0 引言
【研究意义】玉米收获期籽粒含水率显著影响机械粒收质量[1,2,3,4,5,6],在籽粒发育进程中,脱水过程与灌浆同步进行,并持续至籽粒收获,明确玉米籽粒脱水动态及其与灌浆过程的关系,对选育脱水速率快的宜机收品种有重要意义。【前人研究进展】玉米籽粒脱水主要是为了促使籽粒进入休眠状态,以获得逆境耐性[7,8]。MAIORANO等[9]将玉米籽粒水分变化过程划分为迟滞期、灌浆期脱水和生理成熟后脱水3个阶段,而BROOKING[10]将灌浆期脱水称为“生理脱水”(developmental loss of water)。申琳[11]以籽粒含水量上升、平稳和下降的变化动态,将生理成熟前的籽粒发育过程划分为3个阶段。CRANE [12]研究发现一些吐丝期相近的品种,在籽粒含水率降至45%之前籽粒含水量和干物质积累量的变化存在同步性。MA等[13]研究认为,随着籽粒灌浆过程推进,乳线至50%时,籽粒含水率为38.5%,乳线消失时,籽粒含水率为31.2%,但是品种和年份间存在一定差异。李德新等[14]认为灌浆速率与收获期籽粒含水率呈显著正相关。孙月轩[15]、乔江方等[16]研究认为,玉米籽粒含水率变化与灌浆速率显著相关。这些研究表明,籽粒灌浆和脱水过程可能存在一定的联系。【本研究切入点】中国各玉米区多选用熟期相对较长的品种以提高光温资源利用效率,实现玉米高产。但收获时玉米籽粒含水率普遍偏高,影响了机械粒收质量和效益。机械粒收要求的籽粒脱水快与作物高产要求的灌浆期长、灌浆速率高之间相互关系如何?两者能否统一?玉米籽粒灌浆与籽粒脱水过程的关系尚缺乏深入的研究。【拟解决的关键问题】通过系统观测不同品种玉米籽粒干重、鲜重、含水量与含水率的变化动态,明确玉米籽粒脱水过程并探讨该过程与灌浆过程的关系,为适宜机械粒收品种的选育和配套技术推广应用提供理论依据。1 材料与方法
1.1 试验设计
试验于2015和2016年在中国农业科学院新乡综合试验站(35°10′N,113°47′E)进行。种植密度均为75 000株/hm2,田间管理同大田生产。试验处理见表1。Table 1
表1
表1试验处理
Table 1Experimental treatment
年份 Year | 试验处理 Experimental treatment | 玉米品种名称 Maize Cultivar |
---|---|---|
2015 | 6月16日播种,随机区组设计,每品种3次重复,小区长8 m,宽5.4 m,面积43.2 m2 Sowing on 16 June; Randomized block design with three replications; The plots were 8 m long and 5.4 m wide and had an area of 43.2 m2 | 郑单958、先玉335、农华101、农华816、京农科728、中单909、宁玉721、联创808、裕丰303、中科玉505、禾田1号 ZD958, XY335, NH101, NH816, JNK728, ZD909, NY721, LC808, YF303, ZKY505, HT1 |
2016 | 6月4日播种,大区种植,每区长18 m,宽7.8 m,面积140.4 m2 Sowing on 4 June; Big plots; The plots were 18 m long and 7.8 m wide and had an area of 140.4 m2 | 郑单958、先玉335、农华101、农华816、京农科728、中单909、华美1号、真金323、新单58、新单65、辽单575、锦华318、锦华207、金通152、迪卡517、陕单636、丰垦139 ZD958, XY335, NH101, NH816, JNK728, ZD909, HM1, ZJ323, XD58, XD65, LD575, JH318, JH207, JT152, DK517, SD636, FK139 |
新窗口打开
1.2 测定项目与方法
详细观测各品种生育进程。吐丝前,各品种选择标记200株生长一致、无病虫害的代表性植株进行雌穗套袋。吐丝后3 d内统一授粉,以确保取样果穗授粉日期一致。测定时选择统一授粉的5个果穗,取中部籽粒以烘干法测定籽粒含水率。取样间隔5 d一次,为避免错过生理成熟时间,接近生理成熟期取样间隔缩短至1—3 d。每次取样拍照留存籽粒乳线图像,至乳线消失、黑层完全形成判定品种生理成熟日期。测定时如遇降水天气,则该次取样测定顺延1 d。2015年测定自授粉后25 d开始,至生理成熟后26—52 d结束,主要关注籽粒灌浆至生理成熟后的脱水情况;2016年自授粉后11 d开始测定,至生理成熟后16—35 d结束,兼顾籽粒建成后期和灌浆期的含水率变化情况。1.3 测试指标和计算方法
1.3.1 籽粒含水量和含水率含水量(g)=鲜重(g)-干重(g)
含水率(%)=

1.3.2 籽粒脱水速率计算 根据文献记载,细胞原生质含水率在70%—90%[17],籽粒形成初期细胞含水率约为90%[7],玉米籽粒授粉后12 d之前籽粒含水率在80%—90%[18],本研究把籽粒形成初期的含水率规定为90%。用初始籽粒含水率(90%)和生理成熟期籽粒含水率的差值与授粉至生理成熟积温(≥0℃活动积温,下同)的比值计算生理成熟前籽粒平均脱水速率。用生理成熟期籽粒含水率和收获期籽粒含水率差值与生理成熟后积温的比值计算生理成熟后籽粒平均脱水速率;收获期籽粒含水率以生理成熟后第N天(第10—15 d中某一测试当天)的籽粒含水率表示。以初始籽粒含水率与收获期籽粒含水率差值与二者之间积温(总积温)的比值表示籽粒总脱水速率。计算公式如下:
生理成熟前籽粒平均脱水速率(%·(℃·d)-1)=(90%-生理成熟期籽粒含水率(%))/授粉至生理成熟积温(℃·d);
生理成熟后籽粒平均脱水速率(%·(℃·d)-1)=(生理成熟期籽粒含水率(%)-收获期籽粒含水率(%))/生理成熟后积温(℃·d);
籽粒脱水速率(%·(℃·d)-1)=(90%-收获期籽粒含水率(%))/总积温(℃·d)。
1.3.3 灌浆速率计算 以生理成熟期百粒干重与授粉至生理成熟积温的比值计算平均灌浆速率,公式为:
籽粒脱水速率(%·(℃·d)-1)=(90%-收获期籽粒含水率(%))/总积温(℃·d)。
1.3.4 籽粒含水率模型 为了避免环境条件差异对不同熟期品种籽粒脱水时间造成的影响,本研究以相互关系更为稳定[19,20,21]的授粉后积温与籽粒含水率进行分析,建立两者的回归关系模型,以授粉后积温估算籽粒含水率。通过观察籽粒含水率与积温的散点图分布形态,选择Logistic Power非线性增长模型建立回归模型,模型形式如下:
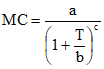
式中,a、b、c为模型参数,T(℃·d)为自变量,即授粉后积温,MC(%)为因变量,即籽粒含水率。a为模型极值,即籽粒初始含水率,本研究将a参数设定为90。利用CurveExpert Professional统计软件的非线性曲线拟合功能,得出不同品种回归模型b、c参数的最优估计值,根据拟合度(R2)和F检验评价模型优劣。
1.3.5 籽粒含水量和鲜重模型 采用Rational Function非线性增长模型分别对籽粒含水量和鲜重与授粉后积温的关系进行回归分析,模型如下:
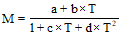
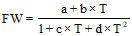
式中,a,b,c,d为模型参数,T(℃·d)为自变量,即授粉后积温,M(g)和FW(g)为因变量,分别
为籽粒含水量和籽粒鲜重。
1.3.6 籽粒干重模型 采用Ratkowsky Model非线性增长模型对籽粒干重与授粉后积温的关系进行回归分析,模型如下:

式中,a,b,c为模型参数,T(℃·d)为自变量,即授粉后积温,DW(g)为因变量,即籽粒干重,e为自然对数的底数。
1.4 数据处理
用Excel 2007进行数据计算和作图。用Curve Expert Professional 2.2.0 进行籽粒含水率、干重、鲜重和含水量动态变化的曲线拟合。用SPSS 16.0进行相关分析,相关分析结果用Pearson相关系数表示,显著性检验采用Two-tailed检验。模型建立所用籽粒含水率、含水量、鲜重和干重数据为逐次测定结果的均值,积温数据以中国气象局数据中心“中国地面气候资料日值数据集(V3.0)”[22]新乡站(站点编号:53986,距试验点直线距离24.5 km)逐日平均温度,根据授粉时间和测定时间累加计算。2 结果
2.1 不同玉米品种的生育进程
试验条件下,参试玉米品种播种和出苗日期相同,吐丝日期和生理成熟日期存在差异。2015年,11个供试品种的吐丝期和生理成熟期最大相差7 d和26 d,授粉至生理成熟平均61 d,变化范围为48—69 d,平均积温为1 393℃·d,变化范围为1 166—1 553℃·d;2016年17个供试品种的吐丝期、生理成熟期最大相差8 d和23 d,授粉至生理成熟平均54 d,变化范围为43—60 d,灌浆期平均积温为1 415℃·d,变化范围为1 179—1 553℃·d(表2)。Table 2
表2
表2不同品种玉米生育进程与积温
Table 2Growth stages of maize cultivars
年份 Year | 品种 Cultivar | 出苗 Emergence (M-D) | 吐丝 Silking (M-D) | 授粉 Pollination (M-D) | 生理成熟 Physiological maturity | 授粉-生理成熟天数 Days from pollination to physiological maturity (d) | 授粉-生理成熟积温 Accumulated temperature from pollination to physiological maturity (℃·d) |
---|---|---|---|---|---|---|---|
2015 | JNK728 | 6-23 | 8-7 | 8-9 | 9-28 | 50 | 1200 |
NH816 | 6-23 | 8-10 | 8-12 | 10-8 | 57 | 1316 | |
NH101 | 6-23 | 8-9 | 8-12 | 10-11 | 60 | 1365 | |
ZD909 | 6-23 | 8-9 | 8-12 | 10-15 | 64 | 1439 | |
ZD958 | 6-23 | 8-9 | 8-10 | 10-14 | 65 | 1475 | |
XY335 | 6-23 | 8-9 | 8-10 | 10-14 | 65 | 1475 | |
YF303 | 6-23 | 8-9 | 8-10 | 10-18 | 69 | 1553 | |
NY721 | 6-23 | 8-10 | 8-13 | 10-19 | 67 | 1485 | |
LC808 | 6-23 | 8-10 | 8-12 | 10-15 | 64 | 1439 | |
ZKY505 | 6-23 | 8-10 | 8-13 | 10-15 | 63 | 1410 | |
HT1 | 6-23 | 8-3 | 8-6 | 9-23 | 48 | 1166 | |
2016 | JNK728 | 6-9 | 7-26 | 7-26 | 9-12 | 48 | 1291 |
NH816 | 6-9 | 7-29 | 7-29 | 9-20 | 53 | 1389 | |
NH101 | 6-9 | 7-28 | 7-28 | 9-20 | 54 | 1421 | |
ZD909 | 6-9 | 7-30 | 7-31 | 9-28 | 59 | 1501 | |
ZD958 | 6-9 | 7-31 | 7-31 | 9-28 | 59 | 1501 | |
XY335 | 6-9 | 7-30 | 7-30 | 9-26 | 58 | 1490 | |
DK517 | 6-9 | 7-28 | 7-28 | 9-21 | 55 | 1443 | |
ZJ323 | 6-9 | 7-29 | 7-29 | 9-20 | 53 | 1389 | |
SD636 | 6-9 | 7-26 | 7-26 | 9-19 | 55 | 1459 | |
LD575 | 6-9 | 7-29 | 7-29 | 9-20 | 53 | 1389 | |
HM1 | 6-9 | 7-25 | 7-25 | 9-13 | 50 | 1344 | |
FK139 | 6-9 | 7-23 | 7-24 | 9-5 | 43 | 1179 | |
JT152 | 6-9 | 7-29 | 7-29 | 9-23 | 56 | 1457 | |
JH207 | 6-9 | 7-29 | 7-29 | 9-23 | 56 | 1457 | |
JH318 | 6-9 | 7-28 | 7-28 | 9-26 | 60 | 1553 | |
XD65 | 6-9 | 7-28 | 7-28 | 9-19 | 53 | 1400 | |
XD58 | 6-9 | 7-25 | 7-25 | 9-15 | 52 | 1394 |
新窗口打开
2.2 玉米籽粒含水率与积温的关系
参试品种籽粒含水率与授粉后积温的关系呈现先快速下降后逐渐减缓的单调变化趋势。利用各品种籽粒含水率与其授粉后积温,以Logistic Power模型拟合建立回归模型,能够很好地反映不同品种籽粒含水率与其授粉后积温的关系,且不受年际变化影响(图1)。全部参试品种拟合度R2值在0.962—0.995之间,F检验均达到极显著水平(表3)。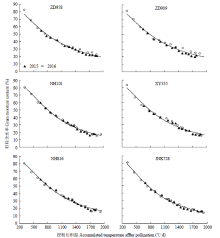
图1不同品种籽粒含水率预测模型
-->Fig. 1Prediction model of grain moisture content of different cultivars
-->
Table 3
表3
表3不同玉米品种Logistic Power模型拟合结果
Table 3Fitting results of Logistic Power model in different maize cultivars
年份 Year | 品种 Cultivar | b | c | R2 | 授粉—28%含水率积温 Accumulated temperature from pollination to 28% MC (℃·d) | 授粉—25%含水率积温 Accumulated temperature from pollination to 25% MC (℃·d) |
---|---|---|---|---|---|---|
2015 | HT1 | 811.189 | 2.337 | 0.962** | 1140 | 1221 |
ZKY505 | 877.380 | 1.993 | 0.984** | 1307 | 1417 | |
LC808 | 895.960 | 1.943 | 0.986** | 1349 | 1465 | |
NY721 | 892.299 | 1.682 | 0.968** | 1431 | 1575 | |
YF303 | 950.148 | 1.769 | 0.985** | 1489 | 1630 | |
2016 | FK139 | 836.987 | 1.829 | 0.994** | 1293 | 1411 |
DK517 | 838.297 | 1.833 | 0.989** | 1293 | 1412 | |
JH207 | 851.805 | 1.834 | 0.986** | 1314 | 1434 | |
HM1 | 915.269 | 2.121 | 0.992** | 1331 | 1436 | |
XD65 | 843.911 | 1.742 | 0.995** | 1332 | 1461 | |
JT152 | 908.628 | 1.961 | 0.986** | 1363 | 1479 | |
XD58 | 881.079 | 1.750 | 0.994** | 1388 | 1521 | |
LD575 | 884.834 | 1.750 | 0.986** | 1394 | 1528 | |
ZJ323 | 899.704 | 1.770 | 0.988** | 1410 | 1544 | |
SD636 | 936.666 | 1.921 | 0.984** | 1417 | 1540 | |
JH318 | 920.968 | 1.770 | 0.994** | 1443 | 1580 | |
2015-2016 | JNK728 | 834.19 | 1.952 | 0.993** | 1253 | 1361 |
NH101 | 904.387 | 2.029 | 0.993** | 1338 | 1448 | |
XY335 | 886.981 | 1.879 | 0.982** | 1354 | 1475 | |
NH816 | 903.747 | 1.944 | 0.989** | 1360 | 1477 | |
ZD958 | 896.136 | 1.612 | 0.988** | 1467 | 1621 | |
ZD909 | 925.174 | 1.687 | 0.986** | 1482 | 1630 |
新窗口打开
不同品种籽粒脱水过程中累积的授粉后积温存在较大差异(表3),2015年11个品种籽粒含水率降至28%所需要的授粉后积温平均为1 361℃·d,变化范围为1 140—1 489℃·d;降至25%所需要的授粉后积温平均为1 484℃·d,变化范围为1 221—1 630℃·d。2016年17个品种籽粒含水率降至28%所需要的授粉后积温平均为1 367℃·d,变化范围为1 253—1 482℃·d;降至25%所需要的授粉后积温平均为1 492℃·d,变化范围为1 361—1 630℃·d。
以2015和2016年各品种生理成熟时测定的籽粒含水率与以Logistic Power回归模型计算的籽粒含水率进行比较(图2),两年间测定的生理成熟籽粒含水率与模型预测的生理成熟籽粒含水率具有极显著的正相关关系(P<0.01),均值比较(paired sample T test)差异不显著。建立的Logistic Power回归模型具有良好的籽粒含水率预测性能。
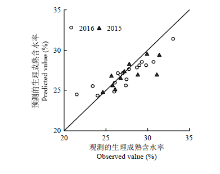
图2生理成熟期籽粒含水率模型预测值与测定值的关系
-->Fig. 2Relationship between predicted value and observed value of grain moisture content at physiological maturity
-->
用Logistic Power方程对2015和2016年所有参试品种籽粒含水率与授粉后积温拟合,得到参试品种籽粒含水率预测模型:MC=90/[1+(T/883.187)]1.851(R2=0.974**,n=423),回归方程显著性达到极显著水平(P<0.01)。实测籽粒含水率数据多数分布于模型95%置信区间范围内,可以很好的描述现有品种籽粒含水率变化与授粉后积温的相互关系(图3)。
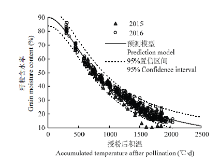
图3参试品种籽粒含水率预测模型
-->Fig. 3Prediction model of grain moisture content of all testing cultivars
-->
基于籽粒含水率与授粉后积温回归建立的预测模型,以黄淮海夏玉米机械粒收要求的28%、25%含水率[2, 23]为标准,当前品种籽粒含水率降至28%,需要授粉后积温为1 357℃·d,其95%置信区间为1 126— 1 646℃·d,参试品种达到相同的28%籽粒含水率所需积温相差为520℃·d;籽粒含水率降至25%,需要授粉后积温为1 480℃·d,置信区间为1 218—1 810℃·d,两者相差达592℃·d(表4)。
Table 4
表4
表4玉米籽粒含水率与需要的授粉后积温
Table 4Maize grain moisture content and the related accumulated temperature after pollination (°C·d)
数值范围 Value range | 28%含水率 28%MC | 25%含水率 25%MC | 20%含水率 20%MC | 15%含水率 15%MC |
---|---|---|---|---|
预测值 Predicted value | 1357 | 1480 | 1738 | 2107 |
95%置信区间上限Upper bound of 95% confidence interval | 1646 | 1810 | 2159 | 2672 |
95%置信区间下限 Lower bound of 95% confidence interval | 1126 | 1218 | 1405 | 1665 |
新窗口打开
2.3 玉米籽粒脱水与灌浆进程
2016年,试验测定由籽粒授粉后11 d开始,重点分析籽粒建成后期和灌浆过程中,水分和干物质变化与籽粒含水率持续降低之间的关系。分别将17个参试品种的籽粒干重、籽粒含水量、籽粒鲜重与授粉后积温建立回归模型,3个关系模型均达到极显著水平,拟合结果如下:DW=30.1532/[1+e(3.6193-0.0053T)](R2=0.91**,n=262);
M=(8.4648-0.0002T)/(1-0.0013T+7.8999×10-7T2)
(R2=0.84**,n=262);
FW=(0.3173-0.0373T)/(1-0.0008T+7.2421×10-7T2)(R2=0.78**,n=262)。
结果显示,DW随着T的增加先增加后趋于稳定,灌浆速率在684℃·d积温时达到最大值,435—932℃·d积温之间为DW的线性增长期;M随着T的增加先增加后降低,自授粉后至籽粒灌浆中期,M均高于DW,此阶段籽粒仍在净吸收水分,M在828℃·d积温时达到最高值,此后M开始不断降低。FW随着T的增加呈先增加后降低的趋势,在1 171℃·d积温时达最大值,其变化曲线是DW与M变化的复合曲线(图4)。
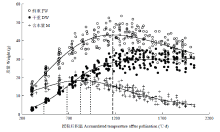
图4玉米籽粒含水量、鲜重和干重随授粉后积温的变化
-->Fig. 4Maize grain moisture, fresh weight and dry weight change with the accumulated temperature after pollination
-->
2.4 玉米籽粒脱水特性与灌浆特性的相关关系
对籽粒脱水与灌浆参数的相关分析表明(表5),生理成熟期籽粒含水率与收获期籽粒含水率呈正相关,2016年达到极显著水平;生理成熟期籽粒含水率与授粉至生理成熟天数及相应积温之间,2015年极显著负相关,2016年相关不显著;生理成熟期籽粒含水率与生理成熟前籽粒平均脱水速率之间没有显著相关性;生理成熟前籽粒平均脱水速率与授粉至生理成熟天数及相应的积温之间呈极显著负相关,表明灌浆期越短,生理成熟前籽粒平均脱水速率越快;籽粒生理成熟前、后及总脱水速率与灌浆速率之间相关性不显著;生理成熟期百粒干重与授粉至生理成熟天数及相应积温呈显著或极显著正相关,与平均灌浆速率呈极显著正相关关系,即灌浆期越长、灌浆速率越大,粒重越大。Table 5
表5
表5玉米籽粒脱水与灌浆参数相关分析
Table 5Correlation analysis of dehydration parameters and filling parameters of maize
年份 | 授粉-生理 | 授粉-生理 | 生理成熟期含水率 | 收获期含水率 | 生理成熟期 | 平均灌浆速率 | 生理成熟前平均 | 生理成熟后平均 | 总脱水 | |
---|---|---|---|---|---|---|---|---|---|---|
Year | 成熟天数 | 成熟积温 | MC at physiological maturity | MC at | 百粒干重 | Average | 脱水速率 | 脱水速率 | 速率 | |
Days from pollination to physiological maturity | Accumulated temperature from pollination to physiological maturity | harvest | 100-kernel dry weight at physiological maturity | filling rate | Average dehydration rate before physiological maturity | Average dehydration rate after physiological maturity | Total dehydration rate | |||
授粉-生理成熟天数 | 2015 | 1 | 0.995** | -0.815** | 0.192 | 0.785** | -0.273 | -0.940** | -0.800** | -0.942** |
Days from pollination to physiological maturity | 2016 | 1 | 0.991** | -0.309 | -0.248 | 0.642** | 0.176 | -0.794** | 0.06 | -0.696** |
授粉-生理成熟积温 | 2015 | 1 | -0.810** | 0.194 | 0.791** | -0.269 | -0.943** | -0.806** | -0.950** | |
Accumulated temperature | 2016 | 1 | -0.356 | -0.299 | 0.589* | 0.107 | -0.773** | 0.067 | -0.677** | |
from pollination to physiological maturity | ||||||||||
生理成熟期含水率 | 2015 | 1 | 0.317 | -0.906** | -0.193 | 0.577 | 0.620* | 0.627* | ||
MC at physiological | 2016 | 1 | 0.843** | -0.418 | -0.304 | -0.316 | 0.055 | -0.274 | ||
maturity | ||||||||||
收获期含水率 | 2015 | 1 | -0.23 | -0.610* | -0.433 | -0.477 | -0.432 | |||
MC at harvest | 2016 | 1 | -0.283 | -0.163 | -0.271 | -0.411 | -0.407 | |||
生理成熟期百粒干重 | 2015 | 1 | 0.374 | -0.595 | -0.53 | -0.617* | ||||
100-kernel dry weight at physiological maturity | 2016 | 1 | 0.866** | -0.301 | 0.046 | -0.212 | ||||
平均灌浆速率 | 2015 | 1 | 0.492 | 0.345 | 0.455 | |||||
Average filling rate | 2016 | 1 | 0.114 | 0.004 | 0.161 | |||||
生理成熟前平均脱水 | 2015 | 1 | 0.768** | 0.979** | ||||||
速率 | 2016 | 1 | -0.092 | 0.879** | ||||||
Average dehydration | ||||||||||
rate before physiological maturity | ||||||||||
生理成熟后平均脱水 | 2015 | 1 | 0.866** | |||||||
速率 | 2016 | 1 | 0.368 | |||||||
Average dehydration rate after physiological maturity | ||||||||||
总脱水速率 | 2015 | 1 | ||||||||
Total dehydration rate | 2016 | 1 |
新窗口打开
3 讨论
3.1 玉米籽粒脱水和积温的关系及理想脱水的品种类型
研究表明,玉米籽粒含水率随生育进程的推进呈现单调递减的趋势。但受温度、湿度、风速等环境因素的影响,籽粒含水率变化动态有明显差异[10, 24-26]。对于不同熟期的品种,由于其籽粒脱水进程处于不同的环境条件下,难以比较品种间籽粒脱水速率的差异。积温作为作物生育进程的估算指标应用广泛[27,28],在籽粒脱水动态的研究中也有应用[19,20,21]。本研究以授粉后积温与籽粒含水率建立回归模型,具有优良的预测精度。尽管2015和2016年的气温和降水[29]等条件差异较大,但是该模型在年际间也表现出较好的预测稳定性,可以作为不同积温条件下玉米籽粒含水率的估算方法。这表明,虽然籽粒含水率变化过程受多种外界环境因素影响,但温度是其变化的决定性影响因素。两年22个参试品种拟合得到的籽粒含水率回归模型显示,现有品种降至适于机械籽粒收获的28%含水率需要授粉后积温1 126—1 646℃·d,平均为1 357℃·d;降至25%含水率需要授粉后积温1 218—1 810℃·d,平均1 480℃·d。这表明,参试的黄淮海夏玉米品种籽粒脱水至适宜机械粒收标准,所需积温相差达到520—592℃·d,以该区常年气象条件分析,品种间达到适宜机械粒收标准的时间差距将达30—45 d。显然,接近模型置信区间下限的品种更适合在农时相对紧张的黄淮海夏玉米区实现机械籽粒收获。3.2 玉米籽粒脱水与灌浆的关系
随着籽粒灌浆进程的推进,淀粉、蛋白质、油脂等合成产物不断充实籽粒,水分则不断被消耗和替代,籽粒含水率呈现不断下降的趋势,籽粒灌浆与脱水应存在某种关联性。本研究显示,授粉后籽粒含水率呈现单调下降的趋势,这一单调过程结合籽粒干重、籽粒含水量的变化分析,可分为两个阶段:(1)第一阶段是籽粒建成至线性灌浆期结束。籽粒水分处于净吸收的状态,在线性灌浆速率达到峰值后,籽粒含水量也达到最高值,较高的籽粒含水量将维持至线性灌浆期结束。由于籽粒干重增长迅速,灌浆速率大于含水量增长速率,两者变化的差异形成了籽粒含水率变化曲线前段由平缓下降至加速下降的形态。因此,这一阶段籽粒脱水速率是由籽粒灌浆速率所决定的。(2)第二阶段自线性灌浆期结束至籽粒收获。线性灌浆期结束后,籽粒干物质积累速度逐渐减缓,籽粒干重至生理成熟时达到最大值。同时,籽粒水分开始净散失,籽粒含水率下降主要是由于水分散失造成的。GAMBIN等[30]测定了不同品种灌浆期籽粒含水率变化,认为籽粒含水量达到最大起至生理成熟,这一阶段的脱水速率决定了籽粒灌浆期长度,而脱水速率由含水量下降速率和干物质积累速率共同作用。上述结果与本研究分析的第一阶段的过程类似。但这一阶段的终点并非是生理成熟,而是线性灌浆期的结束。籽粒含水率变化的第二阶段是更纯粹的水分散失过程。生理成熟后籽粒含水率变化属于第二阶段,MISEVIC等[31]研究认为高油玉米生理成熟后籽粒脱水速率较普通玉米慢,张立国等[32]发现淀粉、蛋白质和油脂等含量与生理成熟后籽粒脱水速率显著相关,MATHRE等[33]认为,玉米生理成熟后籽粒脱水速率受果穗直径、行粒数等因素影响,张立国等[34]研究发现,穗粗、穗行数、粒宽与玉米生理成熟后籽粒脱水速率呈显著或极显著正相关,穗长与脱水速率呈极显著负相关。这些研究表明籽粒组分、籽粒形态、果穗形态等性状的差异会对含水率变化的第二阶段起到关键作用。
上述分析表明,籽粒灌浆速率是籽粒含水率变化重要但不唯一的影响因素。相关分析结果显示,生理成熟前、后籽粒的脱水速率以及总脱水速率均与灌浆速率之间无显著相关性,不能简单地以籽粒灌浆的快慢来判断籽粒脱水的快慢。籽粒含水率的变化以线性灌浆期结束为界,分为前后两个阶段。籽粒灌浆速率主要影响了籽粒含水率变化的前期阶段,而籽粒水分的散失速率是后期,特别是生理成熟后含水率变化的决定因素。评价适宜机械粒收的品种,既要关注籽粒灌浆速率,还需要注重影响籽粒含水量散失的性状。
3.3 玉米生理成熟期籽粒含水率与灌浆期时长的关系
生产中普遍存在早熟品种生理成熟期籽粒含水率低,晚熟品种生理成熟期籽粒含水率高的认识。本研究结果显示,授粉至生理成熟天数与总脱水速率呈极显著负相关关系(表5),这表明现有品种存在早熟脱水速率快,晚熟脱水速率慢的整体趋势。但不同品种生理成熟期籽粒含水率有较大差异[35],生理成熟前籽粒脱水速率与生理成熟期籽粒含水率之间没有显著相关性(表5),存在熟期短但生理成熟期含水率高的品种,也存在熟期长而生理成熟期含水率低的品种。以试验品种ZD958和XY335为例分析,两品种生育期基本一致(授粉至生理成熟所需积温分别约为1 488℃·d和1 483℃·d),但生理成熟期含水率分别为28.4%和24.9%[35],灌浆期时长并不是决定生理成熟期籽粒含水率的首要因素。灌浆期长度和灌浆速率高低与百粒重间存在显著正相关关系(表5),而百粒重是影响产量的关键因素[36,37]。追求合理的产量必须要保证合理的灌浆期时长,那么留给籽粒降低水分含量的时间就会相应减少。在农时紧张的黄淮海夏玉米区,就需要关注品种籽粒水分散失快慢的差异,以协调产量和适宜机械粒收含水率要求之间的关系。因此,在机械粒收品种的选育上,需结合品种熟期要求并筛选籽粒脱水快的品种,兼顾光温资源的高效利用与机械粒收,实现产量和生产效率的协同提高。同时,可以利用生理成熟期籽粒含水率与授粉至生理成熟积温建立玉米品种机械粒收适宜性的评价和选育标准,便于生产中机械粒收品种的筛选应用。
4 结论
授粉后积温与籽粒含水率、籽粒含水量、籽粒干重及籽粒鲜重的变化动态间均具有极显著的非线性关系,可以用授粉后积温建立上述指标的预测模型。其中,籽粒含水率与授粉后积温符合Logistic Power模型关系,在品种和年际间具有预测稳定性,可以用来预测籽粒含水率变化。现有品种降至适于机械籽粒收获的28%含水率需要授粉后积温1 126—1 646℃·d,平均1 357℃·d;降至25%含水率需要授粉后积温1 218—1 810℃·d,平均1 480℃·d。籽粒含水率变化由籽粒灌浆和籽粒脱水两个关键因素分阶段主导,评价适宜机械粒收的品种,不仅要注意籽粒灌浆特性和熟期,还要关注籽粒脱水特性的选择。The authors have declared that no competing interests exist.
参考文献 原文顺序
文献年度倒序
文中引用次数倒序
被引期刊影响因子
[1] | . 研究玉米品种、子粒水分含量、产量水平、种植密度、种植行距、植株高度、穗位高度及收割速率等因素对玉米机械收粒质量(子粒破碎率、杂质率和损失率)的影响。结果表明,子粒含水量与机收时子粒破碎率、损失率和杂质率呈显著相关,子粒水分含量越高,机收子粒破碎率和杂质率越高,但田间损失率越低。不同来源品种机收质量特性表现出较大差异。 研究玉米品种、子粒水分含量、产量水平、种植密度、种植行距、植株高度、穗位高度及收割速率等因素对玉米机械收粒质量(子粒破碎率、杂质率和损失率)的影响。结果表明,子粒含水量与机收时子粒破碎率、损失率和杂质率呈显著相关,子粒水分含量越高,机收子粒破碎率和杂质率越高,但田间损失率越低。不同来源品种机收质量特性表现出较大差异。 |
[2] | . 设置不同玉米品种和收获时期试验,研究黄淮海地区夏玉米子粒机械收获的可能性及影响收获质量的因素。结果表明,选择适宜品种和收获时期,在黄淮海小麦/玉米一年两作区实施夏玉米机械直接收获子粒是可行的。影响收粒质量的主要因素是子粒水分含量,随含水量增加,机收时子粒损失率、破碎率和杂质率明显上升,适宜子粒收获的含水量建议控制在27%以内。 设置不同玉米品种和收获时期试验,研究黄淮海地区夏玉米子粒机械收获的可能性及影响收获质量的因素。结果表明,选择适宜品种和收获时期,在黄淮海小麦/玉米一年两作区实施夏玉米机械直接收获子粒是可行的。影响收粒质量的主要因素是子粒水分含量,随含水量增加,机收时子粒损失率、破碎率和杂质率明显上升,适宜子粒收获的含水量建议控制在27%以内。 |
[3] | Breeding for faster grain dry-down in corn is an essential objective of modern corn breeding programs all over the world due to important economic reasons. A reliable, large scale, fast, non-destructive wood moisture Voltcraft FM-200 Humidity meter was used to successively measure the grain moisture of field corn individual plants for a large number of corn genotypes, hybrids and inbred lines, in different stages of Pioneer corn breeding program, during 2010-2012. A calibration curve (successive determinations of the grain moisture by using in parallel the wood moisture meter readings and grain moisture obtained by standard gravimetric method) has been used to transform the wood moisture meter readings in estimated % grain moisture (EPGM). DDIND values, a propose selection index, computed as the slope between EPGM and measurement timing, have been used to apply ANOVA analysis; Preliminary results showed that the methods allowed identification of real genotypic differences and advance genotypes with fast DDR. Using the components of variances obtained in ANOVA analysis, an estimate of the heritability of the DDIND was computed. The relative large value of the heritability, of more than 0.50 (50%),脗聽 suggested the efficiency of the method in detecting genotypic variations and that selecting脗聽 by using DDIND for rapid dry down would result in an efficient improvement of this important agronomic trait of modern corn inbred lines and hybrids. |
[4] | . 机械粒收是玉米收获技术发展的方向,是玉米实现全程机械化、转变生产方式的关键。当前,籽粒收获过程中破碎率高的问题不仅降低玉米等级和销售价格,而且导致收获产量下降,并增大烘干成本、增加安全贮藏的难度,是推广机械粒收技术面临的重要问题。玉米不同基因型间籽粒破碎率存在显著差异,抗破碎特性是可遗传的性状,可通过育种培育抗破碎率的品种;不同收获机械和作业参数对籽粒破碎率有显著影响,选择轴流式收获机,并根据玉米生长、成熟和籽粒含水率状况及时检查与调试收获机参数是保证低破碎率的有效措施;生态环境因素对破碎率也有显著的影响,籽粒形成、自然干燥和收获期的光照、温度、湿度等因素均会影响到籽粒硬度、容重、含水率和质地等与籽粒破碎相关的特性;种植密度、水肥管理、收获时期等栽培管理措施对籽粒破碎率也会产生明显的影响。因此,针对不同区域生态环境条件,应选择适宜生育期内能与当地光温资源匹配的品种以及确定品种适宜的种植区域。合理种植密度、优化氮肥管理和适量灌溉有利于降低破碎率,而选择在最佳收获期收获是降低籽粒破碎率的最有效措施。 . 机械粒收是玉米收获技术发展的方向,是玉米实现全程机械化、转变生产方式的关键。当前,籽粒收获过程中破碎率高的问题不仅降低玉米等级和销售价格,而且导致收获产量下降,并增大烘干成本、增加安全贮藏的难度,是推广机械粒收技术面临的重要问题。玉米不同基因型间籽粒破碎率存在显著差异,抗破碎特性是可遗传的性状,可通过育种培育抗破碎率的品种;不同收获机械和作业参数对籽粒破碎率有显著影响,选择轴流式收获机,并根据玉米生长、成熟和籽粒含水率状况及时检查与调试收获机参数是保证低破碎率的有效措施;生态环境因素对破碎率也有显著的影响,籽粒形成、自然干燥和收获期的光照、温度、湿度等因素均会影响到籽粒硬度、容重、含水率和质地等与籽粒破碎相关的特性;种植密度、水肥管理、收获时期等栽培管理措施对籽粒破碎率也会产生明显的影响。因此,针对不同区域生态环境条件,应选择适宜生育期内能与当地光温资源匹配的品种以及确定品种适宜的种植区域。合理种植密度、优化氮肥管理和适量灌溉有利于降低破碎率,而选择在最佳收获期收获是降低籽粒破碎率的最有效措施。 |
[5] | . . |
[6] | . 【目的】机械粒收是玉米生产的发展方向,收获质量是影响其推广应用的主要因素。中国玉米机械粒收还处于起步阶段,目前在西北和东北等春播玉米区推广应用面积较大,黄淮海夏播玉米区正在积极开展试验示范。本研究通过分析黄淮海夏玉米机械粒收质量及其影响因素,为该技术的推广应用提供支持。【方法】2013—2015年累计选用了23个玉米品种,在黄淮海典型代表区河南新乡开展试验研究。2013年和2015年在收获期分别进行2次机械收获,2014年1次机械收获。收获当天测定各个品种的收获前籽粒含水率,并调查测产。机械收获后从机仓随机取一定量籽粒样品,立即测定收获后籽粒含水率,然后手工分拣样品,测定籽粒破碎率和杂质率;收获后,在田间选取3个代表性样区,调查落穗损失和落粒损失。【结果】2013—2015年,籽粒破碎率共调查131个样点,结果显示,收获时玉米籽粒含水率在20.80%—41.08%,籽粒破碎率变幅为4.98%—41.36%,籽粒破碎率随着籽粒含水率的提高明显升高;破碎率低于8%的有38个样点,占比29.01%,籽粒含水率低于26.92%时,收获的玉米籽粒能够满足破碎率8%以下的要求。机收杂质率共调查134个样点,杂质率0.37%—5.28%,杂质率低于3%的样点有107个,占比79.85%,杂质率也随着籽粒含水率的升高而增加;2013—2014年,籽粒含水率低于28.27%时,杂质率能够低于3%的国家标准;2015年收获时籽粒含水率虽然较高,但杂质率均在3%以下。田间损失率共调查108个样点,变幅为0.18%—2.85%(落穗率和落粒率),均能满足国家标准,损失率不是影响机械收获质量的限制因素。在本试验条件下,籽粒含水率低于26.92%时,破碎率和杂质率分别低于8%和3%,田间损失率也符合国家标准,能够满足机械粒收质量要求。研究还发现,籽粒含水率相近的不同品种之间,机械收获的破碎率和杂质率也存在显著差异,17 . 【目的】机械粒收是玉米生产的发展方向,收获质量是影响其推广应用的主要因素。中国玉米机械粒收还处于起步阶段,目前在西北和东北等春播玉米区推广应用面积较大,黄淮海夏播玉米区正在积极开展试验示范。本研究通过分析黄淮海夏玉米机械粒收质量及其影响因素,为该技术的推广应用提供支持。【方法】2013—2015年累计选用了23个玉米品种,在黄淮海典型代表区河南新乡开展试验研究。2013年和2015年在收获期分别进行2次机械收获,2014年1次机械收获。收获当天测定各个品种的收获前籽粒含水率,并调查测产。机械收获后从机仓随机取一定量籽粒样品,立即测定收获后籽粒含水率,然后手工分拣样品,测定籽粒破碎率和杂质率;收获后,在田间选取3个代表性样区,调查落穗损失和落粒损失。【结果】2013—2015年,籽粒破碎率共调查131个样点,结果显示,收获时玉米籽粒含水率在20.80%—41.08%,籽粒破碎率变幅为4.98%—41.36%,籽粒破碎率随着籽粒含水率的提高明显升高;破碎率低于8%的有38个样点,占比29.01%,籽粒含水率低于26.92%时,收获的玉米籽粒能够满足破碎率8%以下的要求。机收杂质率共调查134个样点,杂质率0.37%—5.28%,杂质率低于3%的样点有107个,占比79.85%,杂质率也随着籽粒含水率的升高而增加;2013—2014年,籽粒含水率低于28.27%时,杂质率能够低于3%的国家标准;2015年收获时籽粒含水率虽然较高,但杂质率均在3%以下。田间损失率共调查108个样点,变幅为0.18%—2.85%(落穗率和落粒率),均能满足国家标准,损失率不是影响机械收获质量的限制因素。在本试验条件下,籽粒含水率低于26.92%时,破碎率和杂质率分别低于8%和3%,田间损失率也符合国家标准,能够满足机械粒收质量要求。研究还发现,籽粒含水率相近的不同品种之间,机械收获的破碎率和杂质率也存在显著差异,17 |
[7] | |
[8] | . The seeds and embryos of Zea mays L. cv. Yuedan 9117 were used to determine the acquisition of desiccation tolerance and germinability during maize seed development. Physiological maturity of seeds was at 42 days after pollination (DAP). The embryos obtained germinability during 14-21 DAP, and the desiccation tolerance of embryos was obtained during 25-28 DAP, desiccation tolerance still being strengthened after 28 DAP. The changes in electrolyte leakage rate of seeds and embryos during development of maize seeds might suggest that there was a close relationship among desiccation tolerance,membran e development,and protection of membrane injury.Desiccation is beneficial to germ ination of seeds and embryos during the development of maize. The seeds and embryos of Zea mays L. cv. Yuedan 9117 were used to determine the acquisition of desiccation tolerance and germinability during maize seed development. Physiological maturity of seeds was at 42 days after pollination (DAP). The embryos obtained germinability during 14-21 DAP, and the desiccation tolerance of embryos was obtained during 25-28 DAP, desiccation tolerance still being strengthened after 28 DAP. The changes in electrolyte leakage rate of seeds and embryos during development of maize seeds might suggest that there was a close relationship among desiccation tolerance,membran e development,and protection of membrane injury.Desiccation is beneficial to germ ination of seeds and embryos during the development of maize. |
[9] | . |
[10] | . Dry-matter and water content of kernels, rachii and ears of maize ( Zea mays L.) cultivar Pioneer 3901 were measured during the period from silking to harvest maturity in order to (a) determine when net loss of water from the kernels and ear commenced, (b) investigate the use of ear moisture-content (% wet-weight basis) as a scale for kernel development during grain-filling, and (c) use this scale to investigate the relationships between ear moisture, physiological maturity and the environment. Water loss occurred in two phases. The first phase, ending at physiological maturity, had a constant rate of water loss, and was interpreted as a 鈥榙evelopmental鈥 loss of water associated with grain-filling. The second phase, which commenced at physiological maturity, showed a falling rate of water loss characteristic of the drying process. This pattern of water loss was consistent for 17 other genotypes studied in less detail, with maximum water content of the ear occurring between 80 and 60% ear moisture in all cases. Below 70% ear moisture, dry-weight per kernel increased linearly with decreasing ear moisture, enabling a direct and precise estimate to be made of ear moisture-content at the onset of physiological maturity. This method of analysis proved to be useful for evaluating the influence of the environment on grain-filling in a single cultivar. The relationship between dry-weight per kernel and ear moisture was consistent for ten crops of Pioneer 3901 studied over five seasons, but the stage at which grain-filling ceased varied. In six unstressed crops, physiological maturity was attained at between 41 and 43% ear moisture. In other crops, where cessation of grain-filling was associated with the occurence of air frosts, physiological maturity was attained at ear moistures ranging from 45 to 55%. Ear moisture at physiological maturity was also influenced by genotype, with values ranging from 34.3 to 44.5% for the 18 genotypes studied. Differences in ear moisture at physiological maturity among 12 of these genotypes were associated with different absolute levels of water in the ear during grain-filling. Strategies for lowering grain moisture at harvest through breeding should therefore take account of the absolute levels of water in the ear, as well as the more usual expression of moisture content on a wet-weight basis. |
[11] | . 实验选用早、中、晚三个玉米品种,研究夏玉米籽粒灌浆与籽粒含水率的关系,结果表明,灌浆速度曲线并不随平均气温的波动而变化,不同品种、不同播期玉米灌浆速度峰值的出现以及灌浆的终止等,与籽粒含水率的关系较为稳定,不同品种、不同播期玉米灌浆速度与籽粒含水率的关系曲线均呈“单峰曲线”。根据含水率与灌浆的关系,提出了玉米籽粒灌浆新的分期方法。 . 实验选用早、中、晚三个玉米品种,研究夏玉米籽粒灌浆与籽粒含水率的关系,结果表明,灌浆速度曲线并不随平均气温的波动而变化,不同品种、不同播期玉米灌浆速度峰值的出现以及灌浆的终止等,与籽粒含水率的关系较为稳定,不同品种、不同播期玉米灌浆速度与籽粒含水率的关系曲线均呈“单峰曲线”。根据含水率与灌浆的关系,提出了玉米籽粒灌浆新的分期方法。 |
[12] | . Synopsis: Husk and shank characteristics and shape or size of ear were not found to be major factors associated with differing rates of drying among strains of corn. |
[13] | . |
[14] | . . |
[15] | . . |
[16] | . . |
[17] | |
[18] | . . |
[19] | . Two field experiments were conducted near Guelph, Ontario in both 1969 and 1970 with the objectives of (i) studying the relationship between grain moisture percentage and black layer development and (ii) examining the effect of environment on the length of the interval from planting or silking to black layer formation in corn (Zea mays L.). The first experiment involved 10 adapted commercial hybrids planted in early May at a plant population of 70,000 plants/ha. The second study concerned three commercial hybrids that were planted at a plant population of 55,000 plants/ha on each of four dates in 1969 (May 14 and 23, and June 5 and 13) and four dates in 1970 (May 6 and 20, and June 1 and 8). For both experiments records or measurements were taken of the date of 50% silking, 50% black layer, grain moisture percentage at 50% black layer, and final yield. Weather records were used to compute the number of accumulated heat units, by either the Ontario Corn Heat Unit (OCHU) or the 10- to 30-C Growing Degree Day (GDDHU) systems, from planting or midsilking to 50% black layer. |
[20] | . Comparisons of growth analysis functions within and among experiments are often confounded by sources of variation other than those imposed by treatment. we suggest use of a temperature index, such as modified growing degree days, as the divisor in growth functions to facilitate treatment comparisons within certain experiments and to reduce the effects of differing temperature regimes among experiments on these comparisons. Three experiments were identified to provide data to analyze this new approach. Mean absolute growth rate (GR) and mean relative growth rate (RGR) were compared in two experiments with maize (Zea mays L.) conducted in eastern Nebraska. Previously published values of RGR and mean net assimilation rate (NAR) of barley (Hordeum vulgare L.) grown under controlled environments in a soil temperature and P fertility study were also evaluated. Use of modified growing degree days, rather than days, as the divisor in these growth functions led to the recognition of physiological differences due to or associated with treatment, which were previously masked by normal crop response to temperature, and clarified other treatment differences by reducing the effect of temperature. |
[21] | . In an attempt to find better ways of estimating relative maturity differences in corn (L.), various thermal unit formulas were evaluated. Utilizing data from six plantings of corn over a 2 year period, 22 different methods of computing thermal units were tested for their ability to account for variation in flowering dates. Both hourly and daily temperature data were used in the equations. In general the daily measurements appeared to be approximately as accurate as the hourly measurements. The best equation for predicting flowering dates on the basis of thermal units utilized a base temperature of 10C (50F) and an optimum of 30C (86F). The excess temperature above 30C was subtracted to account for high temperature stress. |
[22] | |
[23] | . 基于隆平华研种业的育种实践和农业部关于籽粒机收玉米的现行标准,提出了机收净籽粒和机收干籽粒的品种标准,机收净籽粒和干籽粒的水分分别控制在20%和16%以内,破碎率控制在3%和1%以内。并阐述了实现这个育种目标的杂交模式。通过试验结果的分析,认为早熟和脱水快是机收玉米的首要核心性状,抗倒抗病是机收玉米的必要支持性状;实现机收丰产的主要途径是提高玉米种植密度。 . 基于隆平华研种业的育种实践和农业部关于籽粒机收玉米的现行标准,提出了机收净籽粒和机收干籽粒的品种标准,机收净籽粒和干籽粒的水分分别控制在20%和16%以内,破碎率控制在3%和1%以内。并阐述了实现这个育种目标的杂交模式。通过试验结果的分析,认为早熟和脱水快是机收玉米的首要核心性状,抗倒抗病是机收玉米的必要支持性状;实现机收丰产的主要途径是提高玉米种植密度。 |
[24] | . Assimilate supply (source) reportedly limits grain yields of early-maturing maize (Zea mays L.) hybrids. We studied combining abilities of S2 lines with extremes in average leaf expansion rate (ALER) values to test for genetically correlated responses for grain yield, ear moisture, stalk lodging, yield components, and yield stability parameters. The S2 hybrids were selected to represent a 2 X 2 factorial arrangement of ALER types (HER = high and LER = low ALER). Two groups of lines (GI and GII) were selected from S2 lines previously rated using the formula ALER = (leaf number X length X width X 0.75)/(d to pollination). Four sets of lines with extremes for ALER ratings were paired by source population, year of evaluation, and silking date. Within each set, the four S2 lines from GI were crossed to the four S2 lines from GII to produce 16 hybrids representing a 2 X 2 factorial arrangement of four hybrids each of HER X HER, HER X LER, LER X HER, and LER X LER classes. Maize hybrids were grown in field experiments arranged in simple lattice designs with two replicates in seven environments. Across all environments, hybrids from HER parents yielded 8.5 and 6.9% more than LER hybrids for GI and GII, respectively. For both GI and GII, the HER hybrids produced kernel weights 0.06 g heavier than LER hybrids. The HER parents produced hybrids with ears 33 g kg-1 lower in moisture at harvest in GI. Our data support the theory that source limits yield of early maize. We suggest that selecting for HER should be a simple, effective means of increasing the source without increasing ear moisture at harvest. |
[25] | . Moisture data for 12 years from the period 1940 to 1963 were summarized to determine the relation between kernel moisture and time. The rate of kernel moisture reduction was determined for five arbitrary moisture phases and used to predict when specified moisture levels would be attained from pollination date. Correlation studies were made between rate of kernel moisture reduction and four weather factors (air temperature, saturation deficit, wet bulb depression, and relative humidity). |
[26] | . . |
[27] | . Phenological development of corn was studied under field conditions at several locations throughout southwestern Manitoba. Data for 12 location-years were collected from eight sites during 1980–1983. Two early-maturing hybrids (‘Pioneer 3995’ and ‘Northrup King 403’) and a medium maturity hybrid (‘Pride 1108’) were used. A temperature response function that accurately described phenological development under a controlled environment was modified and used to estimate phenological development of field-grown corn. The iterative temperature (IT) model allowed more accurate estimation of the duration from emergence to stem elongation and from stem elongation to silking than corn heat unit (CHU) or growing degree day (GDD) models. The IT model was independent of growth phase duration; this was not the case for CHU and GDD. The model is not suited to hybrid maturity rating, but may be useful for simulation modeling purposes. |
[28] | . Abstract Thermal indices predict and describe development rate more accurately than time in days and are commonly used to rate maize (Zea mays L.) for maturity. Separate temperature response functions for the vegetative and grain-filling periods predict more accurately time to maturity than a single function for the two periods combined. However, use of two functions requires a priori knowledge of the silking date, which becomes the transition date from the vegetative function to the grain-filling function. The objective of this study was to evaluate the sensitivity of estimates of silking and maturity dates to the transition date between vegetative and grain-filling functions and to develop a protocol to combine the two temperature response functions in a general thermal index (GTI) for maize. Frequency distributions of mean daily air temperatures for five 20-d periods spanning mid-June to late September at 19 locations in the northern USA and southern Ontario from 1992 to 1995 indicated few days (鈮12%) with mean daily air temperatures less than 15掳C before late August. This was significant, as the two response functions diverged significantly at temperatures below 15掳C. Standard errors in estimating maturity date using different transition dates remained small (<7.5 d) unless the transition date was delayed beyond the first week of September. Based on this analysis, a standard transition date of 1 August was proposed for the GTI. Testing on an independent data set indicated that the GTI and a transition date of 1 August provided more accurate estimates of the planting to maturity period than growing degree days (GDD) or crop heat units (CHU), with a standard error of 8.2 d (compared with 14.5 d using GDD and 12.5 d using CHU). |
[29] | . . |
[30] | . Kernel water relations play a key role in controlling the duration of grain filling. This duration is controlled by the relationship between kernel water and biomass development, as it determines the timing kernels reach a critical percent moisture content (MC, measured on a fresh weight basis) at which biomass accumulation stops. The time in which this critical percent MC is attained can be affected by the timing kernel net water uptake stops (i.e. maximum water content is reached), or by the relationship between water loss and biomass deposition after maximum water content is attained. Which of the two mechanisms could be behind genotypic differences in maize ( Zea mays L.) grain-filling duration was unknown. We also studied the relationship between kernel water and volume development, as it was unknown in this species. Thirteen commercial hybrids were evaluated under different growing environments, and weight, water content and volume of their kernels were measured throughout grain filling. There were no differences among hybrids in their kernel percent MC at physiological maturity ( p > 0.05), showing that hybrid differences in grain-filling duration (from 1117 to 1470 掳C day) were related to variations in the accumulated thermal time from flowering to this critical percent MC. There were no differences in the accumulated thermal time from silking to kernel maximum water content, and this stage was always reached at the same kernel percent MC (ca. 540 g kg 鈭1). Differences in grain-filling duration were explained by the pattern of percent MC decline after maximum water content was reached. This percent MC decline was dependent upon the relationship between water loss and biomass deposition; the higher the water loss rate and the higher the kernel growth rate the shorter the duration ( r 2 = 0.60; p < 0.001). Maximum kernel volume was achieved after maximum water content, and close to physiological maturity. Hybrids differed ( p < 0.05) in the kernel volume generated after maximum water content, and this was also related to the relationship between biomass and water development late in grain filling. Results showed the importance of understanding and predicting percent MC development throughout grain filling, as there were no differences between hybrids and environments in their kernel percent MC at specific developmental stages (i.e. maximum water content or physiological maturity). Our results highlighted the importance of the relationship between water loss and biomass deposition during late kernel development in the duration of maize grain filling. |
[31] | . High-oil hybrids having the same date of anthesis as normal-oil hybrids are frequently found to have higher moisture content in the grain at harvest time. Field drying rate of maize (Zea mays L.) grain was followed in nine single-cross hybrids differing in percent oil in the kernel and in agronomic traits. The objectives of this study were to determine the duration of the grain filling period (GFP), moisture content at physiological maturity, and grain moisture loss throughout the period after physiological maturity. Hybrids were grown in 1984 in a randomized complete-block design with four replications at Urbana, IL, and Zemun Polje, Yugoslavia. Three ears were harvested from each plot 14 d after pollination (DAP) and at weekly intervals until 70 DAP. Percent moisture in the grain, and 100-kernel weight were measured for each hybrid, harvest date, and location. High-oil hybrids had higher moisture content 70 DAP than did normal-oil hybrids. Differences in kernel moisture percent among oil groups at the beginning of GFP were not significant at Zemun Polje, but were significant at Urbana. At the time of approximate physiological maturity the 7% oil group had significantly lower moisture percent in the grain than did the normal- and 9% oil hybrids at both locations, and had significantly lower moisture percent in the grain than the 9% oil hybrids at Zemnn Polje only. There were no significant differences in rate of kernel water loss among oil groups throughout the GFP. However, normal-oil hybrids were faster drying than 7 and 9% oil hybrids after physiological maturity. Differences in the drying down rate between the 7 and 9% oil groups were not significant at one (Urbana) of the two locations. |
[32] | . . |
[33] | Resistance was evaluated in [], [] and var. [subsp. ], an agrotriticum with 56 chromosomes and 8 species. Both of the species and the agrotriticum showed high resistance to []. Most species were highly susceptible, and it is suggested that chromosome substitution would have to be used to obtain resistance. |
[34] | . 为选育脱水速率快的玉米新品 种,以黑龙江省10个熟期相近而脱水速率差异较大的优良玉米自交系为试验材料,采用完全双列杂交设计配置杂交组合,对玉米的百粒重、穗粗等12个农艺性状 与玉米生理成熟后籽粒脱水速率进行遗传相关和通径分析。结果表明:穗粗、穗行数、粒宽和胚重/胚乳重与玉米生理成熟后籽粒脱水速率之间均表现为显著或极显 著正向相关;百粒重、穗长、胚占籽粒体积比和果皮厚度与玉米生理成熟后籽粒脱水速率之间均表现为极显著负向相关。通径分析结果表明:穗粗、穗行数、粒宽和 胚重/胚乳重与玉米生理成熟后籽粒脱水速率直接通径系数为正值,百粒重、穗长、胚占籽粒体积比和果皮厚度与玉米生理成熟后籽粒脱水速率直接通径系数为负 值。为获取脱水速率快的玉米,应主要选育果穗短粗、籽粒宽度较大、果皮薄和百粒重小的基因型的玉米杂交种。 . 为选育脱水速率快的玉米新品 种,以黑龙江省10个熟期相近而脱水速率差异较大的优良玉米自交系为试验材料,采用完全双列杂交设计配置杂交组合,对玉米的百粒重、穗粗等12个农艺性状 与玉米生理成熟后籽粒脱水速率进行遗传相关和通径分析。结果表明:穗粗、穗行数、粒宽和胚重/胚乳重与玉米生理成熟后籽粒脱水速率之间均表现为显著或极显 著正向相关;百粒重、穗长、胚占籽粒体积比和果皮厚度与玉米生理成熟后籽粒脱水速率之间均表现为极显著负向相关。通径分析结果表明:穗粗、穗行数、粒宽和 胚重/胚乳重与玉米生理成熟后籽粒脱水速率直接通径系数为正值,百粒重、穗长、胚占籽粒体积比和果皮厚度与玉米生理成熟后籽粒脱水速率直接通径系数为负 值。为获取脱水速率快的玉米,应主要选育果穗短粗、籽粒宽度较大、果皮薄和百粒重小的基因型的玉米杂交种。 |
[35] | . 为明确夏玉米生理成熟期子粒含水率及其影响因素,2014-2016年,以郑单958、先玉335等玉米品种为研究对象,分别在北京和河南新乡开展品种比较和播期研究.结果表明:生理成熟期玉米子粒含水率平均27.8%,在品种间存在极显著差异,变幅为21.5%~33.1%,按80%置信区间为24.2%~31.4%;环境条件对子粒达到生理成熟的时间和含水率有极显著影响,且环境和品种之间具有明显的交互作用;生理成熟期子粒含水率高低与授粉到生理成熟经历的天数之间相关度较低.夏玉米区主栽品种郑单958、先玉335、农华101、中单909、京农科728、华美1号和农华816生理成熟期子粒含水率平均值分别为28.4%、24.9%、27.9%、29.1%、28.7%、29.2%和29.9%. . 为明确夏玉米生理成熟期子粒含水率及其影响因素,2014-2016年,以郑单958、先玉335等玉米品种为研究对象,分别在北京和河南新乡开展品种比较和播期研究.结果表明:生理成熟期玉米子粒含水率平均27.8%,在品种间存在极显著差异,变幅为21.5%~33.1%,按80%置信区间为24.2%~31.4%;环境条件对子粒达到生理成熟的时间和含水率有极显著影响,且环境和品种之间具有明显的交互作用;生理成熟期子粒含水率高低与授粉到生理成熟经历的天数之间相关度较低.夏玉米区主栽品种郑单958、先玉335、农华101、中单909、京农科728、华美1号和农华816生理成熟期子粒含水率平均值分别为28.4%、24.9%、27.9%、29.1%、28.7%、29.2%和29.9%. |
[36] | . Grain weight of maize is determined by the sink establishment, grain filling rate and filling duration. Environmental and cultivating factors (such as temperature, water, fertilizer and plant growth regulators) can influence such process by regulating the carbohydrate metabolism and hormone level in the plants. The key point of further study on grain weight was discussed. . Grain weight of maize is determined by the sink establishment, grain filling rate and filling duration. Environmental and cultivating factors (such as temperature, water, fertilizer and plant growth regulators) can influence such process by regulating the carbohydrate metabolism and hormone level in the plants. The key point of further study on grain weight was discussed. |
[37] | . 【Objective】It is very important to study photosynthesis of super high-yielding maize hybrids, so a field trail was conducted to research the relation to photosynthetic traits and yield of over-15000 kg ha-1 summer maize hybrids during grain filling period. 【Method】Three summer maize hybrids (XY335, DH3632 and DH3806) were planted at 78000 plants ha-1 in National Corn Project Technology Research Center (Shandong) randomly. Above-ground biomass partitioning and photosynthetic characteristics of ear leaves were investigated to evaluate yield formation of three super high-yielding maize hybrids during grain filling period.【Result】Yields of three-type maize hybrids were over 15000 kg ha-1, and yield of XY335 was higher than that of DH3632 and DH3806 significantly (P<0.05). Characteristic of grain filling analyzed by Richards equation showed XY335 had the higher grain-filling rate, the longer active growing period, and it reached the maximum grain-filling rate earlier than DH3632 and DH3806. The result indicated grain-filling traits like XY335 was favorable to high yield in the experiment. The leaves’ photosynthetic physiology quantity of XY335 was highest of the three-type hybrids. XY335 had high net photosynthetic rate (Pn), PEPCase activity, RuBPCase activity and chlorophyll a/b value after anthesis, and the leaf area index (LAI) and soluble protein content decreased slowly from 20d and 30d after flowering, respectively. 【Conclusion】To obtain 15000 kg ha-1 of super high-yielding breeding and cultivation in practice, we need to improve the leaves photosynthetic physiology quantity to maintain high grain-filling rate and long active growing period after anthesis, enhance the solar energy use efficiency. . 【Objective】It is very important to study photosynthesis of super high-yielding maize hybrids, so a field trail was conducted to research the relation to photosynthetic traits and yield of over-15000 kg ha-1 summer maize hybrids during grain filling period. 【Method】Three summer maize hybrids (XY335, DH3632 and DH3806) were planted at 78000 plants ha-1 in National Corn Project Technology Research Center (Shandong) randomly. Above-ground biomass partitioning and photosynthetic characteristics of ear leaves were investigated to evaluate yield formation of three super high-yielding maize hybrids during grain filling period.【Result】Yields of three-type maize hybrids were over 15000 kg ha-1, and yield of XY335 was higher than that of DH3632 and DH3806 significantly (P<0.05). Characteristic of grain filling analyzed by Richards equation showed XY335 had the higher grain-filling rate, the longer active growing period, and it reached the maximum grain-filling rate earlier than DH3632 and DH3806. The result indicated grain-filling traits like XY335 was favorable to high yield in the experiment. The leaves’ photosynthetic physiology quantity of XY335 was highest of the three-type hybrids. XY335 had high net photosynthetic rate (Pn), PEPCase activity, RuBPCase activity and chlorophyll a/b value after anthesis, and the leaf area index (LAI) and soluble protein content decreased slowly from 20d and 30d after flowering, respectively. 【Conclusion】To obtain 15000 kg ha-1 of super high-yielding breeding and cultivation in practice, we need to improve the leaves photosynthetic physiology quantity to maintain high grain-filling rate and long active growing period after anthesis, enhance the solar energy use efficiency. |