彭孝天1,2,冯诗愚1,2,陈晨1,2,张瑞华1,2,潘俊3,王洋洋3
(1.南京航空航天大学 航空学院,南京 210016;2.飞行器环境控制与生命保障工业和信息化部重点实验室(南京航空航天大学),南京 210016;3.航空机电系统综合航空科技重点实验室(南京机电液压工程研究中心),南京 211106)
摘要:
了解析水及气体溶解逸出对新型催化惰化系统性能影响,可以为系统设计提供借鉴.首先,设计了低温可控耗氧催化惰化系统流程,然后,以油箱中抽吸气体的摩尔流量为基准,推导了流经催化反应器、冷却器前后各气体组分的流量关系,并通过状态方程及气体平衡溶解关系,确定油箱气相空间各气体浓度变化. 另外,在燃油中有、无气体溶解逸出情况下,研究了风机流量、载油率两个关键参数对系统中产水及冷却性能的影响. 研究结果表明:油箱气相空间的氧浓度随惰化的进行逐渐降低,水蒸气摩尔分数随惰化时间逐渐增加但增长速率逐渐变缓;催化反应器和冷却器所需的冷却功率、催化反应器出口气体的相对湿度、冷却器中析出的液态水量均随时间逐渐降低;另外,燃油中氧气、氮气、二氧化碳等气体的溶解逸出对系统性能影响较大,并且考虑水析出时,冷却器中所需的冷却气体量较不考虑水析出时要高约33%. 因此今后在设计催化惰化系统时,燃油中气体的溶解逸出及液态水析出对惰化系统性能影响均不容忽略.
关键词: 惰化 催化燃烧 航空燃油 析水 溶解逸出
DOI:10.11918/201909084
分类号:V37
文献标识码:A
基金项目:国家自然科学基金民航联合基金(U1933121);江苏省研究生科研与实践创新计划项目(KYCX19_0198);南京航空航天大学研究生拔尖创新人才培养“引航计划”跨学科创新基金项目(KXKCXJJ202004)
Effect of water precipitation and gas dissolution and escape on performance of catalytic inerting system
PENG Xiaotian1,2,FENG Shiyu1,2,CHEN Chen1,2,ZHANG Ruihua1,2,PAN Jun3,WANG Yangyang3
(1.College of Aerospace Engineering, Nanjing University of Aeronautics and Astronautics, Nanjing 210016, China; 2. Key Laboratory of Aircraft Environmental Control and Life Support (Nanjing University of Aeronautics and Astronautics), Ministry of Industry and Information Technology, Nanjing 210016, China; 3. Aviation Key Laboratory of Science and Technology on Aero Electromechanical System Integration (Nanjing Engineering Institute of Aircraft Systems), Nanjing 211106, China)
Abstract:
The understanding of the influence of water and gas dissolution and escape on the performance of a new type of catalytic inerting system can provide guidance for the design of the system. First, a low temperature controllable oxygen consumed catalytic inerting system process was designed. Then, based on the molar flow rate of the pumped gas in the fuel tank, the flow relationship of each gas component before and after flowing through the catalytic reactor and the cooler was deduced, and the concentration variation of each gas in the gas phase space of the fuel tank was determined through the gas state equation and the gas equilibrium dissolution relationship. In addition, in the cases of fuel with or without gas dissolution and escape, the effect of two key parameters, i.e., flow rate and oil loading rate of fan, on the water production and cooling performance of the system was studied. Results show that the oxygen concentration in the gas phase space of the tank decreased with the inerting process, and the molar fraction of the water vapor increased while the growth rate gradually slowed down. The cooling power required in the reactor and the cooler, the relative humidity at the outlet of the reactor, and the water removal in the cooler all decreased with time. Besides, the dissolved and escaped gases such as oxygen, nitrogen, and carbon dioxide in the fuel had a great impact on the system performance, and the amount of cooling gas required in the cooler was about 33% higher when water precipitation was taken into account. Therefore, the influence of gas dissolution and escape and water precipitation on the performance of the inerting system should not be neglected in the design of catalytic inerting systems in the future.
Key words: inerting catalytic combustion aviation fuel water precipitation gas dissolution and escape
彭孝天, 冯诗愚, 陈晨, 张瑞华, 潘俊, 王洋洋. 析水及溶解逸出对催化惰化系统性能影响[J]. 哈尔滨工业大学学报, 2021, 53(6): 71-76. DOI: 10.11918/201909084.

PENG Xiaotian, FENG Shiyu, CHEN Chen, ZHANG Ruihua, PAN Jun, WANG Yangyang. Effect of water precipitation and gas dissolution and escape on performance of catalytic inerting system[J]. Journal of Harbin Institute of Technology, 2021, 53(6): 71-76. DOI: 10.11918/201909084.

基金项目 国家自然科学基金民航联合基金(U1933121);江苏省研究生科研与实践创新计划项目(KYCX19_0198);南京航空航天大学研究生拔尖创新人才培养“引航计划”跨学科创新基金项目(KXKCXJJ202004) 作者简介 彭孝天(1993—),男,博士研究生 通信作者 冯诗愚,shiyuf@nuaa.edu.cn 文章历史 收稿日期: 2019-09-10
Abstract Full text Figures/Tables PDF
析水及溶解逸出对催化惰化系统性能影响
彭孝天1,2, 冯诗愚1,2


1. 南京航空航天大学 航空学院, 南京 210016;
2. 飞行器环境控制与生命保障工业和信息化部重点实验室(南京航空航天;
3. 航空机电系统综合航空科技重点实验室(南京机电液压工程研究中心),南京 211106)
收稿日期: 2019-09-10
基金项目: 国家自然科学基金民航联合基金(U1933121);江苏省研究生科研与实践创新计划项目(KYCX19_0198);南京航空航天大学研究生拔尖创新人才培养“引航计划”跨学科创新基金项目(KXKCXJJ202004)
作者简介: 彭孝天(1993—),男,博士研究生
通信作者: 冯诗愚,shiyuf@nuaa.edu.cn
摘要: 了解析水及气体溶解逸出对新型催化惰化系统性能影响,可以为系统设计提供借鉴.首先,设计了低温可控耗氧催化惰化系统流程,然后,以油箱中抽吸气体的摩尔流量为基准,推导了流经催化反应器、冷却器前后各气体组分的流量关系,并通过状态方程及气体平衡溶解关系,确定油箱气相空间各气体浓度变化.另外,在燃油中有、无气体溶解逸出情况下,研究了风机流量、载油率两个关键参数对系统中产水及冷却性能的影响.研究结果表明:油箱气相空间的氧浓度随惰化的进行逐渐降低,水蒸气摩尔分数随惰化时间逐渐增加但增长速率逐渐变缓;催化反应器和冷却器所需的冷却功率、催化反应器出口气体的相对湿度、冷却器中析出的液态水量均随时间逐渐降低;另外,燃油中氧气、氮气、二氧化碳等气体的溶解逸出对系统性能影响较大,并且考虑水析出时,冷却器中所需的冷却气体量较不考虑水析出时要高约33%.因此今后在设计催化惰化系统时,燃油中气体的溶解逸出及液态水析出对惰化系统性能影响均不容忽略.
关键词: 惰化 催化燃烧 航空燃油 析水 溶解逸出
Effect of water precipitation and gas dissolution and escape on performance of catalytic inerting system
PENG Xiaotian1,2, FENG Shiyu1,2


1. College of Aerospace Engineering, Nanjing University of Aeronautics and Astronautics, Nanjing 210016, China;
2. Key Laboratory of Aircraft Environmental Control and Life Support (Nanjing University of Aeronautics and Astronautics), Ministry of Industry and Information Technology, Nanjing 210016, China;
3. Aviation Key Laboratory of Science and Technology on Aero Electromechanical System Integration (Nanjing Engineering Institute of Aircraft Systems), Nanjing 211106, China)
Abstract: The understanding of the influence of water and gas dissolution and escape on the performance of a new type of catalytic inerting system can provide guidance for the design of the system. First, a low temperature controllable oxygen consumed catalytic inerting system process was designed. Then, based on the molar flow rate of the pumped gas in the fuel tank, the flow relationship of each gas component before and after flowing through the catalytic reactor and the cooler was deduced, and the concentration variation of each gas in the gas phase space of the fuel tank was determined through the gas state equation and the gas equilibrium dissolution relationship. In addition, in the cases of fuel with or without gas dissolution and escape, the effect of two key parameters, i.e., flow rate and oil loading rate of fan, on the water production and cooling performance of the system was studied. Results show that the oxygen concentration in the gas phase space of the tank decreased with the inerting process, and the molar fraction of the water vapor increased while the growth rate gradually slowed down. The cooling power required in the reactor and the cooler, the relative humidity at the outlet of the reactor, and the water removal in the cooler all decreased with time. Besides, the dissolved and escaped gases such as oxygen, nitrogen, and carbon dioxide in the fuel had a great impact on the system performance, and the amount of cooling gas required in the cooler was about 33% higher when water precipitation was taken into account. Therefore, the influence of gas dissolution and escape and water precipitation on the performance of the inerting system should not be neglected in the design of catalytic inerting systems in the future.
Keywords: inerting catalytic combustion aviation fuel water precipitation gas dissolution and escape
近50年来,全球范围内运输类飞机燃油箱爆炸事故共发生18起,总计542人遇难,已成为民用航空安全的主要威胁之一[1-2].美国联邦航空局(Federal aeronautics administration,FAA)和国家运输安全部(National transportation safety board,NTSB)均认为采用燃油箱惰化技术是一种可行的措施来降低油箱的燃爆风险[3-4].目前,中空纤维膜机载制氮惰化技术(Hollow fiber membrane on-board inert gas generation system, HFM-OBIGGS)是飞机上应用最广的制取富氮气体的油箱防爆技术,在波音和空客的各型飞机及国内的C919和新舟700等机型中均使用了该技术[5-6].但从国内外应用现状来看,HFM-OBIGGS技术仍存在分离膜效率低、入口需求压力高、污染环境等问题[7].
相关科研机构和厂商一直致力于研究新型燃油箱防爆技术[8-9],以克服HFM-OBIGGS的不足,而耗氧型燃油箱惰化技术具有流程简单、效率高等优点,近年来被认为是最可能替代HFM-OBIGGS的下一代惰化方式[10-11],该系统不需要使用发动机引气,系统除一个低压泵外,无任何运动部件,因此功耗低、结构紧凑,质量轻.该系统不但能够解决HFM-OBIGGS存在的需要发动机引气的问题,而且不像纤维膜式系统一样排放废气,因而被称作“绿色机载惰性气体发生系统”(Green on-board inert gas generation system, GOBIGGS)[12-13].
鉴于GOBIGGS明显的技术优势和诱人的应用前景,本课题组在国内率先展开随该技术的追踪与研究[14-15],并结合国产航空燃油饱和蒸汽压高的特点[16-17],提出了一种低温可控耗氧催化惰化技术(3CIS-low temperature Controllable oxygen Consumed Catalytic Inerting System).经过4年多的研究,目前在3CIS需求分析、系统构架和权衡,特别是催化剂方面已取得一定成果.但是仍然存在大量问题亟待解决,如与HFM-OBIGGS不同的是,在3CIS系统中,催化反应器中反应会产生大量的水,必须将混合惰气充分干燥,否则水蒸气随惰气流回油箱,将与温度较低的油箱壁面接触,冷却析出,进一步导致滋生微生物、改变燃油物性等问题,最终影响燃油在发动机中的燃烧.
了解3CIS中反应器后的析水量及油箱内的水含量对除水部件的设计及系统性能的评价至关重要,而目前的研究中均忽略或未重点考虑该问题[18].除此之外,与HFM-OBIGGS相不同的是,3CIS系统需要提供冷源来冷却催化反应器,反应后的高温气体在冷却器中也需要被冷却降温.所以,得到不同惰化条件下,催化反应器和冷却器中所需要的冷却功率,可为系统设计提供理论支撑[19-20].因此,本文建立了3CIS数学模型,研究系统的产水及冷却性能,并分析关键参数变化对其产生的影响.
1 惰化系统原理及假设图 1为3CIS流程图,系统中主要参数关系及文中参数意义均在图 1中表示,系统基本原理为:飞机燃油箱上部的油气混合物在风机的抽吸作用下,流入电加热器中被预热,然后进入反应器中进行低温催化反应.燃油蒸气与氧气反应生成二氧化碳和水,反应后的混合高温气体在冷却其中被冷却并除去液态水后,得到混合惰气(Oxygen depleted air, ODA),最终被注回油箱上部气体空间进行冲洗惰化,来达到降低油箱氧浓度、惰化油箱的目的.
Fig. 1
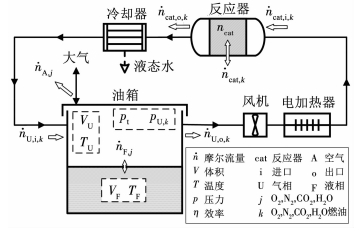
文中在建立数学模型时,基于以下假设:
1) 燃油虽然由复杂的碳氢化合物组成,本文认为其分子式可表示为CaHb,并且不考虑燃油分子中存在的微量元素,如氧、硫和氮等;
2) 忽略油箱与外界及气体与燃油的换热,认为油箱温度恒定为40 ℃; 催化反应器进出口的气体温度均为200 ℃,催化反应放出的热量被冷却介质冷却带走; 冷却器出口的混合惰气被冷却与油箱温度相同;
3) 利用冷却空气对反应器及冷却器进行冷却降温,并认为冷却空气冷却前后温差为100 ℃;
4) 忽略燃油箱内气体的混合及气体在燃油中逸出和溶解所需时间,并且气体的逸出和溶解满足平衡溶解关系;
5) 油箱内压力与外界环境压力一致,并且燃油蒸气分压与同温度下的饱和蒸气压相同,并按德里蒸气压计算,惰化过程在海平面进行;
6) 认为大气环境和燃油中不存在水蒸气.
2 数学模型在催化反应器中,反应过程满足式(1),对于RP-3燃油,分子式为CaHb,其中a=10.05,b=20.42.
${{\rm{C}}_a}{{\rm{H}}_b} + \left( {a + \frac{b}{4}} \right){{\rm{O}}_2} = a{\rm{C}}{{\rm{O}}_2} + \frac{b}{2}{{\rm{H}}_2}{\rm{O}}.$ (1)
由于催化反应中,即使氧气量足够,燃油蒸气也不能够完全反应,因此可定义催化反应器效率ηcat为
${\eta _{{\rm{cat}}}} = \frac{{{{\mathop n\limits^ \cdot }_{{\rm{cat}},{\rm{F}}}}}}{{{{\mathop n\limits^ \cdot }_{{\rm{cat}},{\rm{i}},F}}}}.$ (2)
若催化反应器进口气体中的氧气足量,而燃油蒸气不足,则参与反应的的燃油蒸气摩尔流量按下式确定:
${{\dot n}_{{\rm{cat}},{\rm{F}}}} = {{\dot n}_{{\rm{cat}},{\rm{i}},{\rm{F}}}}.$ (3)
如果进入催化反应器中的氧气不足,而燃油蒸气过量,则参与反应的氧气摩尔流量为
${{\dot n}_{{\rm{cat}},{\rm{O}}}} = {{\dot n}_{{\rm{cat}},{\rm{i}},{\rm{O}}}}. $ (4)
所以,催化反应器中参与反应的氧气以及反应所生成的二氧化碳和水蒸气的摩尔流量与参与反应的燃油蒸气量的关系满足:
${{\dot n}_{{\rm{cat}},{\rm{O}}}} = \left( {a + \frac{b}{4}} \right){{\dot n}_{{\rm{cat}},{{\rm{F}}^,}}}$ (5)
${{\dot n}_{{\rm{cat}},{\rm{C}}}} = {\rm{a}}{{\dot n}_{{\rm{cat}},{\rm{F}}}},$ (6)
${{\dot n}_{{\rm{cat}},{\rm{H}}}} = \frac{b}{2}{{\dot n}_{{\rm{ cat}},{\rm{F}}}}{\rm{.}}$ (7)
式中:
冷却介质为空气,因此由冷却器入口气体的温度及相对湿度,根据焓湿图,可确定其露点温度Td.并且已知冷却器出口气体温度为T(单位:K),此温度下对应的饱和蒸汽压为ps.由Td与T的大小关系,可以判断出冷却器出口气体是否为湿饱和状态.从而冷却器出口气体的含湿量、相对湿度、焓值、水蒸气分压力等参数均可被确定.
选取控制体为油箱气相空间,对O2、N2、CO2、H2O等组分建立状态方程,即
$({{\dot n}_{{\rm{U}},{\rm{i}},j}} - {{\dot n}_{{\rm{U}},o,j}}) + {{\dot n}_{{\rm{A}},j}} + {{\dot n}_{{\rm{F}},j}} = \frac{{{V_{\rm{U}}}}}{{R{T_{\rm{U}}}}}\frac{{{\rm{d}}{p_{{\rm{U}},j}}}}{{{\rm{d}}t}}. $ (8)
由亨利定律计算从燃油中向气相逸出或向液态燃油中溶解的各气体量,并按照平衡关系计算,当压力降低时,逸出值为正,可按下式确定:
${{\dot n}_{{\rm{F}},j}} = - \left( {\frac{{{\beta _j}{V_F}}}{{R{T_F}}}} \right)\frac{{{\rm{d}}{p_{{\rm{U}},j}}}}{{{\rm{d}}t}}. $ (9)
式中:
由于,在惰化时,流入与流出油箱的气体流量不同.因此,气体具体流动方向,需要从油箱内物质变化量来确定.
当流入油箱的气体量较多,油箱压力变大时,油箱中的气体按比例向外界环境排放,直至压力与外界相同,各组分排出量满足:
$ \frac{{{{\mathop n\limits^ \cdot }_{{\rm{A}}, {\rm{O}}}}}}{{{p_{{\rm{U}}, {\rm{O}}}}}} = \frac{{{{\mathop n\limits^ \cdot }_{{\rm{A}}, {\rm{N}}}}}}{{{p_{{\rm{U}}, {\rm{N}}}}}} = \frac{{{{\mathop n\limits^ \cdot }_{{\rm{A}}, {\rm{C}}}}}}{{{p_{{\rm{U}}, {\rm{C}}}}}} = \frac{{{{\mathop n\limits^ \cdot }_{{\rm{A}}, {\rm{H}}}}}}{{{p_{{\rm{U}}, {\rm{H}}}}^ \cdot }} $ (10)
当流回燃油箱的气体流量不足,油箱压力降低时,则外界气体流入油箱,且流量满足:
$ \frac{{{{\mathop n\limits^ \cdot }_{{\rm{A}}, {\rm{O}}}}}}{{{x_{{\rm{A}}, {\rm{O}}}}}} = \frac{{{{\mathop n\limits^ \cdot }_{{\rm{A}}, {\rm{N}}}}}}{{{x_{{\rm{A}}, {\rm{N}}}}}}, $ (11)
$ {\mathop n\limits^ \cdot _{{\rm{A}}, {\rm{C}}}} = {\mathop n\limits^ \cdot _{{\rm{A}}, {\rm{H}}}} = 0 $ (12)
补充计算条件为油箱气相各气体组分压力之和与外界环境总压力pt相同,即式中:
${p_{\rm{t}}} = {p_{{\rm{U}}, {\rm{O}}}} + {p_{{\rm{U}}, {\rm{N}}}} + {p_{{\rm{U}}, {\rm{C}}}} + {p_{{\rm{U}}, {{\rm{F}}^ \cdot }}} $ (13)
3 计算结果计算油箱尺寸为1 m×1 m×1 m,在考虑与不考虑燃油中气体溶解逸出两种状态下,计算了风机流量、载油率对3CIS生成水的性能影响,并比较了是否考虑有液态水析出对系统冷却性能的影响差异.
3.1 风机流量计算了在燃油中有、无气体溶解逸出两种情况下,RP-3燃油在风机流量20,30,40 L/min时,惰化系统的产水性能.首先得到油箱上部气相空间水蒸气体积分数及氧浓度随惰化时间的变化,如图 2所示.由于反应器内反应不断产生水,而冷却器不能将水完全除去,因此油箱上部水蒸气占比逐渐增大.与此同时,风机流量越大,反应强度越快,水蒸气体积分数上升越快,但最终浓度相差不大.另外,考虑燃油逸出时,水蒸气体积分数与不考虑燃油逸出时相比偏小,但总体相差不大.与水蒸气占比变化相反,油箱气相空间内氧气浓度逐渐降低,且风机流量越大,氧浓度下降越快; 特别地,燃油中气体溶解逸出对氧浓度影响明显,考虑逸出时,氧浓度更高,而由于不考虑燃油中溶解的水蒸气,因此溶解逸出主要通过氧氮的逸出间接影响水蒸气体积分数.
Fig. 2
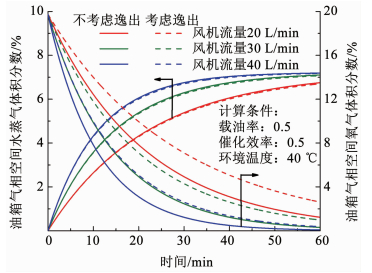
由于催化反应是放热额,所以需要提供冷却介质来对反应器进行冷却,将产生的热量带走.如图 3所示为反应器中所需冷却气体量随惰化时间的变化关系,可以看出,所需冷却气体流量逐渐变小.这是因为,随着惰化的不断进行,油箱气相空间氧浓度降低,导致反应强度也逐渐减弱.惰化刚开始时,风机流量较大,参与反应的燃油蒸气、氧气较多,放热量大,反应器所需冷却气体量多.同时,油箱气相氧浓度下降的也更快,因此惰化一段时间后,油箱抽吸的流量更大时,参与反应的氧气量反而更少,因此所需冷却气体量更低.值得注意的是,考虑燃油中气体溶解逸出时,随着油箱上部氧浓度的减少,燃油中的氧气逸出,因此参与反应的氧气量更多,反应放热量也更大,反应器中所需冷却空气量较不考虑溶解逸出时平均高9.1%.
Fig. 3
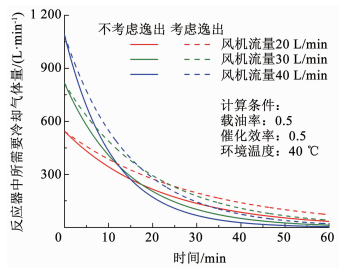
除了反应器外,冷却器中也需要提供冷却介质,计算得到考虑及不考虑水析出时冷却器中所需的冷却气体流量,由图 4可见,风机流量越大,冷却器中所需冷却气体量更多.考虑有水析出时,冷却器中所需冷却气体量较不考虑水析出时平均高33%,这是由于水蒸气冷凝为液态水时放出大量潜热.另外,燃油中逸出的氧气为反应提供原料会产生更多的水蒸气,需被冷凝成液态水,故考虑水析出较不考虑水析出相比,燃油中气体逸出对冷却器中冷却气量计算结果影响更大; 由于燃油中气体溶解逸出问题在整个航程中一直存在,在高空尤甚,因此,液态水析出对催化惰化系统性能影响巨大.
Fig. 4
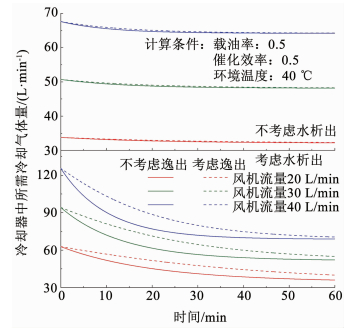
图 5为基于是否考虑溶解氧逸出情况,在不同风机流量下,反应器出口相对湿度随时间的变化.随着惰化的进行,反应强度逐渐减弱,反应器中产生的水蒸气也慢慢减少.初始时,氧气充足,无论抽吸流量大小,生成的水都是成比例的,反应器出口相对湿度相同,但风机抽吸流量大,油箱内氧浓度下降的更快,再次进入反应器的单位流量的氧含量就少,因此单位流量反应产生的水也变小,故此相对湿度更低; 另外,风机流量越小,燃油中逸出的氧气占抽吸气的比例越大,进入反应器中参与反应,因此考虑溶解逸出对结果影响越大,如风机流量为20,40 L/min时,考虑逸出的计算结果分别约是不考虑逸出时的2.08、4.15倍.
Fig. 5
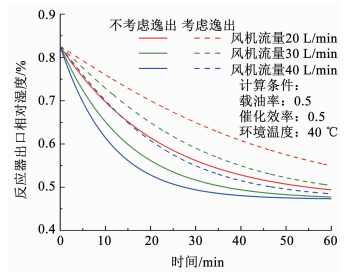
冷却器中析出的水量随时间的变化如图 6所示,其变化趋势及原因与反应器中需冷却气量相同,在该条件下时,冷却器出口返回油箱的混合惰气始终为湿饱和状态,即相对湿度为100%,含湿量恒定为48.4 g/kg干空气.
Fig. 6
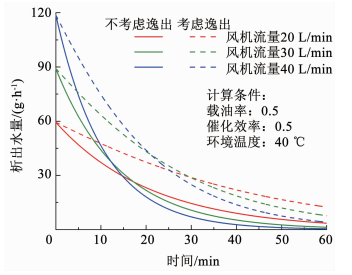
3.2 载油率载油率表示油箱内燃油量的相对大小,随时间递减.载油率越小,燃油越少,燃油箱气相空间体积越大.本文计算了RP-3燃油在载油率0.2、0.5、0.8的情况下,系统各性能参数随惰化时间的变化情况.
图 7为不同载油率下,油箱气相空间水蒸气体积分数的变化曲线,不难发现,载油率越高,油箱上部气相空间水蒸气体积分数上升越快.另外,考虑燃油逸出时,水蒸气体积分数偏小,且载油率越大,燃油中氧氮的溶解逸出对结果影响越大.
Fig. 7
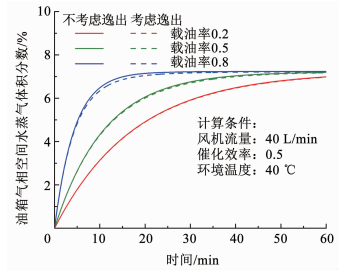
反应器需冷却气量随时间的变化关系如图 8所示,载油率越大,反应器需冷却气量越少,这是因为气相空间体积越小,需要被置换出的氧气量也越少,氧浓度下降越快,反应放热越少; 载油率越大,考虑逸出对结果影响更大,如载油率分别为0.2、0.5、0.8时,考虑逸出的结果分别是无逸出时的1.19、2.39、8.35倍.
Fig. 8
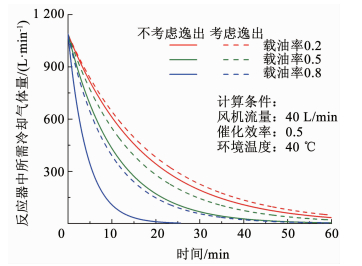
冷却器中有水析出,会释放出大量潜热,因此是否考虑水析出,冷却器中所需冷却气体量结果相差较大,如图 9所示,特别地,考虑水析出时,载油率为0.8,考虑溶解逸出,由于露点温度发生变化,曲线刚开始有一段上升.
Fig. 9
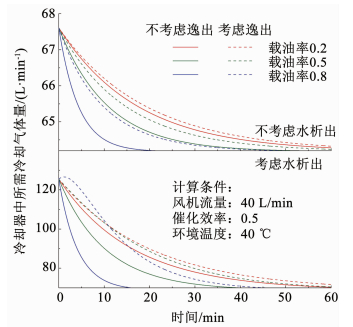
随着惰化的进行,油箱气相上部氧浓度逐渐降低,反应器中产生的水也越来越少,冷却器在不同载油率下的除水量分别如图 10所示,可以看出载油率越大,则燃油中气体的溶解逸出影响越大.
Fig. 10

4 结论1) 油箱气相空间的氧浓度随惰化的进行逐渐降低,水蒸气体积分数逐渐增加,但增加速率逐渐减缓; 而催化反应器所需冷却气量、冷却器所需冷却气量、反应器出口相对湿度、冷却器中除水量等均随时间逐渐降低.
2) 风机流量、载油率等关键参数对系统水性能影响较大,风机流量越大,油箱水蒸气体积分数上升越快、需要的冷却空气量更大; 载油率越高,气相体积越小,水蒸气体积分数上升越快,但需要的冷却空气量更少.
3) 燃油中气体的溶解逸出对系统性能有较大影响,考虑溶解逸出时,油箱氧浓度更高而水蒸气体积分数偏小、系统需更多的冷却介质,析出的水量也更高.
4) 是否考虑冷却器有液态水析出,计算得到的冷却器中所需的冷却气体量结果差别较大,故未来在设计惰化系统时,应充分考虑系统的产水及水析出对系统性能的影响.
参考文献
[1] DESMARAIS L, TOLLE F, ALLEN T. Evaluation of advanced airplane fire extinguishants[C]//Proceedings of the 19th Joint Propulsion Conference. Seattle, WA: AIAA, 2013: 1. DOI: 10.2514/6.1983-1141
[2] GRENICH A F, JOHNSON A M, DESMARAIS L A, et al. Vulnerability methodology and protective measures for aircraft fire and explosion hazards: AFWAL-TR-85-2060[R]. Seattle: Boeing Military Airplane Company, 1986
[3] LANGTON R, CLARK C, HEWITT M, et al. Aircraft fuel systems[M]. [S.l.]: John Wiley and Sons, 2009: 1. DOI: 10.1002/9780470059470
[4] GILLERMAN J B, JOHNSON R L. Aircraft fuel tank inerting system: AFWALTR-82-2115[R]. [S.l.]: Final Report, 1983
[5] CAVAGE W M. The cost of implementing ground-based fuel tank inerting in the commercial fleet: DOT/FAA/AR-00/19 [R]. [S.l.]: FAA Report, 2000
[6] REYNOLDS T L, BAILEY D, LEWINSKI D, et al. Onboard inert gas generation system onboard oxygen gas generation system (OBIGGS OBOGS) study: NASA/CR-2001-210903/PT1[R]. Washington DC: NASA, 2001
[7] SMITH D E. Fuel tank inerting systems for civil aircraft[D]. Colorado: Colorado State University, 2014: 32
[8] GUPTA A. System and method to make a fuel tank inert: US 7918358 B2 [P]. 2011
[9] GUPTA A. Fuel vapor removal methods and systems for flammability reduction: US 20130255493 A1 [P]. 2013
[10] JOHNSON R W, ZAKI R, YATES S F. Advanced carbon dioxide fuel tank inerting system: 7905259 B2[P]. 2011
[11] MORRIS R W, MILLER J, LIMAYE S Y. Fuel deoxygenation and aircraft thermal management[C]//Proceedings of the 4th International Energy Conversion Engineering Conference and Exhibit. San Diego: AIAA, 2013: 1. DOI: 10.2514/6.2006-4027
[12] WALKER S, JUNG W, ROBERTSON S. Demonstration of a novel catalyst based green on board inert gas generation system (GOBIGGS)for fuel tank inerting[C]//Proceedings of the AHS 69th Annual Forum. Phoenix: American Helicopter Society International, Inc., 2013
[13] STUART R, WESLEY J, DONALDK, et al. Development of green on-board inert gas generation system (GOBIGGSTM) [R]. 2007
[14] LI Chaoyue, FENG Shiyu, SHAO Lei, et al. Measurement of the Diffusion coefficient of water in RP-3 and RP-5 jet fuels using digital holography interferometry[J]. International Journal of Thermophysics, 2018, 39(4): 53. DOI:10.1007/s10765-018-2373-4
[15] 冯诗愚, 邵垒, 李超越, 等. 航空燃油类型对催化惰化系统性能的影响[J]. 航空学报, 2016, 37(6): 1819.
FENG Shiyu, SHAO Lei, LI Chaoyue, et al. Performance of catalytic inerting system affected by various aviation jet fuels[J]. Acta Aeronautica et Astronautica Sinica, 2016, 37(6): 1819. DOI:10.7527/S1000-6893.2015.0344
[16] 刘永峰, 裴普成, 田洪森. 缸内燃油喷射发动机碳烟生成机理特征分析[J]. 哈尔滨工业大学学报, 2012, 44(7): 102.
LIU Yongfeng, PEI Pucheng, TIAN Hongsen. Analysis on soot formation mechanism in direct-injection fuel engine[J]. Journal of Harbin Institute of Technology, 2012, 44(7): 102. DOI:10.11918/j.issn.0367-6234.2012.07.020
[17] 童升华.国产燃油理化性能与易燃性研究[D].南京: 南京航空航天大学, 2013: 19
TONG Shenghua. Research on physicochemical characteristics &. flammability of domestic fuels[D]. Nanjing: Nanjing University of Aeronautics and Astronautics, 2013: 19
[18] 高秀峰, 刘卫华, 熊斌, 等. 飞机燃油箱冲洗惰化过程的理论研究[J]. 西安交通大学学报, 2010, 44(9): 16.
GAO Xiufeng, LIU Weihua, XIONG Bin, et al. Theoretical study of washing inerting process in aircraft fuel tank[J]. Journal of Xi'an Jiaotong University, 2010, 44(9): 16.
[19] 李超越, 冯诗愚, 邵垒, 等. RP-3航空燃油中CO2扩散系数实验分析[J]. 北京航空航天大学学报, 2018, 44(4): 765.
LI Chaoyue, FENG Shiyu, SHAO Lei, et al. Experimental analysis of carbon dioxide diffusion coefficient in RP-3 jet fuel[J]. Journal of Beijing University of Aeronautics & Astronautics, 2018, 44(4): 765. DOI:10.13700/j.bh.1001-5965.2017.0265
[20] 高阳, 吴文海, 周思羽. 垂直/短距起降飞机非线性动力学建模与仿真[J]. 哈尔滨工业大学学报, 2019, 51(4): 121.
GAO Yang, WU Wenhai, ZHOU Siyu, et al. Nonlinear aerodynamic modeling and simulation of vertical and/or short take-off and landing aircraft[J]. Journal of Harbin Institute of Technology, 2019, 51(4): 121. DOI:10.11918/j.issn.0367-6234.201712110