准δD和δ18O的漂移范围分别在-77‰ - -82‰和-8.0‰ - -12.5‰之间。Keppler等(2010)发现基于OA-ICOS技术在连续6天的试验期间δ13CCH4的漂移达到0.84‰。利用与CO2相似的校正方法, 可以对H2O、CH4和N2O等多种同位素测量结果进行浓度依赖性和时间漂移校正, 以获得可追溯到国际标准的原位连续测量数据, 为在不同时空尺度上研究植被-大气碳水交换的生物物理过程、甲烷生产/消耗、硝化/反硝化作用的环境生物控制机制提供新的机遇。5.3 IRIS技术在生态学中的应用潜力
IRIS技术的发展带来的CO2碳氧同位素的原位连续观测极大地拓展了我们对生态系统、区域和大陆尺度影响碳收支的生物物理过程的研究和解释。IRIS技术与涡度协方差、通量廓线和动态箱式技术相结合可以实现CO2碳氧同位素比值和通量的原位连续观测(Griffis et al., 2004, 2005; Zhang et al., 2006; Santos et al., 2012), 有助于我们深入理解生态系统碳周转、分配和利用等一系列复杂的生物物理过程及其环境和生物控制机制。例如, Wingate等(2010)利用TDLAS技术与通量廓线和动态箱式技术结合, 研究表明茎秆、土壤和生态系统呼吸的CO2 δ13C比光合δ13C分馏存在更小的短期变异并存在2-10天的滞后效应。Wehr等(2016)利用QCLAS技术与涡度协方差技术结合进行通量拆分, 研究表明传统拆分方法高估了生长季前期的生态系统光合和白天呼吸, 错误地描述了生态系统光能利用效率。在全球通量网络(Fluxnet)中, 同位素观测相对较少且多限制在短期集中观测。随着IRIS技术的发展, 未来在更多的通量观测站点结合进行碳氧同位素的野外连续原位观测, 将有助于提升我们对生态系统和大气间碳水交换生物物理过程的理解(Griffis, 2013)。此外, 通过野外同位素标记示踪实验结合原位连续观测, 可以有效地评价和验证同位素生态系统模型(例如SiLSM和CLM-CN 3.5)中的光合作用、呼吸、碳库和分配过程与机制等。
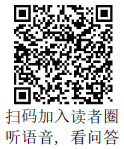
参考文献 原文顺序
文献年度倒序
文中引用次数倒序
被引期刊影响因子
δ
DOI:10.5194/amt-5-1491-2012URL [本文引用: 2]

Variations of stable water isotopes in water vapour have become measurable at a measurement frequency of about 1 Hz in recent years using novel laser spectroscopic techniques. This enables us to perform continuous measurements for process-based investigations of the atmospheric water cycle at the time scales relevant for synoptic meteorology. An important prerequisite for the interpretation of data from automated field measurements lasting for several weeks or months is a detailed knowledge about instrument properties and the sources of measurement uncertainty. We present here a comprehensive characterisation and comparison study of two commercial laser spectroscopic systems based on cavity ring-down spectroscopy (Picarro) and off-axis integrated cavity output spectroscopy (Los Gatos Research). The uncertainty components of the measurements were first assessed in laboratory experiments, focussing on the effects of (i) water vapour mixing ratio, (ii) measurement stability, (iii) uncertainties due to calibration and (iv) response times of the isotope measurements due to adsorption-desorption processes on the tubing and measurement cavity walls. Based on the experience from our laboratory experiments we set up a one-week field campaign for comparing measurements of the ambient isotope signals of the two laser spectroscopic systems. The optimal calibration strategy determined for both instruments was applied as well as the correction functions for water vapour mixing ratio effects. The root mean square difference between the isotope signals from the two instruments during the field deployment was 2.3‰ for δ2H, 0.5‰ for δ18O and 3.1‰ for deuterium excess. These uncertainty estimates from field measurements compare well to those found in the laboratory experiments. The present quality of measurements from laser spectroscopic instruments combined with a calibration system opens new possibilities for investigating the atmospheric water cycle and the land-atmosphere moisture fluxes.
DOI:10.1093/jxb/erq137URLPMID:2905191 [本文引用: 1]

Mesophyll conductance to CO2(gm) limits carbon assimilation and influences carbon isotope discrimination (Δ) under most environmental conditions. Current work is elucidating the environmental regulation ofgm, but the influence ofgmon model predictions of Δ remains poorly understood. In this study, field measurements of Δ andgmwere obtained using a tunable diode laser spectroscope coupled to portable photosynthesis systems. These data were used to test the importance ofgmin predicting Δ using the comprehensive Farquhar model of Δ (Δcomp), wheregmwas parameterized using three methods based on: (i) meangm; (ii) the relationship between stomatal conductance (gs) andgm; and (iii) the relationship between time of day (TOD) andgm. Incorporating meangm,gs-basedgm, and TOD-basedgmdid not consistently improve Δcomppredictions of field-grown juniper compared with the simple model of Δ (Δsimple) that omits fractionation factors associated withgmand decarboxylation. Sensitivity tests suggest thatb, the fractionation due to carboxylation, was lower (25‰) than the value commonly used in Δcomp(29‰) and Δsimple(27‰). These results demonstrate the limits of all tested models in predicting observed juniper Δ, largely due to unexplained offsets between predicted and observed values that were not reconciled in sensitivity tests of variability ingm,b, ore, the day respiratory fractionation.
DOI:10.1029/2004GB002394URL [本文引用: 6]

[1] The dynamics of forest-atmosphere CO2 carbon isotope exchange were examined in a coniferous forest in Colorado, United States. Tunable diode laser absorption spectrometry provided extensive characterization of the carbon isotope content (020713C) of CO2. Observed patterns in 020713C of forest air were associated with photosynthesis, respiration, and atmospheric boundary layer dynamics. Similar relationships between 020713C and CO2 were observed at all forest heights and confined to a relatively narrow envelope. Substantial variation was observed in the isotope ratio of nocturnal ecosystem respiration (020713CR, calculated from isotopic mixing lines). A systematic bias was identified when estimating 020713CR from data sets with small range in CO2 in the samples, leading us to restrict analysis of 020713CR to periods with CO2 range >40 0204mol mol0908081. Values of 020713CR varied from 09080828.1 to 09080825.20900°, with variation from one night to the next as large as 1.70900°. A consistent difference was observed between 020713CR calculated near the forest floor (<2 m height) versus the upper canopy (509000911 m) on the same nights. 020713CR was more enriched in the upper canopy than near the ground on 34 of 43 nights, with a mean enrichment of 0.60900° and a maximum of 2.30900°. A similar pattern was observed comparing 020713CR at night with the analogous quantity calculated during daytime, but only a few daytime periods met the 40 0204mol mol0908081 criterion. Comparisons between air samples measured (1) 10 m above the forest canopy, (2) 3 km away, and (3) within the convective boundary layer 125 km distant showed CO2 differences between sites as large as 50900096 0204mol mol0908081 even at midday. These results suggest that attempts to use flask measurements at remote monitoring stations as a proxy for the air directly interacting with a vegetation canopy should be made with caution. However, our results also suggest that substantial information about biosphere-atmosphere isotopic exchange can be obtained by simultaneous examination of CO2 and 020713C at multiple spatial scales.
DOI:10.1016/S0168-1923(03)00074-1URL [本文引用: 10]

The stable isotope content of atmospheric CO 2 provides information about ecosystem carbon–water relations and biosphere–atmosphere carbon exchange. Virtually every isotope study within these fields has required air sample collection at remote locations followed by isotope analysis at a laboratory. This requirement severely limits sampling frequency and experiment duration. In this paper, we evaluate a tunable diode laser absorption spectrometer (TDL) for measuring the carbon isotope content of CO 2 at atmospheric mole fractions (350–700 μmol mol 611) and isotopic abundance ( δ 13 C of 616 to 6116‰). Using infrared absorption, the TDL system determines the mole fractions of 12 CO 2 and 13 CO 2 independently, rather than their ratio as in mass spectrometry (MS). The ability of the instrument to measure isotope ratios ( δ 13 C) was tested outdoors in a grassland and compared to standard laboratory-based MS measurements made on field-collected flask samples. The TDL was operated at a sampling flow rate of 230 ml min 611 and a sampling interval of 2 min for two intake heights. There was a consistent offset for δ 13 C of 1.77‰ between the TDL and MS measurements, and the standard deviation of the error (MS61TDL) was 0.35‰ ( n=82). Removal of two outliers improved this standard deviation to 0.25‰ ( n=80). After removing the offset, 62 out of 82 samples had absolute differences less than 0.3‰. Subsequent laboratory experiments indicated that the TDL/MS offset was caused by pressure broadening, and can be avoided in the future by calibrating the TDL with CO 2 mixed with air rather than nitrogen. Based on these results we estimate the precision for δ 13 C to be 0.25‰ for our sampling scheme. A similar comparison with flask-based measurements of CO 2 mole fraction ( 12 CO 2+ 13 CO 2) made with a calibrated infrared gas analyzer indicated a TDL precision of 0.4% (1.6 μmol mol 611 at 400 μmol mol 611). The TDL was used to investigate the vertical and temporal variation in the carbon isotope content of respired CO 2 ( δ 13 C R) from the grassland. Measurements of δ 13 C of CO 2 in air were made during four separate nights at 1 and 60 cm height above ground. δ 13 C R did not vary with height, but it did vary from one night to the next. Hourly measurements of δ 13 C R showed it changed as much as 6.4‰ (6129.1±0.4 to 6122.7±0.8‰) in a single night. Temporal changes in δ 13 C R during the night have not been reported in prior studies. Such observations could provide a new way to investigate temporal dynamics of the carbon substrates utilized for ecosystem respiration.
DOI:10.1016/j.agrformet.2017.08.002URL [本文引用: 1]

react-text: 112 Generally, system parameters that are nonlinear in the model output are estimated by nonlinear least-squares (NLS) optimization algorithms. As an alternative, for nonlinear discrete-time models with a so-called rational structure in input, output, and parameters, in this paper a method is proposed to re-parameterize the model such that the model becomes linear in its new parameters.... /react-text react-text: 113 /react-text [Show full abstract]
DOI:10.5194/amt-5-991-2012URL [本文引用: 1]

Tunable diode laser absorption (TDL) and cavity ring-down spectroscopic (CRDS) sensors for atmospheric carbon dioxide were co-deployed during summer and fall of 2010 in field and laboratory conditions at Los Alamos National Laboratory. Both sensors were characterized for accuracy and precision for ambient carbon dioxide measurements at ground level and compared using both laboratory and ambient field data. After post-processing that included water vapor correction and calibration to WMO reference standards, overall mean [lt;supgt;12lt;/supgt;Clt;supgt;16lt;/supgt;Olt;subgt;2lt;/subgt;] = 392.05 ± 8.92 ppm and [lt;supgt;12lt;/supgt;Clt;supgt;16lt;/supgt;Olt;subgt;2lt;/subgt;] = 392.22 ± 9.05 ppm were observed between 29 July and 16 August 2010. The mean difference between the CRDS and TDL data for lt;supgt;12lt;/supgt;COlt;subgt;2lt;/subgt; was 0.04 ± 1.80 ppm (±1σ in 60 s) for ambient field data, demonstrating the sensors meet the WMO/IAEA compatibility standard. The observations show over the 19-day period the [COlt;subgt;2lt;/subgt;]lt;subgt;CRDSlt;/subgt;/[COlt;subgt;2lt;/subgt;]lt;subgt;TDLlt;/subgt; ratio exhibits a Gaussian distribution centered at lt;igt;xlt;/igt;lt;subgt;0lt;/subgt; = 1.003 ± 3.38 × 10lt;supgt;amp;minus;5lt;/supgt; (±1σ), indicating the ratio is dominated by random noise as opposed to a bias in the output of either sensor. The CRDS sensor is capable of measuring [lt;supgt;12lt;/supgt;Clt;supgt;16lt;/supgt;Olt;subgt;2lt;/subgt;] to a precision of 23 ppb in 1 min and decreases to 6.5 ppb in 58 min. At one and 58-min, the TDL exhibits precisions of 29 ppb and 53 ppb. The CRDS is compact, fast, and stable; the TDL is larger and requires frequent calibrations to maintain its precision. The sensors also exhibit consistent hourly averaged diurnal values underscoring the interplay of biological, anthropogenic, and transport processes regulating COlt;subgt;2lt;/subgt; at the site.
DOI:10.1016/j.agrformet.2013.02.009URL [本文引用: 7]

Development of optical isotope techniques over the last several years has provided scientists a set of tools for tracing the transport and cycling of CO2 and water vapor between the biosphere and atmosphere. Here, I take a micrometeorological perspective and review these technological advances, assess key instrument performance characteristics, examine how these techniques have been used in the field to improve our understanding of the processes governing the exchange of CO2 and water vapor, and discuss future research directions. Review of the recent literature indicates that: (1) optical techniques have been used to quantify the isotope composition of biosphere–atmosphere exchange using the traditional Keeling mixing line, flux–gradient, eddy covariance, and chamber approaches under a variety of field conditions with near-continuous data records now extending to more than 5-years; (2) high frequency and near continuous isotope measurements at the canopy scale have demonstrated important new insights regarding the behaviour of kinetic fractionation at the leaf versus canopy scales and the controls on ecosystem respiration that could not have been observed previously using traditional methods; (3) based on the assessment of instrument performance, carbon isotope disequilibrium (the difference between the isotope composition of photosynthesis and respiration), carbon turnover rates, and measurement uncertainties, 13C-CO2 investigations are best suited for examining the contributions and changes to ecosystem respiration with a need for more innovative 13C-isotope labeling experiments and compound specific isotope analyses under field conditions; (4) significant progress has been made in measuring the oxygen isotope composition of water vapor fluxes and canopy leaf water enrichment. These new data have provided an opportunity to evaluate models of leaf water enrichment and their application to the canopy scale; and (5) the use of 18O-H2O and 18O-CO2 as tracers of the coupled carbon–water cycle has matured significantly in recent years. Evidence from a range of ecosystems indicates that 18O-CO2 disequilibrium (ranging from 0 to 17‰) is much larger than for 13C-CO2 (typical less than 3‰ in natural C3 ecosystems) making it a useful tracer of coupled carbon and water cycle processes. However, a better understanding of the role of carbonic anhydrase in photosynthetic and respiratory processes under field conditions is now needed in order to make further progress. Finally, the increasing use and development of isotope-enabled land surface schemes along with the acquisition of high temporal resolution isotope data is providing a new opportunity to constrain the carbon and water cycle processes represented in these models.
DOI:10.1016/j.agrformet.2004.01.009URL [本文引用: 1]

The combination of micrometeorological and stable isotope techniques offers a relatively new approach for elucidating ecosystem-scale processes. Here we combined a micrometeorological gradient technique with tunable diode laser absorption spectroscopy (TDLAS) using the Trace Gas Analyzer (TGA100, Campbell Scientific, Inc., Utah, USA) to measure field-scale isotopic CO 2 mixing ratios and fluxes of 12 CO 2 and 13 CO 2. The experiment was conducted in a recently harvested soybean ( Glycine max) field that had been in corn ( Zea mays) production the previous 4 years. Measurements were made over a period of 26 days from October 25 to November 19, 2002. Weather conditions were unusually cold and dry during the experiment. Isotopic gradients were small and averaged 610.153 and 610.0018 μmol mol 611 m 611 for 12 CO 2 and 13 CO 2, respectively for u 65>0.1 m s 611. The average 12 CO 2 and 13 CO 2 flux for the period was 1.0 and 0.012 μmol m 612 s 611, respectively. The isotope ratio of respired carbon ( δ 13 C R) obtained from the linear intercept of a Keeling plot was 6127.93‰ (±0.32‰) for the experimental period. The Keeling plot technique was compared to a new flux ratio methodology that estimates δ 13 C R from the slope of a linear plot of 13 CO 2 versus 12 CO 2 flux. This method eliminates a number of potential limitations associated with the Keeling plot and provides a δ 13 C R value that can be directly related to the flux footprint. In this initial comparison, our analysis showed that the flux ratio method produced a similar δ 13 C R value (6128.67‰), but with greater uncertainty (±2.1‰). Better results are expected during growing season conditions when fluxes are substantially larger and the signal to noise ratio is improved. The isotope ratio of respired carbon was consistent with C 3 agricultural systems indicating that soybean decomposition was the dominant substrate for respiration. The observed increase in ecosystem respiration ( R E) and decrease in δ 13 C R following tillage indicated that the incorporation of fresh soybean residue provided the major source for decomposition and further illustrates that the combination of micrometeorological and stable isotope techniques can be used to better interpret changes in carbon cycle processes. Long-term and continuous measurements of isotopic CO 2 exchange using tunable diode laser absorption spectroscopy and micrometeorological techniques offers a new opportunity to study carbon cycle processes at the field-scale.
DOI:10.1016/j.agrformet.2005.10.002URL [本文引用: 6]

Stable isotopes of carbon dioxide (CO 2) can be used as natural tracers to better understand carbon cycle processes and exchange pathways between the biosphere and atmosphere. In this study, we used a tunable diode laser (TDL) technique for continuous fast measurement of the stable isotopomers C 18O 16O and C 16O 2 and their ratio, δ a. The TDL system was configured to measure mixing ratios of [C 16O 2] and [C 18O 16O] at wavenumber frequencies of 2308.225 and 2308.416 cm 611, respectively. Two-minute precision (1 standard deviation) was 0.0004, 0.09 μmol mol 611, and 0.26‰ for [C 18O 16O], [C 16O 2] and δ a, respectively. Comparison of TDL and mass spectrometry flask measurements showed relatively good agreement ( r 2 = 0.94) with a standard deviation of 0.55‰ for the residual values. Estimates of the isotope signature of ecosystem flux components over a soybean ( Glycine max) field were examined. These data represent one of the first continuous flux measurements of C 18O 16O. The isotope signature of net ecosystem CO 2 exchange at night ranged from 6115 to 617‰ and during the daytime from 6140 to 6120‰. A simple estimate of canopy-scale photosynthetic discrimination showed significant diurnal variation and averaged 10.5‰ (±8.8‰). The large difference between the isotope signature of respiration and midday canopy photosynthesis represented significant isotopic disequilibrium. Coupled with recent advances in measuring water vapor isotopomers with the TDL technique, a new opportunity is emerging to better understand the dynamics, complex interactions, and discrimination mechanisms controlling land-atmosphere C 18O 16O exchange.
DOI:10.5194/amtd-5-3717-2012URL [本文引用: 4]

Concern in recent decades about human impacts on Earths climate The isotopic specificity of the optically-based technique and analysis allows application of the analyser in isotopic tracer experiments, for example lt;supgt;13lt;/supgt;C in COlt;subgt;2lt;/subgt; and eview a number of applications illustrating use of the analyser in clean air monitoring, micrometeorological flux and tower measurements, mobile measurements on a train, and soil flux chamber measurements.
DOI:10.1007/s00340-015-6013-4URL [本文引用: 5]

CO 2 stable carbon isotopes are very attractive in environmental research to investigate both natural and anthropogenic carbon sources. Laser-based isotope ratio infrared spectrometers (IRIS) allow in situ continuous monitoring of CO 2 isotopes, and therefore they have a potential for unprecedented understanding of carbon sources and dynamics with a high temporal resolution. Here we present the performance assessment of a commercial IRIS analyzer, including the measurement setup and the data processing scheme that we used. Even if the analyzer performs 1-Hz measurements, an integration time of the order of 1h is commonly needed to obtain acceptable precision for 13 C. The main sources of uncertainty on 13 C come from the concentration dependence and from the temporal instability of the analyzer. The method is applied to the in situ monitoring of the CO 2 carbon isotopes in an underground cavity (Roselend Natural Laboratory, France) during several months. On a weekly timescale, the temporal variability of CO 2 is dominated by transient contamination by human breath. Discarding these anthropogenic contaminations, CO 2 and 3 C backgrounds do not show diurnal or seasonal fluctuations. A CO 2 flux released into the tunnel by the surrounding rocks is measured. The carbon isotope composition of this CO 2 , identified with a Keeling plot, is consistent with a main production by microbial respiration and a minor production from weathering of carbonate minerals. The presented instrument and application study are relevant to cave monitoring, whether to understand CO 2 dynamics in visited and/or painted caves for preservation purposes or to understand paleoclimate recording in speleothems.
DOI:10.1007/s00340-012-4942-8URL [本文引用: 7]

CO 2 stable carbon isotopes are very attractive in environmental research to investigate both natural and anthropogenic carbon sources. Laser-based CO 2 carbon isotope analysis provides continuous measurement at high temporal resolution and is a promising alternative to isotope ratio mass spectrometry (IRMS). We performed a thorough assessment of a commercially available CO 2 Carbon Isotope Analyser (CCIA DLT-100, Los Gatos Research) that allows in situ measurement of δ 13 C in CO 2 . Using a set of reference gases of known CO 2 concentration and carbon isotopic composition, we evaluated the precision, long-term stability, temperature sensitivity and concentration dependence of the analyser. Despite good precision calculated from Allan variance (5.002ppm for CO 2 concentration, and 0.0502‰ for δ 13 C at 60 s averaging), real performances are altered by two main sources of error: temperature sensitivity and dependence of δ 13 C on CO 2 concentration. Data processing is required to correct for these errors. Following application of these corrections, we achieve an accuracy of 8.702ppm for CO 2 concentration and 1.302‰ for δ 13 C, which is worse compared to mass spectrometry performance, but still allowing field applications. With this portable analyser we measured CO 2 flux degassed from rock in an underground tunnel. The obtained carbon isotopic composition agrees with IRMS measurement, and can be used to identify the carbon source.
DOI:10.5194/amtd-5-3645-2012URL [本文引用: 1]

We thoroughly evaluate the performance of a multi-species, in-situ FTIR analyser with respect to high accuracy needs for greenhouse gas monitoring networks. The in-situ FTIR analyser measures fractions continuously, all with better reproducibility than requested by the WMO-GAW inter-laboratory compatibility (ILC) goal. Simultaneously deter reaches reproducibility as good as 0.03amp;permil;. This paper focuses on the quantification of residual dependencies between the measured components and the thermodynamic properties of the sample as well as the cross-sensitivities among the sample constituents. The instrument has proven to be linear for all components in the ambient range. The temporal stability of the instrument was investigated by 10 months of continuously collected quality control measures. Based on these measures we conclude that for moderately stable laboratory conditions weekly calibrations of the instrument are sufficient to reach WMO-GAW ILC goals.
DOI:10.1021/es100460dURLPMID:20540538 [本文引用: 1]

Methane production by anaerobic digestion of biomass has recently become more attractive because of its potential for renewable energy production. Analytical tools are needed to study and optimize the ongoing processes in biogas reactors. It is considered that optical methods providing continuous measurements at high temporal resolution of carbon isotope ratios of methane (delta(13)C(CH4)) might be of great help for this purpose. In this study we have tested near-infrared laser optical spectrometry and compared it with conventional continuous-flow isotope ratio mass spectrometry (CF-IRMS) using several methane carbon isotope standards and a large number of biogas samples from batch anaerobic reactors. Results from measurements on these samples were used to determine and compare the precision of the two techniques and to quantify for systematic offsets. With pure standards analytical precision of measurements for delta(13)C(CH4) was found to be in the range of 0.33 and 0.48 per thousand, and 0.09 and 0.27 per thousand for the optical method and CF-IRMS, respectively. Biogas samples showed an average mean deviation of delta(13)C(CH4) of 0.38 per thousand and 0.08 per thousand for the optical method and CF-IRMS, respectively. Although the tested laser optical spectrometer showed a dependence of delta(13)C(CH4) on CH(4) mixing ratio in the range of 500 to 8000 ppm this could be easily corrected. After correction, the delta(13)C(CH4) values usually varied within 0.7 per thousand from those measured by conventional CF-IRMS and thus results from both methods agreed within the given analytical uncertainties. Although the precision of the conventional CF-IRMS is higher than the tested optical system, both instruments were well within the acceptable delta(13)C(CH4) precision required for biogas methane measurements. The advantages of the optical system are its simplicity of operation, speed of analysis, good precision, reduced costs in comparison to IRMS, and the potential for field applications.
DOI:10.5194/amtd-5-2821-2012URL

Recent commercially available laser spectroscopy systems enabled us to continuously and reliably measure the δD and δO of atmospheric water vapor. The use of this new technology is becoming popular because of its advantages over the conventional approach based on cold trap collection. These advantages include much higher temporal resolution/continuous monitoring and the ability to make direct measurements of both isotopes in the field. Here, we evaluate the accuracy and precision of the laser based water vapor isotope instrument through a comparison of measurements with those found using the conventional cold trap method. A commercially available water vapor isotope analyzer (WVIA) with the vaporization system of a liquid water standard (Water Vapor Isotope Standard Source, WVISS) from Los Gatos Research (LGR) Inc. was used for this study. We found that the WVIA instrument can provide accurate results if (1) correction is applied for time-dependent isotope drift, (2) normalization to the VSMOW/SLAP scale is implemented, and (3) the water vapor concentration dependence of the isotopic ratio is also corrected. In addition, since the isotopic value of water vapor generated by the WVISS is also dependent on the concentration of water vapor, this effect must be considered to determine the true water vapor concentration effect on the resulting isotope measurement. To test our calibration procedure, continuous water vapor isotope measurements using both a laser instrument and a cold trap system were carried out at the IAEA Isotope Hydrology Laboratory in Vienna from August to December 2011. The calibrated isotopic values measured using the WVIA agree well with those obtained via the cold trap method. The standard deviation of the isotopic difference between both methods is about 1.4‰ for δD and 0.28‰ for δO. This precision allowed us to obtain reliable values for d-excess. The day-to-day variation of d-excess measured by WVIA also agrees well with that found using the cold trap method. These results demonstrate that a coupled system, using commercially available WVIA and WVISS instruments can provide continuous and accurate isotope data, with results achieved similar to those obtained using the conventional method, but with drastically improved temporal resolution.
[本文引用: 2]
[本文引用: 2]
DOI:10.1080/10256011003661326URL [本文引用: 1]
DOI:10.5194/amt-5-1601-2012URL [本文引用: 1]
DOI:10.1002/rcm.6982URLPMID:25132300 [本文引用: 1]

In recent years, research and applications of the N2O site-specific nitrogen isotope composition have advanced, reflecting awareness of the contribution of N2O to the anthropogenic greenhouse effect, and leading to significant progress in instrument development. Further dissemination of N2O isotopomer analysis, however, is hampered by a lack of internationally agreed gaseous N2O reference materials and an uncertain compatibility of different laboratories and analytical techniques.In a first comparison approach, eleven laboratories were each provided with N2O at tropospheric mole fractions (target gas T) and two reference gases (REF1 and REF2). The laboratories analysed all gases, applying their specific analytical routines. Compatibility of laboratories was assessed based on N2O isotopocule data for T, REF1 and REF2. Results for T were then standardised using REF1 and REF2 to evaluate the potential of N2O reference materials for improving compatibility between laboratories.Compatibility between laboratories depended on the analytical technique: isotope ratio mass spectrometry (IRMS) results showed better compatibility for (15)N values, while the performance of laser spectroscopy was superior with respect to N2O site preference. This comparison, however, is restricted by the small number of participating laboratories applying laser spectroscopy. Offset and two-point calibration correction of the N2O isotopomer data significantly improved the consistency of position-dependent nitrogen isotope data while the effect on (15)N values was only minor.The study reveals that for future research on N2O isotopocules, standardisation against N2O reference material is essential to improve interlaboratory compatibility. For atmospheric monitoring activities, we suggest N2O in whole air as a unifying scale anchor.
DOI:10.5194/amt-9-3879-2016URL [本文引用: 4]
DOI:10.1016/j.scitotenv.2015.08.130URLPMID:26363727 [本文引用: 2]

Abstract The stable isotope composition of atmospheric CO2 can be used as a tracer in the study of urban carbon cycles, which are affected by anthropogenic and biogenic CO2 components. Continuous measurements of the mixing ratio and 0207(13)C of atmospheric CO2 were conducted in Beijing from Nov. 15, 2012 to Mar. 8, 2014 including two heating seasons and a vegetative season. Both 0207(13)C and the isotopic composition of source CO2 (0207(13)CS) were depleted in the heating seasons and enriched in the vegetative season. The diurnal variations in the CO2 mixing ratio and 0207(13)C contained two peaks in the heating season, which are due to the effects of morning rush hour traffic. Seasonal and diurnal patterns of the CO2 mixing ratio and 0207(13)C were affected by anthropogenic emissions and biogenic activity. Assuming that the primary CO2 sources at night (22:00-04:00) were coal and natural gas combustion during heating seasons I and II, an isotopic mass balance analysis indicated that coal combustion had average contributions of 83.8300±14.11% and 86.8400±12.27% and that natural gas had average contributions of 16.1700±14.11% and 13.1600±12.27%, respectively. The 0207(13)C of background CO2 in air was the main error source in the isotopic mass balance model. Both the mixing ratio and 0207(13)C of atmospheric CO2 had significant linear relationships with the air quality index (AQI) and can be used to indicate local air pollution conditions. Energy structure optimization, for example, reducing coal consumption, will improve the local air conditions in Beijing. Copyright 0008 2015 Elsevier B.V. All rights reserved.
DOI:10.5194/bg-9-2385-2012URL [本文引用: 2]
DOI:10.5194/acpd-8-9531-2008URL [本文引用: 6]

Tunable diode laser absorption spectrometry (TDLAS) is gaining in popularity for measuring the mole fraction [CO] and stable isotopic composition (δC) of carbon dioxide (CO) in air in studies of biosphere-atmosphere gas exchange. Here we present a detailed examination of the performance of a commercially-available TDLAS located in a high-altitude subalpine coniferous forest (the Niwot Ridge AmeriFlux site), providing the first multi-year analysis of TDLAS instrument performance for measuring COisotopes in the field. Air was sampled from five to nine vertical locations in and above the forest canopy every ten minutes for 2.4 years. A variety of methods were used to assess instrument performance. Measurement of two compressed air cylinders that were in place over the entire study establish the long-term field precision of 0.2 μmol molfor [CO] and 0.35‰ for δC, but after fixing several problems the isotope precision improved to 0.2permil (over the last several months). The TDLAS provided detail on variability of δC of atmospheric COthat was not represented in weekly flask samples, as well as information regarding the influence of large-scale (regional) seasonal cycle and local forest processes on [CO] and δC of CO. There were also clear growing season and winter differences in the relative contributions of photosynthesis and respiration on the [CO] and δC of forest air.
DOI:10.1111/j.1365-2486.2011.02454.xURL [本文引用: 1]

The terrestrial carbon cycle is influenced by environmental variability at scales ranging from diurnal to interannual. Here, we present 5-years of growing season (day 131–275) observations of the carbon isotope ratio of ecosystem respiration (δ13CR) from a semiarid woodland. This ecosystem has a large necromass component resulting from 97%Pinus edulis mortality in 2002, is dominated by drought-tolerant Juniperus monosperma trees, and experiences large variability in the timing and intensity of seasonal and synoptic water availability. Mean growing season δ13CR was remarkably invariant (6123.57±0.4‰), with the exception of particularly enriched δ13CR in 2006 following a winter with anomalously low snowfall. δ13CR was strongly coupled to climate during premonsoon periods (65May to June), including fast (≤2 days) responses to changes in crown-level stomatal conductance (Gc) and vapor pressure deficit (vpd) following rain pulses. In contrast, δ13CR was relatively decoupled from Gc and environmental drivers during monsoon and postmonsoon periods (July–August and September, respectively), exhibiting only infrequent couplings of δ13CR to vpd and soil water content (SWC) with longer lags (658 days) and variable response slopes (both positive and negative). Notably, δ13CR exhibited consistent dynamics after rainfall events, with depleted δ13CR occurring within 1 h, progressive hourly δ13CR enrichment over the remainder of the night, and net δ13CR depletions over the multiple nights postevent in monsoon and postmonsoon periods. Overall this ecosystem demonstrated strong dependence of δ13CR on precipitation, with an apparent dominance by the autotrophic δ13C signal in premonsoon periods when deep soil moisture is abundant and surface soil moisture is low, and weaker coupling during monsoonal periods consistent with increasing heterotrophic dominance when deep soil moisture has declined and surface moisture is variable.
DOI:10.1016/j.agrformet.2011.09.007URL [本文引用: 7]

Stable isotope measurements of the biosphere tmosphere CO 2 exchange can be used to constrain carbon budget estimates on different spatial and temporal scales. Eddy covariance (EC) measurements can provide a direct measure of the isotope flux ratio of CO 2 and thus indicate the ecosystem fingerprint on the isotope budget of the atmosphere. For the first time we report on EC flux measurements of stable CO 2 isotopologues over a forest canopy. We used a quantum cascade laser absorption spectrometer (QCLAS, Aerodyne Research Inc.) for the simultaneous measurement of 12CO 2, 13CO 2 and CO 18O isotopologue fluxes with a sampling rate of 10 Hz over the Lgeren mixed forest site in Switzerland during an intensive field campaign in 2008. The total CO 2 flux measured with the closed-path QCLAS matches well the half-hourly fluxes measured with a conventional open-path infrared gas analyzer ( R 2 = 0.988). The 18O isotope discrimination is strongly reduced after precipitation events, reflecting 18O isotope exchange of CO 2 with water in various water pools of the ecosystem. The 13CO 2/ 12CO 2 flux ratio is surprisingly constant on a diurnal timescale, but the substantial random noise in the half-hourly EC flux ratio puts a limit on resolving diurnal variations of only a few permil. Hence, we explore which component in our EC set-up is limiting the precision of the measured flux ratios. On the one hand we use data simulations to estimate the error contribution from the QCLAS instrument. On the other hand different EC data processing procedures are examined to quantify the uncertainty inherently associated with turbulent flux measurements and calculations. We show that the random error associated with EC data processing is of the same order of magnitude as the flux ratio uncertainty from the instrument precision. Therefore, a direct determination of ecosystem discrimination on hourly or diurnal time scales with the EC technique remains a challenge.
DOI:10.5194/amt-3-67-2010URL
DOI:10.5194/amt-6-1659-2013URL [本文引用: 4]
DOI:10.1016/j.infrared.2013.05.011URL [本文引用: 1]

A near-IR laser absorption spectrometer using a technique of wavelength modulation spectroscopy is used to measure stable carbon isotope ratios of ambient CO 2 (δ 13 C) via the absorption lines 12 CO 2 R(17) (2 ν 1 + ν 1 2 61 ν 1 2 + ν 3 ) at 4978.205cm 611 and 13 CO 2 P(16) ( ν 1 +2 ν 2 + ν 3 ) at 4978.023cm 611 . The isotope ratios are measured with a reproducibility of 0.02‰ (1 σ ) in a 130-s integration time over a 12-h period. The humidity effect on δ 13 C values has been evaluated in laboratory experiments. The δ 13 C values of CO 2 in ambient air were measured continuously over 8days and agreed well with those from isotope ratio mass spectrometry of canister samples. The spectrometer is thus capable of real-time, in situ measurements of stable carbon isotope ratios of CO 2 under ambient conditions.
[本文引用: 7]
DOI:10.5194/amt-6-301-2013URL [本文引用: 6]

With the emergence of wide-spread application of new optical techniques to monitor δC in atmospheric COthere is a growing need to ensure well-calibrated measurements. We characterized one commonly available instrument, a cavity ring-down spectrometer (CRDS) system used for continuous in situ monitoring of atmospheric CO. We found no dependency of δC on the COconcentration in the range of 303-437 ppm. We designed a calibration scheme according to the diagnosed instrumental drifts and established a quality assurance protocol. We find that the repeatability (1-σ) of measurements is 0.25‰ for 10 min and 0.15‰ for 20 min integrated averages, respectively. Due to a spectral overlap, our instrument displays a cross-sensitivity to CHof 0.42 ± 0.024‰ ppm. Our ongoing target measurements yield standard deviations of δC from 0.22‰ to 0.28‰ for 10 min averages. We furthermore estimate the reproducibility of our system for ambient air samples from weekly measurements of a long-term target gas to be 0.18‰. We find only a minuscule offset of 0.002 ± 0.025‰ between the CRDS and Environment Canada's isotope ratio mass spectrometer (IRMS) results for four target gases used over the course of one year.
DOI:10.1016/j.atmosenv.2010.10.015URL [本文引用: 1]

We report the application of Mid-IR laser spectroscopy to differentiation of CO 2 sources. High-frequency isotopic CO 2 measurements (13 C and 18 O) in urban air in Nagoya allow for the contributions of anthropogenic and biogenic CO 2 sources to be differentiated. The laser spectroscopic method, using a Mid-IR quantum cascade laser, provides us with the advantage of higher temporal resolution of the data compared to traditional isotope ratio mass spectroscopy. The relative contribution of CO 2 sources in Nagoya was found to be roughly 0.42, 0.33 and 0.25 for natural combustion, gasoline combustion and biogenic respiration in the spring, but with large short-term (hourly) variations dependent on wind direction, and long-term variations dependent on heating demand and biological seasonality.
DOI:10.1016/j.ijms.2017.08.012URL [本文引用: 1]

Continuous-Flow Isotope Ratio Mass Spectrometry (CF-IRMS) coupled with a GasBench II was designed to rapidly analyze δ 13 C in carbonate or CO 2 in air. The dependence of measured δ 13 C ( δ 13 C m ) on signal intensity (peak area or peak height) was the main error source resulting from blank effect of samples and/or instrument itself effect. To determine the reasons and origins of signal intensity dependence, eight signal intensity gradients for two carbonate ( δ 13 C values were 6149.64 and 1.61‰, respectively) and a gas mixture of 0.3% CO 2 in He ( δ 13 C02=026119.32‰) standards were made respectively. The results showed that there was obvious signal intensity dependence, in particular when sample amount (peak area) was smaller. Given that the dependence was caused by blank effect and/or instrument nonlinear effect, without considering the blank effect, the signal intensity dependence could not be eliminated directly by the instrument nonlinear correction (empirical equation) methods However, it could be eliminated by blank correction (indirect subtraction method). In practice, blank effect should be corrected first, and then the instrument nonlinear effect.
DOI:10.1038/nature17966URLPMID:27357794 [本文引用: 1]

Abstract Terrestrial ecosystems currently offset one-quarter of anthropogenic carbon dioxide (CO2) emissions because of a slight imbalance between global terrestrial photosynthesis and respiration. Understanding what controls these two biological fluxes is therefore crucial to predicting climate change. Yet there is no way of directly measuring the photosynthesis or daytime respiration of a whole ecosystem of interacting organisms; instead, these fluxes are generally inferred from measurements of net ecosystem-atmosphere CO2 exchange (NEE), in a way that is based on assumed ecosystem-scale responses to the environment. The consequent view of temperate deciduous forests (an important CO2 sink) is that, first, ecosystem respiration is greater during the day than at night; and second, ecosystem photosynthetic light-use efficiency peaks after leaf expansion in spring and then declines, presumably because of leaf ageing or water stress. This view has underlain the development of terrestrial biosphere models used in climate prediction and of remote sensing indices of global biosphere productivity. Here, we use new isotopic instrumentation to determine ecosystem photosynthesis and daytime respiration in a temperate deciduous forest over a three-year period. We find that ecosystem respiration is lower during the day than at night-the first robust evidence of the inhibition of leaf respiration by light at the ecosystem scale. Because they do not capture this effect, standard approaches overestimate ecosystem photosynthesis and daytime respiration in the first half of the growing season at our site, and inaccurately portray ecosystem photosynthetic light-use efficiency. These findings revise our understanding of forest-atmosphere carbon exchange, and provide a basis for investigating how leaf-level physiological dynamics manifest at the canopy scale in other ecosystems.
DOI:10.1016/j.agrformet.2013.07.002URL [本文引用: 2]

Here we present a full growing season of direct EC measurements of the isotopic composition of NEE in a temperate deciduous forest, and we use these data: (1) to rigorously assess their limiting sources of error, (2) to test the indirect EC/flask method, and (3) to give an indication of the potential for ecological analyses. We describe the method and instrumentation, including the new cryogen-free, continuous-wave, quantum cascade laser spectrometer, which can determine δ 13 C and δ 18 O in ambient CO 2 with unprecedented noise levels of ±0.02‰ and ±0.03‰ (1 standard deviation), respectively, for a 100s integration time and a 1-h calibration interval. We find: (1) that precision is jointly limited by the instrumentation and by horizontal ecosystem or landscape heterogeneity, so that there is little to be gained by further improvements to instrument performance; (2) that the isotopic composition of NEE obtained by the EC/flask method can be biased, on a monthly timescale, by 2‰; and (3) that the present measurements are precise enough to elucidate biological mechanisms controlling the ecosystem-scale carbon balance.
DOI:10.1175/JTECH-D-10-05037.1URL [本文引用: 1]
DOI:10.5194/amt-6-1491-2013URL [本文引用: 11]

Isotope ratio infrared spectroscopy (IRIS) provides an in situ technique for measuring delta C-13 in atmospheric CO2. A number of methods have been proposed for calibrating the IRIS measurements, but few studies have systematically evaluated their accuracy for atmospheric applications. In this study, we carried out laboratory and ambient measurements with two commercial IRIS analyzers and compared the accuracy of four calibration strategies. We found that calibration based on the C-12 and C-13 mixing ratios (Bowling et al., 2003) and on linear interpolation of the measured delta using the mixing ratio of the major isotopologue (Lee et al., 2005) yielded accuracy better than 0.06 %. Over a 7-day atmospheric measurement in Beijing, the two analyzers agreed to within -0.02 +/- 0.18% after proper calibration. However, even after calibration the difference between the two analyzers showed a slight correlation with concentration, and this concentration dependence propagated through the Keeling analysis, resulting in a much larger difference of 2.44% for the Keeling intercept. The high sensitivity of the Keeling analysis to the concentration dependence underscores the challenge of IRIS for atmospheric research.
DOI:10.1016/j.jhydrol.2007.11.021URL [本文引用: 2]

The D/H and 18 O/ 16 O ratios in atmospheric water vapor provide rich information on the hydrological cycle and gaseous exchange processes between the terrestrial vegetation and the atmosphere. In this paper, we have demonstrated the feasibility to simultaneously measure both D/H and 18 O/ 16 O in atmospheric water vapor using a tunable diode laser absorption spectrometer. Our laboratory tests showed that the 1-h precision (one standard deviation) was 1.1‰ for D/H and 0.07‰ for 18 O/ 16 O at the dewpoint temperature of 1502°C. The difference between the laser measurement and cold trap/mass spectrometer analysis was 1.202±026.0‰ (hourly mean02±02one standard deviation) for D/H and 1.102±021.3‰ for 18 O/ 16 O. Our atmospheric measurement captured the rapidly changing isotopic signals in both D/H and 18 O/ 16 O. The measured isotope ratios were highly correlated with the water vapor mixing ratio as expected and followed very closely the Global Meteoric Water Line except during two transitional periods when the deuterium excess of atmospheric vapor deviated from the standard value. In addition, we have refined a method to provide independent, timely performance test of the in situ system.
DOI:10.1111/j.1469-8137.2010.03384.xURLPMID:20663061 [本文引用: 1]

090004Photosynthetic carbon (C) isotope discrimination (02000207) labels photosynthates (0207A) and atmospheric CO2 (0207a) with variable C isotope compositions during fluctuating environmental conditions. In this context, the C isotope composition of respired CO2 within ecosystems is often hypothesized to vary temporally with 02000207.090004We investigated the relationship between 02000207 and the C isotope signals from stem (0207W), soil (0207S) and ecosystem (0207E) respired CO2 to environmental fluctuations, using novel tuneable diode laser absorption spectrometer instrumentation in a mature maritime pine forest.090004Broad seasonal changes in 02000207 were reflected in 0207W,0207S and 0207E. However, respired CO2 signals had smaller short-term variations than 0200A and were offset and delayed by 209000910 d, indicating fractionation and isotopic mixing in a large C pool. Variations in 0207S did not follow 0200A at all times, especially during rainy periods and when there is a strong demand for C allocation above ground.090004It is likely that future isotope-enabled vegetation models will need to develop transfer functions that can account for these phenomena in order to interpret and predict the isotopic impact of biosphere gas exchange on the C isotope composition of atmospheric CO2.
DOI:10.1007/s004420051016URLPMID:28308584 [本文引用: 1]

Stable isotopes are a powerful research tool in environmental sciences and their use in ecosystem research is increasing. In this review we introduce and discuss the relevant details underlying the use of carbon and oxygen isotopic compositions in ecosystem gas exchange research. The current use and potential developments of stable isotope measurements together with concentration and flux measurements of CO60 and water vapor are emphasized. For these applications it is critical to know the isotopic identity of specific ecosystem components such as the isotopic composition of CO60, organic matter, liquid water, and water vapor, as well as the associated isotopic fractionations, in the soil-plant-atmosphere system. Combining stable isotopes and concentration measurements is very effective through the use of "Keeling plots." This approach allows the identification of the isotopic composition and the contribution of ecosystem, or ecosystem components, to the exchange fluxes with the atmosphere. It also allows the estimation of net ecosystem discrimination and soil disequilibrium effects. Recent modifications of the Keeling plot approach permit examination of CO60 recycling in ecosystems. Combining stable isotopes with dynamic flux measurements requires precision in isotopic sampling and analysis, which is currently at the limit of detection. Combined with the micrometeorological gradient approach (applicable mostly in grasslands and crop fields), stable isotope measurements allow separation of net CO60 exchange into photosynthetic and soil respiration components, and the evapotranspiration flux into soil evaporation and leaf transpiration. Similar applications in conjunction with eddy correlation techniques (applicable to forests, in addition to grasslands and crop fields) are more demanding, but can potentially be applied in combination with the Keeling plot relationship. The advance and potential in using stable isotope measurements should make their use a standard component in the limited arsenal of ecosystem-scale research tools.
DOI:10.1002/rcm.6916URLPMID:24861593 [本文引用: 1]

RATIONALEThe difference between the 15N natural abundance of 14N-15N-O and 15N-14N-O (site preference; SP) is used to understand the mechanisms underlying N2O emissions from soils. We investigated the use of quantum cascade laser (QCL) absorption spectrometry for continuous and precise analysis of the SP of N2O emitted from a field soil at atmospheric mixing ratios.METHODSA QCL-based spectrometer was used to determine the SP of soil-emitted N2O accumulated in a closed chamber system without preconcentration. N2O standards (<2500 ppbv) were used to evaluate the precision of the QCL spectrometry (QCLS) system. CO2 and H2O were removed from the gas samples. Intercomparison measurements of QCLS and isotope ratio mass spectrometry (IRMS) were performed on N2O calibration gases at different mixing ratios. The observed dependency of the QCLS result on the N2O mixing ratio was corrected.RESULTSMeasurement of SP of N2O emitted from the field suggested that the SP of N2O varied from 0 to 40‰ over a period of 165month. The precisions of the SP measurements (300–2500 ppbv) were <1.9‰ for δ15N values, <2.6‰ for δ15Nβ values, <2.1‰ for δ15Nbulk values, and <2.1‰ for the SP (165min averaging time) obtained on a once-an-hour calibrated QCLS system, with a cell temperature control precision of ±0.0165K.CONCLUSIONSContinuous and unattended measurements of the SP of N2O emitted from soils were achieved at low N2O mixing ratios. The accuracy of the QCLS measurements for the SP of N2O was significantly improved by precisely controlling the temperature of the system and by correcting for the concentration dependency of the raw data through an intercomparison with IRMS measurements. Copyright 08 2014 John Wiley & Sons, Ltd.
DOI:10.1111/j.1365-3040.2005.01425.xURLPMID:17080601 [本文引用: 1]

Ecosystem-scale estimation of photosynthesis and respiration using micrometeorological techniques remains an important, yet difficult, challenge. In this study, we combined micrometeorological and stable isotope methods to partition net ecosystem CO 2 exchange ( F N ) into photosynthesis ( F A ) and respiration ( F R ) in a corn–soybean rotation ecosystem during the summer 2003 corn phase. Mixing ratios of 12 CO 2 and 13 CO 2 were measured continuously using tunable diode laser (TDL) absorption spectroscopy. The dynamics of the isotope ratio of ecosystem respiration ( δ R ), net ecosystem CO 2 exchange ( δ N ) and photosynthetic discrimination at the canopy scale (Δ canopy ) were examined. During the period of full canopy closure, F N was partitioned into photosynthesis and respiration using both the isotopic approach and the conventional night-time-derived regression methodology. Results showed that δ R had significant seasonal variation (6132 to 6111‰) corresponding closely with canopy phenology. Daytime δ N typically varied from 6112 to 614‰, while Δ canopy remained relatively constant in the vicinity of 3‰. Compared with the regression approach, the isotopic flux partitioning showed more short-term variations and was considerably more symmetric about F N . In this experiment, the isotopic partitioning resulted in larger uncertainties, most of which were caused by the uncertainties in δ N and the daytime estimate of δ R . By sufficiently reducing these uncertainties, the tunable diode laser (TDL)–micrometeorological technique should yield a better understanding of the processes controlling photosynthesis, respiration and ecosystem-scale discrimination.
Measuring variations of 18O and δD in atmospheric water vapour using two commercial laser-based spectrometers: An instrument characterisation study.
2
2012
... 通过设置不同的仪器校正频率可以有效地校正仪器的时间漂移.校正频率是综合权衡仪器时间漂移、最大校正精度和最小校正时间消耗后的最优校正间隔(
... 随着IRIS技术的发展, 除了CO2外, 也可以实现H2O、CH4和N2O等多种同位素气体的原位连续测量, 在这些测量中同样需要考虑仪器的浓度依赖性和时间漂移等误差来源, 并通过适当的校正策略获得精度和准确度均满足研究需要的测量结果(
Influence of diurnal variation in mesophyll conductance on modelled 13C discrimination: Results from a field study
1
2010
... IRIS技术突破了传统大气CO2气瓶采样-稳定同位素质谱(IRMS)技术的分辨率低且费时耗力的局限性, 能够实现CO2碳氧同位素比值的原位连续观测(
Extensive observations of CO2 carbon isotope content in and above a high-elevation subalpine forest
6
2005
... IRIS技术突破了传统大气CO2气瓶采样-稳定同位素质谱(IRMS)技术的分辨率低且费时耗力的局限性, 能够实现CO2碳氧同位素比值的原位连续观测(
... O的变异特征, 并准确地追溯到国际标准(
... 采用适当的浓度依赖性和时间漂移的校正方法是提升仪器的测量精度, 并获得可追溯到国际标准的准确测量结果的重要保证.为了确保所有IRIS仪器都能高精度地测定自然条件下δ值的变异特征, 并准确地溯源到国际标准, 需要对IRIS仪器的测量结果进行适当的校正, 以消除浓度依赖性和时间漂移带来的误差(
... 前面阐述的浓度依赖性的理论校正和经验校正均指某个时刻的浓度依赖性校正而未涉及时间漂移问题.通常可以直接设置固定的校正频率, 定期测量CO2标准气体进行浓度依赖性的理论或经验动态校正, 这样在浓度依赖性校正的同时也对仪器的时间漂移进行了校正(
... 在前面阐述的浓度依赖性和时间漂移的校正中, 如果采用的已知CO2浓度和δ13C (或δ18O)值的标准气体已经溯源国际标准, 则在浓度依赖性和时间漂移校正的同时就实现了数据溯源(
... 由于IRIS仪器直接测量每个核素的浓度, 单个核素浓度值的测定误差导致了IRIS仪器的浓度依赖性(
Tunable diode laser absorption spectroscopy for stable isotope studies of ecosystem-atmosphere CO2 exchange
10
2003
... 稳定同位素观测仪器和方法的进步使稳定同位素技术成为解决生态、大气和水文等研究热点与难点问题的重要工具(
... IRIS技术突破了传统大气CO2气瓶采样-稳定同位素质谱(IRMS)技术的分辨率低且费时耗力的局限性, 能够实现CO2碳氧同位素比值的原位连续观测(
... 采用适当的浓度依赖性和时间漂移的校正方法是提升仪器的测量精度, 并获得可追溯到国际标准的准确测量结果的重要保证.为了确保所有IRIS仪器都能高精度地测定自然条件下δ值的变异特征, 并准确地溯源到国际标准, 需要对IRIS仪器的测量结果进行适当的校正, 以消除浓度依赖性和时间漂移带来的误差(
... 理想的校正方法是利用不同δ值的CO2标准气体或固体物质产生多个浓度梯度系列涵盖待测气体CO2浓度和δ值的变化范围, 可有效校正仪器的浓度依赖性.通常CO2标准气体浓度涵盖待测气体CO2浓度的范围比其涵盖δ值的范围更为重要(
... 少量研究只利用2个CO2 δ13C (或δ18O)标准气体进行浓度依赖性的理论校正, 由于缺乏独立的质控气体, 无法对数据的校正质量进行评价.例如,
... 大部分研究利用3个或者3个以上CO2 δ13C (或δ18O)标准气体进行相互检验, 以探讨不同CO2 δ13C (或δ18O)标准气体校正结果的准确性.例如: Wen等(2013)利用基于WS-CRDS技术的Picarro G1101-i和基于OA-ICOS技术的Los Gatos DLT-100在北京进行城市大气CO2 δ13C测量, 采用3个CO2在361.25- 436.41 μmol·mol-1和δ13C在-10.134 - -8.652‰的标准气体进行浓度依赖性的理论校正, 每个标准气体测量10 min, 每个测量循环30 min.利用每个测量循环的3个标准气体进行互相校正并评价校正结果的准确性, 校正后数据的准确性均优于0.03‰.Pang等(2016a)利用基于WS-CRDS技术的Picarro G1101-i在北京进行城市大气CO2 δ13C测量, 采用CO2为368.1、451.7和550.1 μmol·mol-1, δ13C均为-20.38‰的3个标准气体进行浓度依赖性的理论校正, 每个标准气体测量40 min, 每个测量循环120 min, 同样每个测量循环的3个标准气体进行互相校正并评价校正结果的准确性, 校正后G1101-i和G2201-i的准确性分别为-0.04‰-0.09‰和-0.13‰-0.03‰.需要注意的是, 虽然理论上要求标准气体同位素组成涵盖或接近待测样品气体同位素组成, 但实践中在浓度依赖性的理论校正中标准气体同位素组成是否有差异并涵盖或接近待测样品气体同位素组成不是最重要的, 需要至少保证标准气体CO2浓度能够涵盖样品气体的CO2浓度变化范围.标准气体CO2浓度范围涵盖样品气体CO2浓度时, 校正的准确性更高(
... 通过设置不同的仪器校正频率可以有效地校正仪器的时间漂移.校正频率是综合权衡仪器时间漂移、最大校正精度和最小校正时间消耗后的最优校正间隔(
... 前面阐述的浓度依赖性的理论校正和经验校正均指某个时刻的浓度依赖性校正而未涉及时间漂移问题.通常可以直接设置固定的校正频率, 定期测量CO2标准气体进行浓度依赖性的理论或经验动态校正, 这样在浓度依赖性校正的同时也对仪器的时间漂移进行了校正(
... 在前面阐述的浓度依赖性和时间漂移的校正中, 如果采用的已知CO2浓度和δ13C (或δ18O)值的标准气体已经溯源国际标准, 则在浓度依赖性和时间漂移校正的同时就实现了数据溯源(
... 由于IRIS仪器直接测量每个核素的浓度, 单个核素浓度值的测定误差导致了IRIS仪器的浓度依赖性(
Inter-comparison among three models for the δ 13C of respiration with four regression approaches.
1
2017
... 稳定同位素观测仪器和方法的进步使稳定同位素技术成为解决生态、大气和水文等研究热点与难点问题的重要工具(
Inter-comparison of two high-accuracy fast-response spectroscopic sensors of carbon dioxide: A case study
1
2012
... 由于IRIS仪器直接测量每个核素的浓度, 单个核素浓度值的测定误差导致了IRIS仪器的浓度依赖性(
Tracing the flow of carbon dioxide and water vapor between the biosphere and atmosphere: A review of optical isotope techniques and their application
7
2013
... 稳定同位素观测仪器和方法的进步使稳定同位素技术成为解决生态、大气和水文等研究热点与难点问题的重要工具(
... ).商业化的仪器包括调制式半导体激光吸收光谱(TDLAS)、波长扫描光腔衰荡光谱(WS-CRDS)、离轴综合腔输出光谱(OA-ICOS)、量子级联激光吸收光谱(QCLAS)和差频激光光谱(DFG)等(
... IRIS技术突破了传统大气CO2气瓶采样-稳定同位素质谱(IRMS)技术的分辨率低且费时耗力的局限性, 能够实现CO2碳氧同位素比值的原位连续观测(
... 采用适当的浓度依赖性和时间漂移的校正方法是提升仪器的测量精度, 并获得可追溯到国际标准的准确测量结果的重要保证.为了确保所有IRIS仪器都能高精度地测定自然条件下δ值的变异特征, 并准确地溯源到国际标准, 需要对IRIS仪器的测量结果进行适当的校正, 以消除浓度依赖性和时间漂移带来的误差(
... 由于IRIS仪器直接测量每个核素的浓度, 单个核素浓度值的测定误差导致了IRIS仪器的浓度依赖性(
... ;
... 相结合可以实现CO2碳氧同位素比值和通量的原位连续观测(
Measuring field-scale isotopic CO2 fluxes with tunable diode laser absorption spectroscopy and micrometeorological techniques
1
2004
... 相结合可以实现CO2碳氧同位素比值和通量的原位连续观测(
Feasibility of quantifying ecosystem-atmosphere C 18O 16O exchange using laser spectroscopy and the flux-gradient method
6
2005
... 理想的校正方法是利用不同δ值的CO2标准气体或固体物质产生多个浓度梯度系列涵盖待测气体CO2浓度和δ值的变化范围, 可有效校正仪器的浓度依赖性.通常CO2标准气体浓度涵盖待测气体CO2浓度的范围比其涵盖δ值的范围更为重要(
... 部分相关研究中也专门设置了CO2 δ13C (或δ18O)质控标准气体, 用于评价校正结果的准确性.例如:
... 通过设置不同的仪器校正频率可以有效地校正仪器的时间漂移.校正频率是综合权衡仪器时间漂移、最大校正精度和最小校正时间消耗后的最优校正间隔(
... 前面阐述的浓度依赖性的理论校正和经验校正均指某个时刻的浓度依赖性校正而未涉及时间漂移问题.通常可以直接设置固定的校正频率, 定期测量CO2标准气体进行浓度依赖性的理论或经验动态校正, 这样在浓度依赖性校正的同时也对仪器的时间漂移进行了校正(
... 在前面阐述的浓度依赖性和时间漂移的校正中, 如果采用的已知CO2浓度和δ13C (或δ18O)值的标准气体已经溯源国际标准, 则在浓度依赖性和时间漂移校正的同时就实现了数据溯源(
... 相结合可以实现CO2碳氧同位素比值和通量的原位连续观测(
A fourier transform infrared trace gas and isotope analyser for atmospheric applications
4
2012
... IRIS技术突破了传统大气CO2气瓶采样-稳定同位素质谱(IRMS)技术的分辨率低且费时耗力的局限性, 能够实现CO2碳氧同位素比值的原位连续观测(
... 浓度依赖性是指IRIS仪器测量具有相同δ13C (或δ18O)的不同浓度的CO2标准气体时表现出的δ测量值与CO2浓度的非线性关系(
... 由于IRIS仪器直接测量每个核素的浓度, 单个核素浓度值的测定误差导致了IRIS仪器的浓度依赖性(
... ;
Monitoring CO2 concentration and 13C in an underground cavity using a commercial isotope ratio infrared spectrometer.
5
2015
... IRIS技术突破了传统大气CO2气瓶采样-稳定同位素质谱(IRMS)技术的分辨率低且费时耗力的局限性, 能够实现CO2碳氧同位素比值的原位连续观测(
... 采用适当的浓度依赖性和时间漂移的校正方法是提升仪器的测量精度, 并获得可追溯到国际标准的准确测量结果的重要保证.为了确保所有IRIS仪器都能高精度地测定自然条件下δ值的变异特征, 并准确地溯源到国际标准, 需要对IRIS仪器的测量结果进行适当的校正, 以消除浓度依赖性和时间漂移带来的误差(
... 时间漂移是指IRIS仪器持续测量具有相同δ13C (或δ18O)的同一浓度的CO2标准气体时表现出的δ测量值随时间的变化特征.仪器运行过程中, 激光频率和强度的改变、腔室内气体温度和压力波动以及激光老化等仪器自身原因都会造成δ测量值的时间漂移(
... 气体进行干燥, 以保持样品的水汽浓度维持在较低的水平(
... 前面阐述的浓度依赖性的理论校正和经验校正均指某个时刻的浓度依赖性校正而未涉及时间漂移问题.通常可以直接设置固定的校正频率, 定期测量CO2标准气体进行浓度依赖性的理论或经验动态校正, 这样在浓度依赖性校正的同时也对仪器的时间漂移进行了校正(
Using a laser-based CO2 carbon isotope analyser to investigate gas transfer in geological media
7
2012
... IRIS技术突破了传统大气CO2气瓶采样-稳定同位素质谱(IRMS)技术的分辨率低且费时耗力的局限性, 能够实现CO2碳氧同位素比值的原位连续观测(
... 采用适当的浓度依赖性和时间漂移的校正方法是提升仪器的测量精度, 并获得可追溯到国际标准的准确测量结果的重要保证.为了确保所有IRIS仪器都能高精度地测定自然条件下δ值的变异特征, 并准确地溯源到国际标准, 需要对IRIS仪器的测量结果进行适当的校正, 以消除浓度依赖性和时间漂移带来的误差(
... 通常, IRIS仪器校正分为内部校正和外部校正.内部校正是指试验前由仪器制造商或者仪器使用者通过直接改变分析仪本身参数设置而达到校正目的(
... 在无法准确获得标准气体12CO2和13CO2或C16O2和C18O16O浓度真值而已知δ13C或δ18O真值的条件下, 可以考虑通过直接建立δ13C或δ18O测量值与CO2测定值关系的多项式拟合经验方程, 对浓度依赖性进行校正(
... 通常建立上述多项式经验拟合方程后, 可以直接利用该方程和δ13C或δ18O真值直接进行经验浓度依赖性校正, 或利用该函数关系将所有测定数据标准化到某CO2浓度(如400 μmol·mol-1)进行经验浓度依赖性校正, 然后再建立该CO2浓度下CO2 δ13C (或δ18O)标准气体的测量值与真值的单点、两点或多点函数关系并进行δ值校正(
... 以往研究已经开展对IRIS仪器的稳定性和精度的各种测试研究, 基于这些仪器稳定性和精度的测试结果可以确定适当的时间漂移校正频率, 以获得符合试验精度需求的校正结果.例如,
... 由于IRIS仪器直接测量每个核素的浓度, 单个核素浓度值的测定误差导致了IRIS仪器的浓度依赖性(
Assessment of a multi-species in situ ftir for precise atmospheric greenhouse gas observations.
1
2013
... 由于IRIS仪器直接测量每个核素的浓度, 单个核素浓度值的测定误差导致了IRIS仪器的浓度依赖性(
Measurements of 13C/ 12C methane from anaerobic digesters: Comparison of optical spectrometry with continuous-flow isotope ratio mass spectrometry
1
2010
... 随着IRIS技术的发展, 除了CO2外, 也可以实现H2O、CH4和N2O等多种同位素气体的原位连续测量, 在这些测量中同样需要考虑仪器的浓度依赖性和时间漂移等误差来源, 并通过适当的校正策略获得精度和准确度均满足研究需要的测量结果(
Evaluation of continuous water vapor δD and δ 18O measurements by off-axis integrated cavity output spectroscopy.
2012
2
2013
... 稳定同位素观测仪器和方法的进步使稳定同位素技术成为解决生态、大气和水文等研究热点与难点问题的重要工具(
... 稳定同位素是指不发生或极不易发生放射性衰变的具有相同质子数、不同中子数的同一元素的不同核素.稳定同位素组成可以用同位素丰度或原子百分比、绝对丰度、原子百分超或同位素比率表示(
2
2013
... 稳定同位素观测仪器和方法的进步使稳定同位素技术成为解决生态、大气和水文等研究热点与难点问题的重要工具(
... 稳定同位素是指不发生或极不易发生放射性衰变的具有相同质子数、不同中子数的同一元素的不同核素.稳定同位素组成可以用同位素丰度或原子百分比、绝对丰度、原子百分超或同位素比率表示(
Long-term continuous sampling of 12CO2, 13CO2 and 12C 18O 16O in ambient air with a quantum cascade laser spectrometer
1
2010
... 稳定同位素观测仪器和方法的进步使稳定同位素技术成为解决生态、大气和水文等研究热点与难点问题的重要工具(
Site selective real-time measurements of atmospheric N2O isotopomers by laser spectroscopy
1
2012
... 随着IRIS技术的发展, 除了CO2外, 也可以实现H2O、CH4和N2O等多种同位素气体的原位连续测量, 在这些测量中同样需要考虑仪器的浓度依赖性和时间漂移等误差来源, 并通过适当的校正策略获得精度和准确度均满足研究需要的测量结果(
Interlaboratory assessment of nitrous oxide isotopomer analysis by isotope ratio mass spectrometry and laser spectroscopy: Current status and perspectives
1
2014
... 随着IRIS技术的发展, 除了CO2外, 也可以实现H2O、CH4和N2O等多种同位素气体的原位连续测量, 在这些测量中同样需要考虑仪器的浓度依赖性和时间漂移等误差来源, 并通过适当的校正策略获得精度和准确度均满足研究需要的测量结果(
a). Intercomparison of two cavity ring-down spectroscopy analyzers for atmospheric 13CO2/ 12CO2 measurement
4
2016
... 根据上述方程, 即使浓度依赖性的响应为非线性的, 在较小的浓度范围内也可近似地认为δ值的浓度依赖性是线性的.例如,
... IRIS仪器对环境条件变化(如温度和压力等)的敏感性会导致仪器δ测量值的时间漂移, 即同一浓度和δ值的CO2标准气体在不同时间的测量结果会发生变化(
... IRMS是相对测量技术, 而IRIS是绝对测量技术.所有的IRMS和IRIS仪器测定的δ值均需要利用1个或者多个标准物质溯源到同位素国际标准, 这一过程称为数据溯源.IRIS技术实现了对空气CO2 δ13C和δ18O的连续测定, 但需要IRMS提供数据溯源至CO2碳或氧同位素国际标准(Vienna Pee Dee Belemnite)的CO2 δ13C和δ18O标准气体(
... 在前面阐述的浓度依赖性和时间漂移的校正中, 如果采用的已知CO2浓度和δ13C (或δ18O)值的标准气体已经溯源国际标准, 则在浓度依赖性和时间漂移校正的同时就实现了数据溯源(
b). Mixing ratio and carbon isotopic composition investigation of atmospheric CO2 in Beijing, China
2
2016
... 前面阐述的浓度依赖性的理论校正和经验校正均指某个时刻的浓度依赖性校正而未涉及时间漂移问题.通常可以直接设置固定的校正频率, 定期测量CO2标准气体进行浓度依赖性的理论或经验动态校正, 这样在浓度依赖性校正的同时也对仪器的时间漂移进行了校正(
... 在前面阐述的浓度依赖性和时间漂移的校正中, 如果采用的已知CO2浓度和δ13C (或δ18O)值的标准气体已经溯源国际标准, 则在浓度依赖性和时间漂移校正的同时就实现了数据溯源(
Use of the isotope flux ratio approach to investigate the 12C 18O 16O and 13CO2 exchange near the floor of a temperate deciduous forest
2
2012
... 其中, F12C和F13C(F16O和F18O)分别为轻重同位素的通量.同位素通量比率可以由涡度协方差或者通量廓线技术的进行观测和计算(
... 相结合可以实现CO2碳氧同位素比值和通量的原位连续观测(
Long-term field performance of a tunable diode laser absorption spectrometer for analysis of carbon isotopes of CO2 in forest air
6
2008
... IRIS技术突破了传统大气CO2气瓶采样-稳定同位素质谱(IRMS)技术的分辨率低且费时耗力的局限性, 能够实现CO2碳氧同位素比值的原位连续观测(
... ;
... 采用适当的浓度依赖性和时间漂移的校正方法是提升仪器的测量精度, 并获得可追溯到国际标准的准确测量结果的重要保证.为了确保所有IRIS仪器都能高精度地测定自然条件下δ值的变异特征, 并准确地溯源到国际标准, 需要对IRIS仪器的测量结果进行适当的校正, 以消除浓度依赖性和时间漂移带来的误差(
... IRIS仪器对环境条件变化(如温度和压力等)的敏感性会导致仪器δ测量值的时间漂移, 即同一浓度和δ值的CO2标准气体在不同时间的测量结果会发生变化(
... 前面阐述的浓度依赖性的理论校正和经验校正均指某个时刻的浓度依赖性校正而未涉及时间漂移问题.通常可以直接设置固定的校正频率, 定期测量CO2标准气体进行浓度依赖性的理论或经验动态校正, 这样在浓度依赖性校正的同时也对仪器的时间漂移进行了校正(
... 在前面阐述的浓度依赖性和时间漂移的校正中, 如果采用的已知CO2浓度和δ13C (或δ18O)值的标准气体已经溯源国际标准, 则在浓度依赖性和时间漂移校正的同时就实现了数据溯源(
The role of interannual, seasonal, and synoptic climate on the carbon isotope ratio of ecosystem respiration at a semiarid woodland
1
2011
... IRIS技术突破了传统大气CO2气瓶采样-稳定同位素质谱(IRMS)技术的分辨率低且费时耗力的局限性, 能够实现CO2碳氧同位素比值的原位连续观测(
Eddy covariance measurements of CO2 isotopologues with a quantum cascade laser absorption spectrometer
7
2012
... 其中, F12C和F13C(F16O和F18O)分别为轻重同位素的通量.同位素通量比率可以由涡度协方差或者通量廓线技术的进行观测和计算(
... 通常, IRIS仪器校正分为内部校正和外部校正.内部校正是指试验前由仪器制造商或者仪器使用者通过直接改变分析仪本身参数设置而达到校正目的(
... 在无法准确获得标准气体12CO2和13CO2或C16O2和C18O16O浓度真值而已知δ13C或δ18O真值的条件下, 可以考虑通过直接建立δ13C或δ18O测量值与CO2测定值关系的多项式拟合经验方程, 对浓度依赖性进行校正(
... 通常建立上述多项式经验拟合方程后, 可以直接利用该方程和δ13C或δ18O真值直接进行经验浓度依赖性校正, 或利用该函数关系将所有测定数据标准化到某CO2浓度(如400 μmol·mol-1)进行经验浓度依赖性校正, 然后再建立该CO2浓度下CO2 δ13C (或δ18O)标准气体的测量值与真值的单点、两点或多点函数关系并进行δ值校正(
... 前面阐述的浓度依赖性的理论校正和经验校正均指某个时刻的浓度依赖性校正而未涉及时间漂移问题.通常可以直接设置固定的校正频率, 定期测量CO2标准气体进行浓度依赖性的理论或经验动态校正, 这样在浓度依赖性校正的同时也对仪器的时间漂移进行了校正(
... 也有研究者单独设置CO2标准气体对仪器的时间漂移进行校正(
... 在前面阐述的浓度依赖性和时间漂移的校正中, 如果采用的已知CO2浓度和δ13C (或δ18O)值的标准气体已经溯源国际标准, 则在浓度依赖性和时间漂移校正的同时就实现了数据溯源(
Water vapor δ 2H and δ 18O measurements using off-axis integrated cavity output spectroscopy
2010
Tracking isotopic signatures of CO2 at the high altitude site jungfraujoch with laser spectroscopy: Analytical improvements and representative results
4
2013
... 时间漂移是指IRIS仪器持续测量具有相同δ13C (或δ18O)的同一浓度的CO2标准气体时表现出的δ测量值随时间的变化特征.仪器运行过程中, 激光频率和强度的改变、腔室内气体温度和压力波动以及激光老化等仪器自身原因都会造成δ测量值的时间漂移(
... 前面阐述的浓度依赖性的理论校正和经验校正均指某个时刻的浓度依赖性校正而未涉及时间漂移问题.通常可以直接设置固定的校正频率, 定期测量CO2标准气体进行浓度依赖性的理论或经验动态校正, 这样在浓度依赖性校正的同时也对仪器的时间漂移进行了校正(
... 也有研究者单独设置CO2标准气体对仪器的时间漂移进行校正(
... 在前面阐述的浓度依赖性和时间漂移的校正中, 如果采用的已知CO2浓度和δ13C (或δ18O)值的标准气体已经溯源国际标准, 则在浓度依赖性和时间漂移校正的同时就实现了数据溯源(
Continuous measurements of stable carbon isotopes in CO2 with a near-IR laser absorption spectrometer
1
2013
... 稳定同位素观测仪器和方法的进步使稳定同位素技术成为解决生态、大气和水文等研究热点与难点问题的重要工具(
High precision and continuous field measurements of δ 13C and δ 18O in carbon dioxide with a cryogen-free qclas.
7
2008
... 采用适当的浓度依赖性和时间漂移的校正方法是提升仪器的测量精度, 并获得可追溯到国际标准的准确测量结果的重要保证.为了确保所有IRIS仪器都能高精度地测定自然条件下δ值的变异特征, 并准确地溯源到国际标准, 需要对IRIS仪器的测量结果进行适当的校正, 以消除浓度依赖性和时间漂移带来的误差(
... 在无法准确获得标准气体12CO2和13CO2或C16O2和C18O16O浓度真值而已知δ13C或δ18O真值的条件下, 可以考虑通过直接建立δ13C或δ18O测量值与CO2测定值关系的多项式拟合经验方程, 对浓度依赖性进行校正(
... 通常建立上述多项式经验拟合方程后, 可以直接利用该方程和δ13C或δ18O真值直接进行经验浓度依赖性校正, 或利用该函数关系将所有测定数据标准化到某CO2浓度(如400 μmol·mol-1)进行经验浓度依赖性校正, 然后再建立该CO2浓度下CO2 δ13C (或δ18O)标准气体的测量值与真值的单点、两点或多点函数关系并进行δ值校正(
... 例如,
... 也有研究者单独设置CO2标准气体对仪器的时间漂移进行校正(
... 在前面阐述的浓度依赖性和时间漂移的校正中, 如果采用的已知CO2浓度和δ13C (或δ18O)值的标准气体已经溯源国际标准, 则在浓度依赖性和时间漂移校正的同时就实现了数据溯源(
... 由于IRIS仪器直接测量每个核素的浓度, 单个核素浓度值的测定误差导致了IRIS仪器的浓度依赖性(
Evaluation of a cavity ring-down spectrometer for in situ observations of 13CO2.
6
2013
... IRIS技术突破了传统大气CO2气瓶采样-稳定同位素质谱(IRMS)技术的分辨率低且费时耗力的局限性, 能够实现CO2碳氧同位素比值的原位连续观测(
... C浓度依赖性分别为1.75‰和0.47‰.仪器的长期漂移也是影响IRIS仪器测量结果准确性的重要因素(
... 采用适当的浓度依赖性和时间漂移的校正方法是提升仪器的测量精度, 并获得可追溯到国际标准的准确测量结果的重要保证.为了确保所有IRIS仪器都能高精度地测定自然条件下δ值的变异特征, 并准确地溯源到国际标准, 需要对IRIS仪器的测量结果进行适当的校正, 以消除浓度依赖性和时间漂移带来的误差(
... 通过设置不同的仪器校正频率可以有效地校正仪器的时间漂移.校正频率是综合权衡仪器时间漂移、最大校正精度和最小校正时间消耗后的最优校正间隔(
... ;
... 由于IRIS仪器直接测量每个核素的浓度, 单个核素浓度值的测定误差导致了IRIS仪器的浓度依赖性(
Observation of carbon and oxygen isotopic compositions of CO2 at an urban site in nagoya using mid-IR laser absorption spectroscopy
1
2011
... 在前面阐述的浓度依赖性和时间漂移的校正中, 如果采用的已知CO2浓度和δ13C (或δ18O)值的标准气体已经溯源国际标准, 则在浓度依赖性和时间漂移校正的同时就实现了数据溯源(
Differentiated correction on the signal intensity dependence of GasBench II-IRMS from blank effect and instrument nonlinear effect
1
2017
... IRMS是相对测量技术, 而IRIS是绝对测量技术.所有的IRMS和IRIS仪器测定的δ值均需要利用1个或者多个标准物质溯源到同位素国际标准, 这一过程称为数据溯源.IRIS技术实现了对空气CO2 δ13C和δ18O的连续测定, 但需要IRMS提供数据溯源至CO2碳或氧同位素国际标准(Vienna Pee Dee Belemnite)的CO2 δ13C和δ18O标准气体(
Seasonality of temperate forest photosynthesis and daytime respiration
1
2016
... 其中, F12C和F13C(F16O和F18O)分别为轻重同位素的通量.同位素通量比率可以由涡度协方差或者通量廓线技术的进行观测和计算(
Long-term eddy covariance measurements of the isotopic composition of the ecosystem- atmosphere exchange of CO2 in a temperate forest
2
2013
... 浓度依赖性是指IRIS仪器测量具有相同δ13C (或δ18O)的不同浓度的CO2标准气体时表现出的δ测量值与CO2浓度的非线性关系(
... 前面阐述的浓度依赖性的理论校正和经验校正均指某个时刻的浓度依赖性校正而未涉及时间漂移问题.通常可以直接设置固定的校正频率, 定期测量CO2标准气体进行浓度依赖性的理论或经验动态校正, 这样在浓度依赖性校正的同时也对仪器的时间漂移进行了校正(
Inter-comparison of four commercial analyzers for water vapor isotope measurement
1
2012
... 随着IRIS技术的发展, 除了CO2外, 也可以实现H2O、CH4和N2O等多种同位素气体的原位连续测量, 在这些测量中同样需要考虑仪器的浓度依赖性和时间漂移等误差来源, 并通过适当的校正策略获得精度和准确度均满足研究需要的测量结果(
Evaluating calibration strategies for isotope ratio infrared spectroscopy for atmospheric 13CO2/ 12CO2 measurement
11
2013
... 稳定同位素观测仪器和方法的进步使稳定同位素技术成为解决生态、大气和水文等研究热点与难点问题的重要工具(
... ;
... 通常, IRIS仪器校正分为内部校正和外部校正.内部校正是指试验前由仪器制造商或者仪器使用者通过直接改变分析仪本身参数设置而达到校正目的(
... 浓度依赖性是指IRIS仪器测量具有相同δ13C (或δ18O)的不同浓度的CO2标准气体时表现出的δ测量值与CO2浓度的非线性关系(
... 根据上述方程可知, 如果b12和b13不为0, 那么方程中的斜率和截距将会随着CO2浓度的变化而变化, 从而导致δ值对CO2浓度的依赖性, 并且在一定CO2浓度范围内表现出非线性响应.因此, 当仪器对12CO2和13CO2浓度的响应为非完全线性时, 也必然会导致δ值的浓度依赖性(
... 理想的校正方法是利用不同δ值的CO2标准气体或固体物质产生多个浓度梯度系列涵盖待测气体CO2浓度和δ值的变化范围, 可有效校正仪器的浓度依赖性.通常CO2标准气体浓度涵盖待测气体CO2浓度的范围比其涵盖δ值的范围更为重要(
... 大部分研究利用3个或者3个以上CO2 δ13C (或δ18O)标准气体进行相互检验, 以探讨不同CO2 δ13C (或δ18O)标准气体校正结果的准确性.例如: Wen等(2013)利用基于WS-CRDS技术的Picarro G1101-i和基于OA-ICOS技术的Los Gatos DLT-100在北京进行城市大气CO2 δ13C测量, 采用3个CO2在361.25- 436.41 μmol·mol-1和δ13C在-10.134 - -8.652‰的标准气体进行浓度依赖性的理论校正, 每个标准气体测量10 min, 每个测量循环30 min.利用每个测量循环的3个标准气体进行互相校正并评价校正结果的准确性, 校正后数据的准确性均优于0.03‰.Pang等(2016a)利用基于WS-CRDS技术的Picarro G1101-i在北京进行城市大气CO2 δ13C测量, 采用CO2为368.1、451.7和550.1 μmol·mol-1, δ13C均为-20.38‰的3个标准气体进行浓度依赖性的理论校正, 每个标准气体测量40 min, 每个测量循环120 min, 同样每个测量循环的3个标准气体进行互相校正并评价校正结果的准确性, 校正后G1101-i和G2201-i的准确性分别为-0.04‰-0.09‰和-0.13‰-0.03‰.需要注意的是, 虽然理论上要求标准气体同位素组成涵盖或接近待测样品气体同位素组成, 但实践中在浓度依赖性的理论校正中标准气体同位素组成是否有差异并涵盖或接近待测样品气体同位素组成不是最重要的, 需要至少保证标准气体CO2浓度能够涵盖样品气体的CO2浓度变化范围.标准气体CO2浓度范围涵盖样品气体CO2浓度时, 校正的准确性更高(
... IRMS是相对测量技术, 而IRIS是绝对测量技术.所有的IRMS和IRIS仪器测定的δ值均需要利用1个或者多个标准物质溯源到同位素国际标准, 这一过程称为数据溯源.IRIS技术实现了对空气CO2 δ13C和δ18O的连续测定, 但需要IRMS提供数据溯源至CO2碳或氧同位素国际标准(Vienna Pee Dee Belemnite)的CO2 δ13C和δ18O标准气体(
... 在前面阐述的浓度依赖性和时间漂移的校正中, 如果采用的已知CO2浓度和δ13C (或δ18O)值的标准气体已经溯源国际标准, 则在浓度依赖性和时间漂移校正的同时就实现了数据溯源(
... 由于IRIS仪器直接测量每个核素的浓度, 单个核素浓度值的测定误差导致了IRIS仪器的浓度依赖性(
... ;
Continuous measurement of water vapor D/H and 18O/ 16O isotope ratios in the atmosphere
2
2008
... 随着IRIS技术的发展, 除了CO2外, 也可以实现H2O、CH4和N2O等多种同位素气体的原位连续测量, 在这些测量中同样需要考虑仪器的浓度依赖性和时间漂移等误差来源, 并通过适当的校正策略获得精度和准确度均满足研究需要的测量结果(
... 存在明显的浓度依赖性.这些浓度依赖性可以通过理论校正或者经验方程校正予以消除(
Photosynthetic carbon isotope discrimination and its relationship to the carbon isotope signals of stem, soil and ecosystem respiration
1
2010
... IRIS技术突破了传统大气CO2气瓶采样-稳定同位素质谱(IRMS)技术的分辨率低且费时耗力的局限性, 能够实现CO2碳氧同位素比值的原位连续观测(
The use of stable isotopes to study ecosystem gas exchange
1
2000
... 稳定同位素观测仪器和方法的进步使稳定同位素技术成为解决生态、大气和水文等研究热点与难点问题的重要工具(
Continuous and unattended measurements of the site preference of nitrous oxide emitted from an agricultural soil using quantum cascade laser spectrometry with intercomparison with isotope ratio mass spectrometry
1
2014
... 随着IRIS技术的发展, 除了CO2外, 也可以实现H2O、CH4和N2O等多种同位素气体的原位连续测量, 在这些测量中同样需要考虑仪器的浓度依赖性和时间漂移等误差来源, 并通过适当的校正策略获得精度和准确度均满足研究需要的测量结果(
Using continuous stable isotope measurements to partition net ecosystem CO2 exchange
1
2006
... 相结合可以实现CO2碳氧同位素比值和通量的原位连续观测(
Copyright © 2021 版权所有 《植物生态学报》编辑部
地址: 北京香山南辛村20号, 邮编: 100093
Tel.: 010-62836134, 62836138; Fax: 010-82599431; E-mail: apes@ibcas.ac.cn, cjpe@ibcas.ac.cn
备案号: 京ICP备16067583号-19