HTML
--> --> -->Accurate retrieval of ozone columns, as well as comprehensive analysis of stratospheric chemistry, dynamics and temperature changes on ozone columns is necessary for the analysis of Antarctic ozone changes. Ozone columns over Antarctica are mainly obtained from satellite observations, ground-based DOAS observations, Brewer spectrophotometers, and Dobson spectrophotometers (?í?ková et al., 2019; Kokhanovsky et al., 2020). Satellite observations and European Centre for Medium-Range Weather Forecasts (ECMWF) data were analyzed to study the influence of stratospheric halogen species (mainly Cl and Br) in the polar vortex, which may lead to ozone depletion over Antarctica (Marsing et al., 2019; Nakajima et al., 2020). In addition, an atmospheric and chemical transport model is used for the analysis of long-term ozone trends and troposphere-stratosphere exchange in Antarctica (Hegglin and Shepherd, 2009; Lu et al., 2019).
The atmosphere over Antarctica is controlled by the strong polar vortex in winter, making it difficult to exchange with mid-latitude atmosphere. The extremely low air temperatures (< ?78°C) inside the polar vortex, lead to the formation of polar stratospheric clouds (PSCs). PSCs, composed of nitrate trihydrate, water ice, etc., provide surfaces for heterogeneous reactions that convert halogen reservoirs to active halogens causing severe ozone depletion (Frieβ et al., 2005; Drdla and Müller, 2012; Marsing et al., 2019). There are three types of PSCs (decided by their state), including nitric acid trihydrate (NAT), supercooled ternary solution (STS), and ice PSCs, and their corresponding temperatures are



As a spectroscopic technique, differential optical absorption spectroscopy (DOAS) has been proven to be powerful and has been widely used to monitor a variety of atmospheric trace gases (Stutz and Platt, 1997; Meller and Moortgat, 2000; Platt and Stutz, 2008). Zenith Scattered Light-DOAS (ZSL-DOAS) is suitable for measuring stratospheric gases, such as stratospheric


In this study, daily variations of ozone vertical column densities (VCDs) are retrieved by ZSL-DOAS, and a correlation analysis is performed between the ZSL-DOAS measurements and OMI, GOME-2 observations and Modern-Era Retrospective analysis for Research and Applications version 2 (MERRA-2). Combining observed ozone VCDs and low-stratospheric PV profiles, the cause of ozone depletion leading to ozone holes from September to October in 2017 and 2018 in the experiment region (62.22°S, 58.96°W) at the edge of the polar vortex is investigated. The aim of this study is to analyze the correlation between ozone depletion and PV at the edge of the polar vortex, where ozone depletion is more sensitive to changes in PV.
2.1. Experiment site
The experiment site and DOAS instrument are shown in Fig. 1. The red star is the location of Chinese Great Wall Station (62.22°S, 58.96°W) in Fildes Peninsula, South Shetland Islands. The red region is the area of the OMI pixel, and the yellow region is the area of the GOME-2 pixel.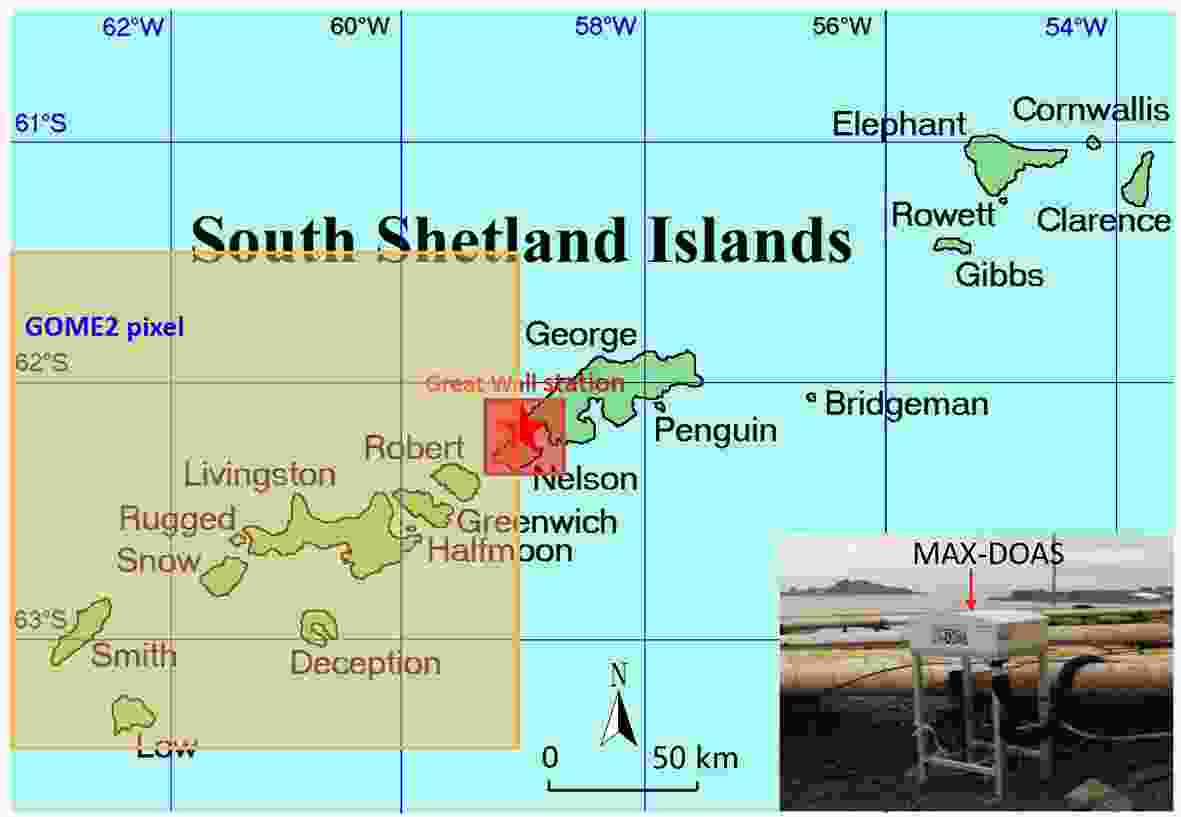
The ground-based passive DOAS system used in this experiment is composed of key parts such as a prism, telescope, motor, filter, CCD spectrometer, and computer. The wavelength range of the spectrometer is 290?420 nm, and the spectral resolution is 0.3 nm. In this experiment, the data from zenith direction is used to retrieve the slant column densities (SCDs) of ozone.
2
2.2. Principles of the DOAS method
The DOAS method retrieves concentrations of trace gases based on their characteristic absorption and the measured intensity, which is based on Lambert-Beer’s law. From Lambert-Beer’s law and derivation:here,







2
2.3. Spectral retrieval
The ozone SCDs are retrieved from the QDOAS software developed by the Royal Belgian Institute for Space Aeronomy (BIRA-IASB) (



Parameter | References |
$ {\rm{O}}_{3} $ | 223 K, 243 K (Bogumil et al., 2003) |
$ {\rm{NO}}_{2} $ | 298 K (VanDaele et al., 1996) |
$ {\rm{O}}_{4} $ | 293 K (Hermans et al., 2003) |
Ring | Ring.exe |
Fitting Interval | 320?340 nm |
Polynomial | 5 |
Table1. Fitting parameters of spectral retrieval.
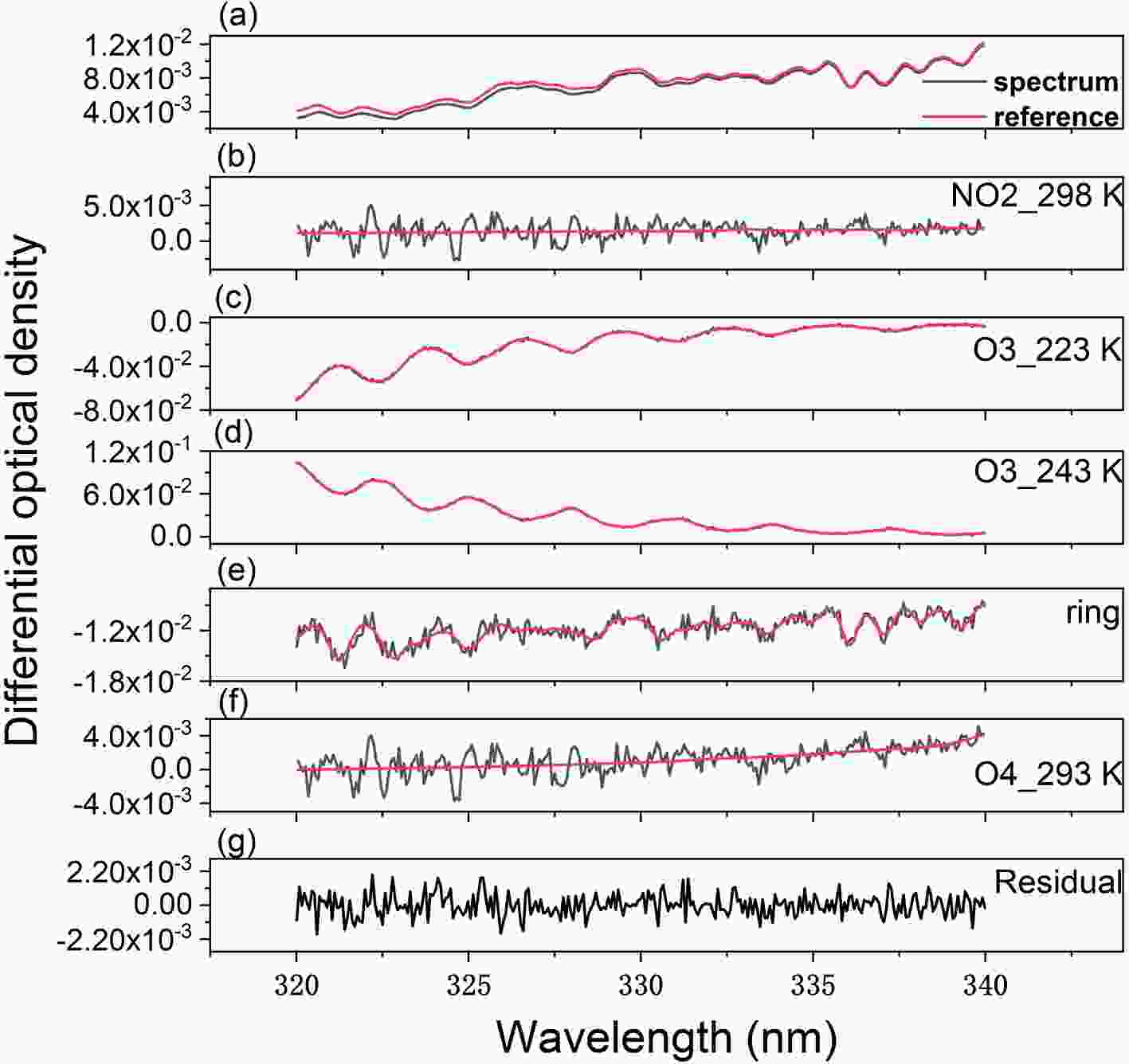
2
2.4. Calculation of ozone VCDs
The ZSL-DOAS method is powerful in measuring stratospheric gases such as ozone. To convert SCD (related to the viewing angles) into vertical column density (VCD), the Air Mass Factor (AMF) must be introduced. The relationship between SCD and VCD is as follows:AMFs are retrieved from the atmospheric radiative transfer model SCIATRAN. The a-priori profiles of ozone, temperature, and pressure used to obtain AMFs are the monthly average profiles from the SCIATRAN profiles database, which are selected by month and latitude. The Fraunhofer absorption, which will have a strong influence on the retrieval of gas concentration, should be removed (Platt and Stutz, 2008). The slant column concentration after deducting Fraunhofer absorption is expressed by the dSCD:
Here,



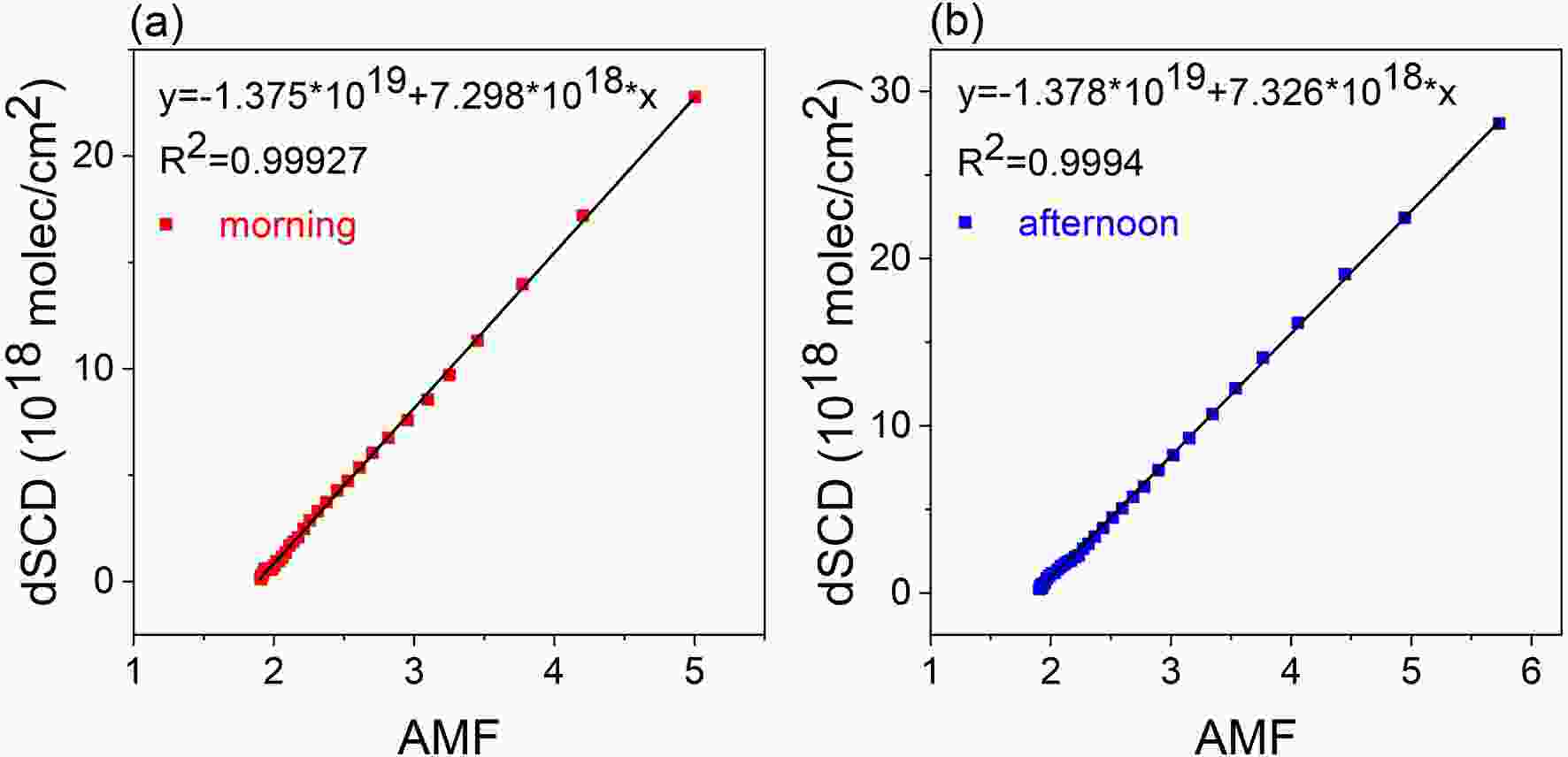
The uncertainty of ozone VCD retrieval through the ZSL-DOAS method comes from the retrieval of SCD and AMF. The comprehensive estimation of uncertainty of ozone SCDs is 1.475% (95% confidence interval, N = 76 902). Parameters including SZA (solar zenith angle), surface albedo, a-priori ozone profile, and wavelength, which would influence the values of AMF, are considered. In this study, the SZA used for calculation is between 35° and 80°, and the surface albedo is between 0.08 and 0.6. The a-priori profile of ozone is obtained from the monthly mean climatology. The detailed parameter nodes to estimate the uncertainty of AMF on wavelength are shown in Table 2. The AMF uncertainty caused by wavelength selection is calculated through



Parameters | Nodes |
SZA | 35°, 40°, 45°, 50°, 55°, 60°, 65°, 70°, 75°, 80° |
Surface albedo | 0.05, 0.1, 0.2, 0.3, 0.4, 0.5, 0.6 |
Wavelength | From 320 to 340 nm in 0.5 nm interval |
Table2. Parameter nodes to estimate the AMF uncertainty on wavelength.
2
2.5. Auxiliary data
The daily ozone VCDs observed by OMI and GOME-2 from January 2017 to February 2020 are obtained for this study. The OMI, launched on 15 July 2004, is onboard the Aura satellite and is a nadir scanning instrument (Xie et al., 2016). The field of view of the OMI can reach 114°, which permits daily global coverage. The OMI can measure ozone in UV (270?380 nm) and VIS (350?500 nm) wavelengths. The spectral resolution of the OMI is 0.5 nm, with high spatial resolution of
GOME-2 is a UV/VIS nadir observation spectrometer, which is onboard the MetOp-A satellite and was launched on 19 October 2006 by the European Space Agency (ESA). The ozone data sets of GOME-2 are retrieved by the GOME-type Direct FITting (GODFIT) v4 algorithm. The wavelength range of the GOME-2 instrument is 240?790 nm. The spectral resolution of GOME-2 is 0.2?0.5 nm, with spatial resolution of

The temperature and ozone profiles used here are obtained from MERRA-2 data and are available every 3 hours. MERRA-2 is an atmospheric reanalysis database, obtained from Goddard Earth Observing System Model, version 5 (GEOS-5) with Atmospheric Data Assimilation System (ADAS) (Ganeshan and Yang, 2019). The spatial resolution of MERRA-2 is

The daily PV data used in this study is obtained from ERA Interim datasets from the ECMWF website (

3.1. Meteorological conditions
The meteorological conditions of the Fildes Peninsula are shown in Figs. 4 and 5, which represent temperatures (at 50 hPa) and PV (on isentropic level of 475 K) respectively.
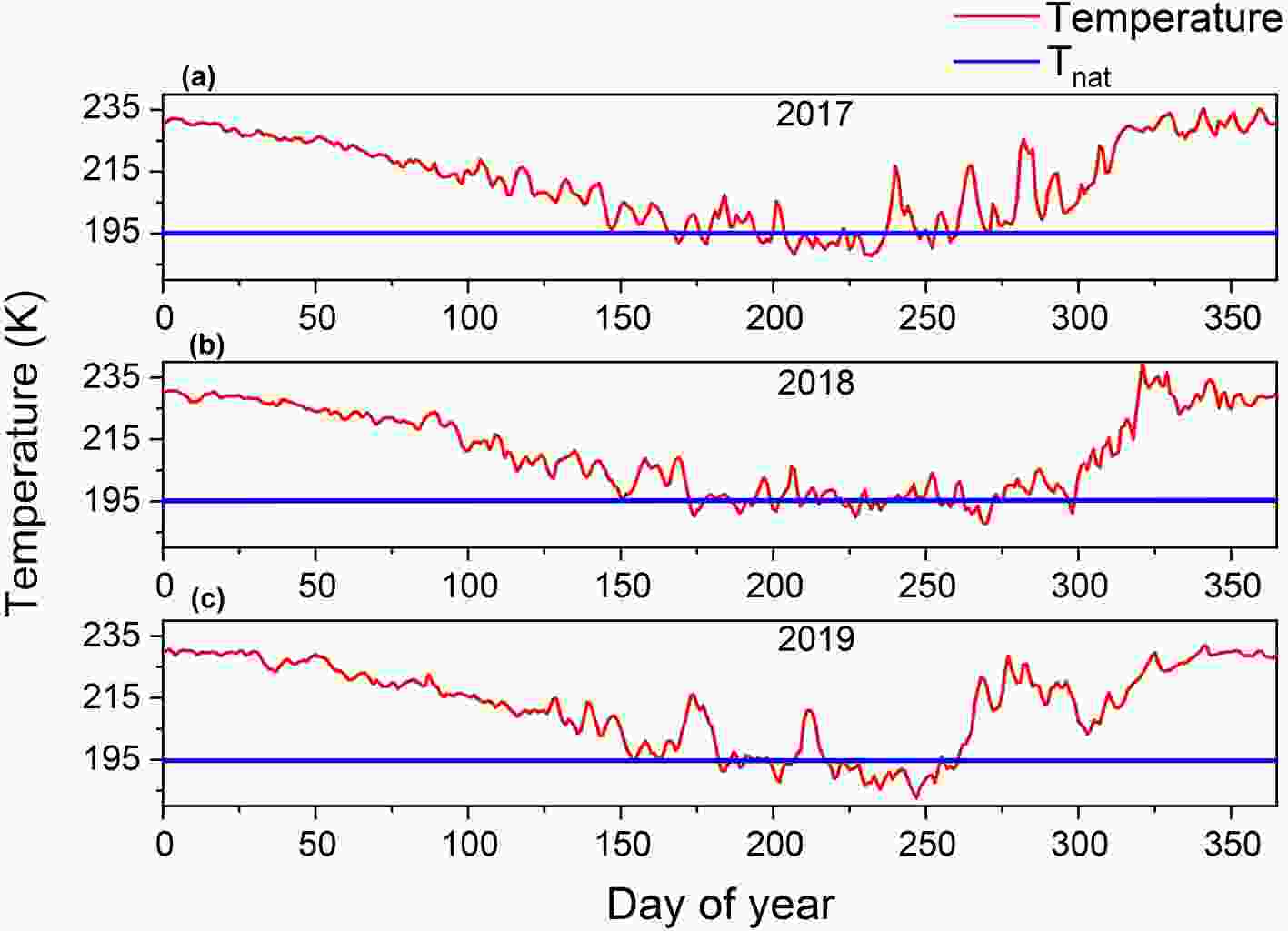
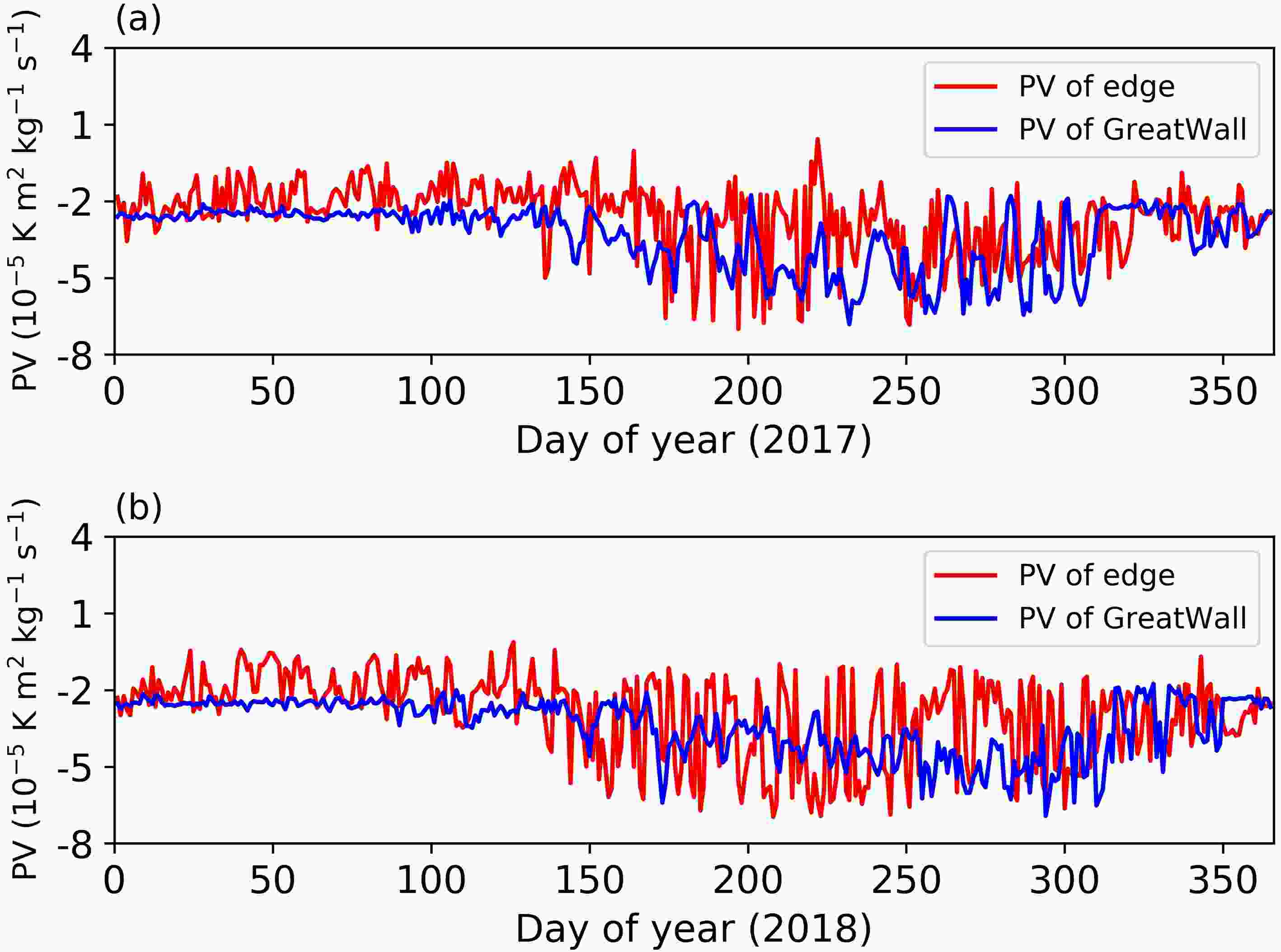
PV is used to represent the capacity for an air mass to rotate in the atmosphere and to define the edge of the polar vortex. PV is calculated using other parameters such as temperature, wind field, etc. The units of PV (potential vorticity units, PVU) are a combination of SI units (


Date | Days inside polar vortex | Days outside polar vortex |
2017 | 273 | 92 |
2018 | 235 | 130 |
2017.9?10 | 39 | 22 |
2018.9?10 | 45 | 16 |
Table3. The number of days inside and outside the polar vortex.
2
3.2. Results of ozone VCDs
Satellite ozone observations may have large biases at high latitudes, especially when the SZAs are large. Therefore, the SZAs used to obtain ozone VCDs from satellite observations are less than 86°. The ZSL-DOAS observations in this study can be used to validate the satellite observations at high latitude.The ozone VCDs retrieved from the ZSL-DOAS instrument, the MERRA-2 dataset and satellite observations from OMI and GOME-2 from January 2017 to February 2020 are shown in Fig. 6a, where the black line located at 220 DU (


The averaged ozone VCDs and ozone hole days for 2017, 2018, and 2019 over the Fildes Peninsula are shown in Table 4. Ozone VCDs start to decline around July with a comparable gradient (around 1.4 DU d?1), which is in agreement with the formation of PSCs in Antarctic winter. Ozone VCDs decline further in the spring, with severe ozone depletion in September and October, and then gradually return to normal levels. During the severe ozone depletion periods in September and October, which lead to the ozone holes (<220 DU), there is a correlation between the polar vortex and ozone concentration, which is discussed in detail in section 3.3. The linear fits of the retrieved ozone VCDs with OMI and GOME-2 satellite observations and the MERRA-2 dataset are shown in Fig. 7. The correlation coefficients (

Date | Average ozone VCDs (DU) | Ozone hole days |
2017 | 295.85 | 16 |
2017.9?10 | 260.76 | 15 |
2018 | 289.32 | 30 |
2018.9?10 | 215.13 | 25 |
2019 | 294.04 | 29 |
2019.9?10 | 283.38 | 22 |
Table4. Averaged ozone VCDs and ozone hole days.
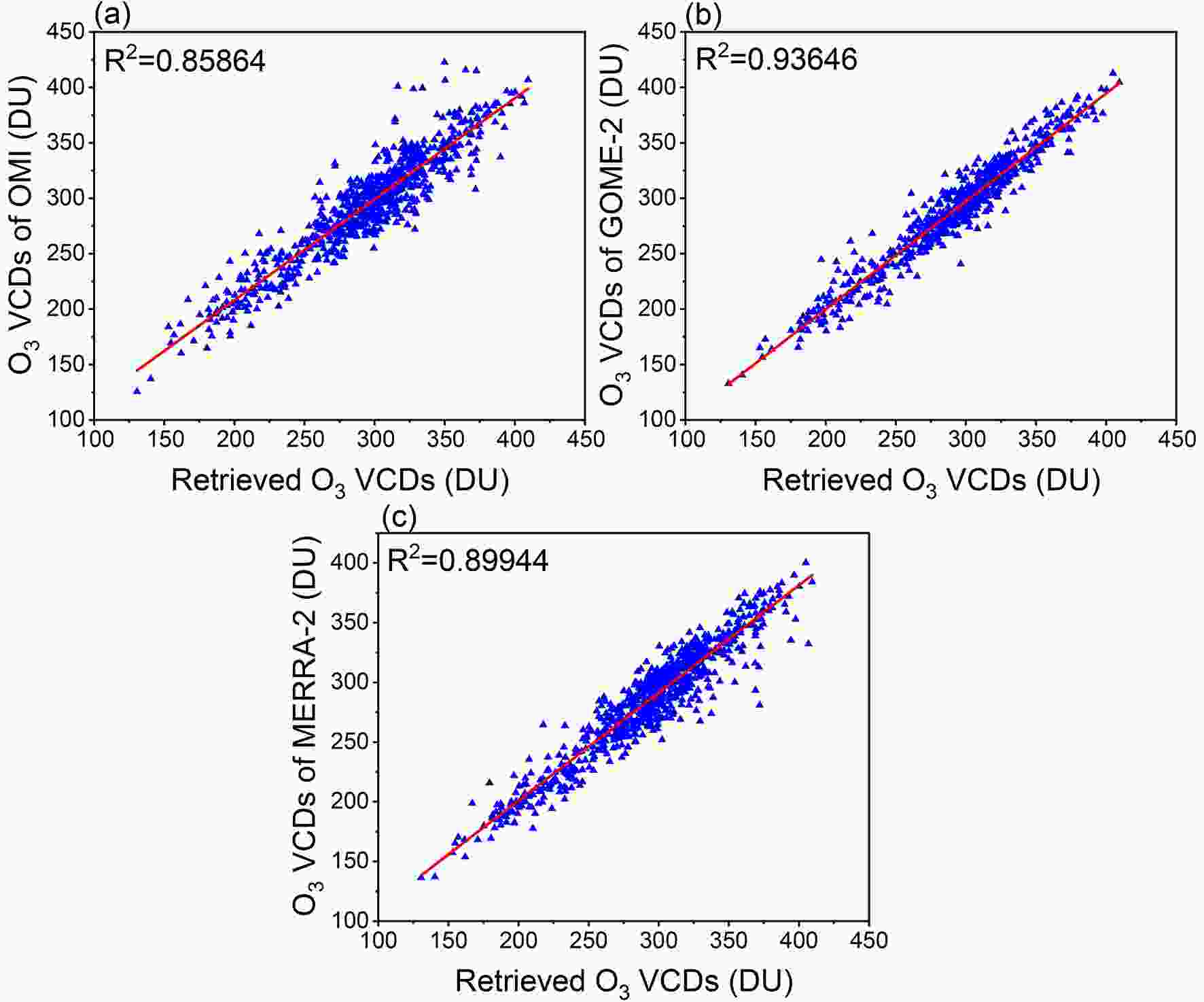
2
3.3. Influence of PV on ozone depletion
The sign of PV is negative in Antarctica while positive in the Arctic. The absolute value of PV is generally greater inside the polar vortex. The PSCs formed inside the polar vortex can activate the halogen species, which lead to severe ozone depletion. The PV, temperatures (at 50 hPa), and retrieved ozone VCDs from September to October during the observation period are shown in Fig. 8. As shown in Figs. 8a-d, the trend of PV and ozone VCDs is similar. In other words, PV is positively correlated with the ozone VCDs. The ozone VCDs fluctuate between 170?405 DU from September to October of 2017. The fluctuations in 2018 are between 150?290 DU. The relationship between PV and ozone VCDs is more obvious in 2017 with greater fluctuations. As shown in Fig. 8a, ozone recovers to its peak values on 22 September, 9 October, 19 October, and 28 October 2017, when Fildes Peninsula is fully outside of the polar vortex. The retrieved ozone VCDs fluctuate with the same pattern as the temperatures at 50 hPa, which means ozone is depleted inside the polar vortex, where the temperature is lower. Therefore, the polar vortex has a strong influence on stratospheric ozone depletion during Antarctic spring.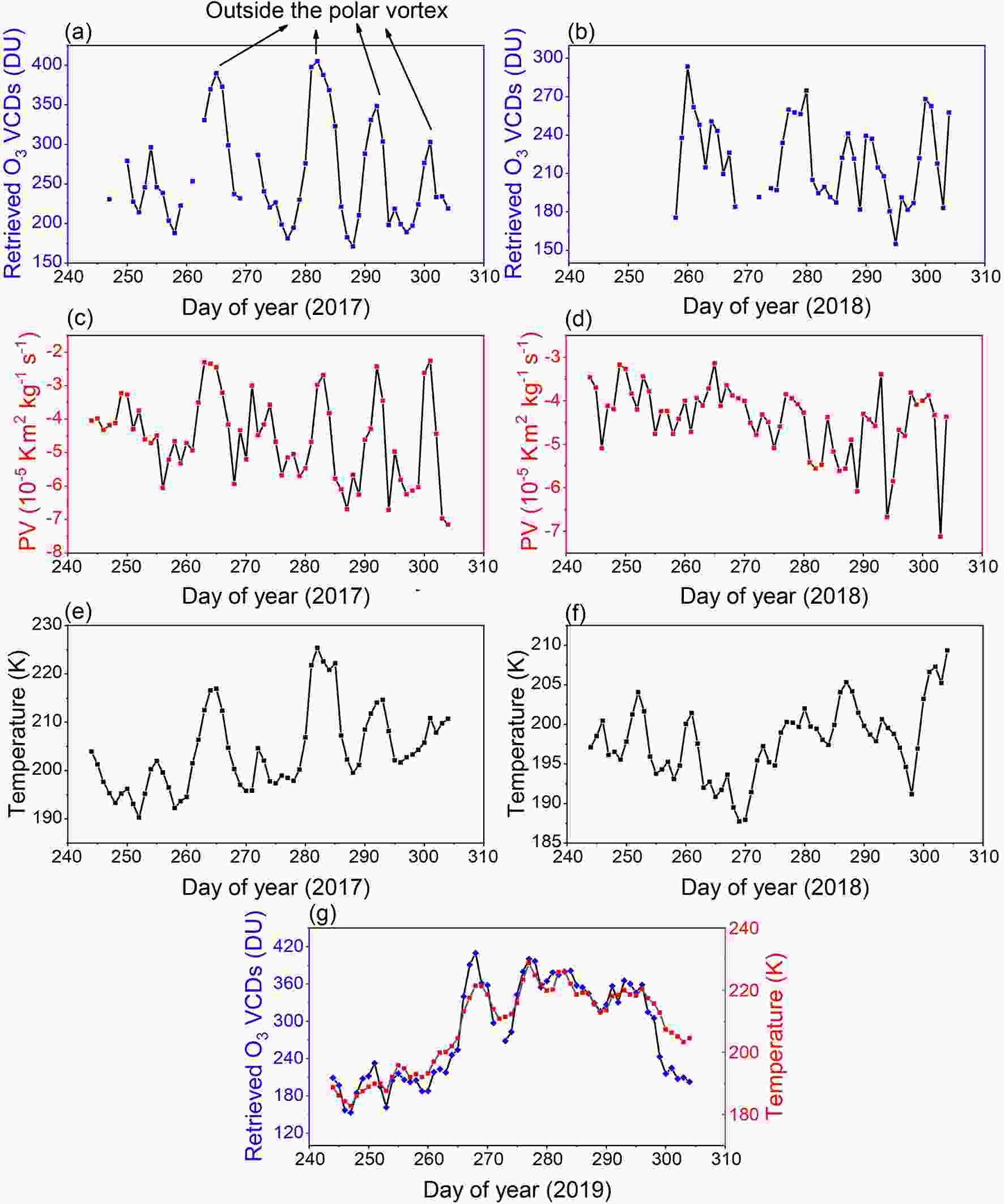
Ozone and PV profiles above the Fildes Peninsula during spring of 2017 and 2018 are analyzed as well. The averaged ozone profiles during the ozone hole periods and non-ozone hole periods from September to October in 2017 are shown in Fig. 9. The averaged ozone profiles and the percentage of ozone loss at different heights indicate that the maximum ozone loss is about 63% at the height of 19.5 km. PV might differ by more than a factor of ten for different heights in the lower stratosphere, which indicates that a small and sensitive height layer should be chosen to discuss its influence on ozone depletion. Therefore, the profile height of 19?20 km, where the photochemical reactions destroying ozone are most severe, was chosen.

Since the observation site is located near the edge of the polar vortex, it is sensitive to the changes of the polar vortex. The synchronized change between ozone and PV indicates the critical influence of the polar vortex on ozone depletion. The profiles of ozone and PV at the height of 19?20 km from September to October in 2017 and 2018 are shown in Fig. 10. The ozone concentration at the height of 19?20 km fluctuates between 0.65?6.87 ppmv and 0.54?7.30 ppmv in 2017 and 2018, respectively. The absolute value of PV shows an obvious increase when the ozone concentration decreases. The ozone depletion in Antarctic spring, which leads to the formation of ozone holes, is closely related to PV. Located at the edge of the polar vortex, the observed data will provide a basis for further analysis and prediction of the inter-annual variation of stratospheric ozone in future.
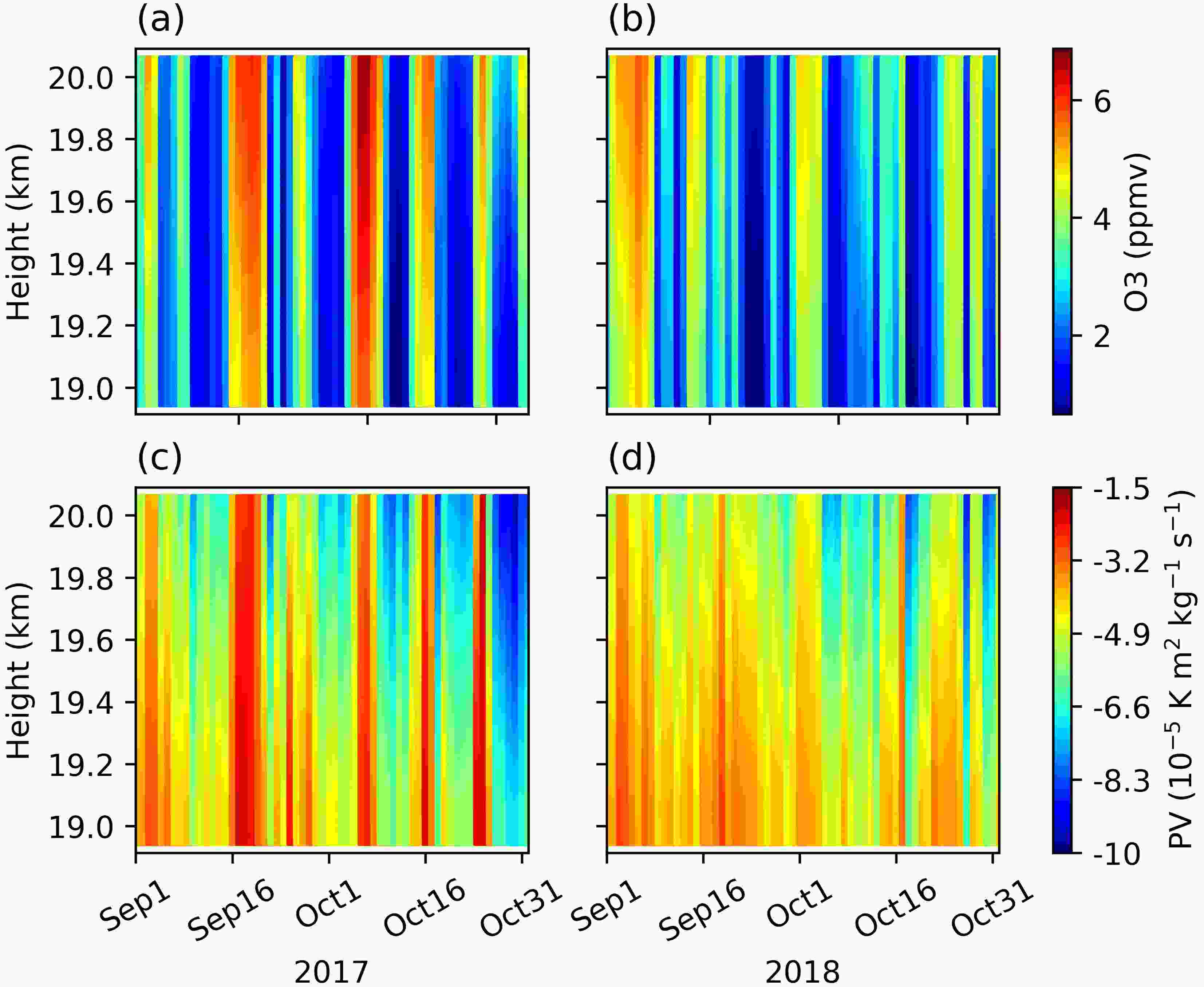

Each spring during the observation period, occurrences of ozone holes over the Fildes Peninsula were detected when the daily ozone VCDs fluctuated sharply. Especially in September 2017, the daily fluctuations of ozone VCDs reached up to 100 DU. The ozone VCDs began to decrease in early winter with a comparable gradient (1.4 DU d?1) throughout the observation period, corresponding with the formation of PSCs. The ozone concentration began to recover at the end of October, and returned to normal levels after November.
In this study, PV was used as an indicator for analysis because it was positively correlated with ozone concentration over Fildes Peninsula in spring. The polar vortex of Antarctic spring has a strong influence on stratospheric ozone depletion.
It should be noted that the uncertainty estimation of the AMF calculation is preliminary, and the uncertainty caused by the a-priori ozone profiles needs further analysis. More accurate a-priori ozone profiles (like column-dependent total ozone profiles) and a better reference spectrum (from direct-sun data) will be used in future research.
Observation of ozone VCDs over Fildes Peninsula will be continually conducted to observe the long-term ozone trends in this region. The observations conducted in this study are also valuable for validating modelled ozone concentrations in this region and contribute to better understanding of ozone recovery and stratosphere-troposphere exchange over the polar vortex edge area.
Acknowledgements. This research was financially supported by the National Natural Science Foundation of China (Grant Nos. 41676184 and 41941011). The authors gratefully acknowledge ECMWF (