Fang Fang1,Gang Wu2,Hongjun Han1,Mingnin Ma1
(1.School of Municipal and Environmental Engineering,Harbin Institute of Technology,Harbin 150090,China;2.Shuangliang Group Co.Ltd.,Jiangyin 214400,Jiangsu,China)
Abstract:
The improvement effect of bioaugmentation with phenol degrading bacteria (PDB) on pollutants removal and chemicals consumption was investigated in a full-scale Lurgi coal gasification wastewater (LCGW) treatment plant. Bioaugmentation with PDB applied in biological contact oxidation tank(BCOT) was carried out in summer to prevent the limitation of low temperature on the bacteria activity. After augmentation, the removal of COD and total phenol (TPh) was significantly enhanced, with efficiencies from 78.5% and 80% to 82.3% and 86.6% in BCOT, respectively. The improvement could also be detected in further processes, including anoxic-oxic, coagulation sedimentation and biological aerated filter, with COD and TPh removal efficiencies increment from 70.1%, 24.7% and 53.4% to 73.9%, 29.1% and 55.9%, from 67.1%, 20% and 25% to 72.5%, 25% and 32%, respectively. In addition, chemicals used for denitrification and coagulation sedimentation showed considerable reduction after bioaugmentation, with methanol, coagulant, coagulant aid and bleaching dosage from 100.0,0.0,0.0 and 60.0 mg/L to 85.0,0.6,7.8 and 45.7 mg/L, respectively. Therefore, bioaugmentation with PDB can be a viable alternative for LCGW treatment plant in pollutants removal and chemicals saving.
Key words: bioaugmentation phenol degrading bacteria Lurgi coal gasification wastewater treatment
DOI:10.11916/j.issn.1005-9113.16153
Clc Number:X703
Fund:
Fang Fang, Gang Wu, Hongjun Han, Mingnin Ma. Improvement Effect of Bioaugmentation with Phenol Degrading Bacteria on Lurgi Coal Gasification Wastewater Treatment[J]. Journal of Harbin Institute of Technology, 2017, 24(6): 52-59. DOI: 10.11916/j.issn.1005-9113.16153.

Fund Sponsored by China Postdoctoral Science Foundation (Grant No.2016M600254) Corresponding author Hongjun Han, E-mail:han13946003379@163.com Article history Received: 2016-08-01
Contents Abstract Full text Figures/Tables PDF
Improvement Effect of Bioaugmentation with Phenol Degrading Bacteria on Lurgi Coal Gasification Wastewater Treatment
Fang Fang1, Gang Wu2, Hongjun Han1


1. School of Municipal and Environmental Engineering, Harbin Institute of Technology, Harbin 150090, China;
2. Shuangliang Group Co.Ltd., Jiangyin 214400, Jiangsu, China
Received: 2016-08-01
Fund: Sponsored by China Postdoctoral Science Foundation (Grant No.2016M600254)
Corresponding author: Hongjun Han, E-mail:han13946003379@163.com
Abstract: The improvement effect of bioaugmentation with phenol degrading bacteria (PDB) on pollutants removal and chemicals consumption was investigated in a full-scale Lurgi coal gasification wastewater (LCGW) treatment plant. Bioaugmentation with PDB applied in biological contact oxidation tank(BCOT) was carried out in summer to prevent the limitation of low temperature on the bacteria activity. After augmentation, the removal of COD and total phenol (TPh) was significantly enhanced, with efficiencies from 78.5% and 80% to 82.3% and 86.6% in BCOT, respectively. The improvement could also be detected in further processes, including anoxic-oxic, coagulation sedimentation and biological aerated filter, with COD and TPh removal efficiencies increment from 70.1%, 24.7% and 53.4% to 73.9%, 29.1% and 55.9%, from 67.1%, 20% and 25% to 72.5%, 25% and 32%, respectively. In addition, chemicals used for denitrification and coagulation sedimentation showed considerable reduction after bioaugmentation, with methanol, coagulant, coagulant aid and bleaching dosage from 100.0, 100.0, 80.0 and 60.0 mg/L to 85.0, 70.6, 57.8 and 45.7 mg/L, respectively. Therefore, bioaugmentation with PDB can be a viable alternative for LCGW treatment plant in pollutants removal and chemicals saving.
Key words: bioaugmentation phenol degrading bacteria Lurgi coal gasification wastewater treatment
1 Introduction Lurgi coal gasification wastewater (LCGW) has become the critical factor which is seriously hindering the development of coal chemical industry[1]. Due to relatively low carbonization temperature, organic carbon of LCGW was high to 10 000-20 000 mg/L, which contains large amount of refractory and toxic components, such as phenols, alkanes, aromatic hydrocarbons, heterocyclic compounds and ammonia nitrogen[2]. Thus, a series of physiochemical and biological processes were usually adopted together for pollutants removal, including phenol and ammonia recovery and followed-up anaerobic-anoxic-aerobic treatment. However, the effluent COD was still high to 130-180 mg/L, with BOD5/COD value of 0.05-0.07[3]. In order to meet standards of industrial circulating cooling water, advanced treatment process (e.g. coagulation sedimentation, activated carbon adsorption, advanced oxidation) was adopted for further treatment[4].
However, these further processes have cost challenges in terms of chemicals and energy consumption. Therefore, improvement of biological technology was still topic issue for LCGW treatment. Previous researches focused on improving efficiency by technological optimization, such as altering influent split ratio, adding filler, adding co-metabolic substrate[5-7]. Nevertheless, the final effluent quality of bio-process was not improved satisfactorily. Due to the harsh influent (with total phenols (TPh) 300-700 mg/L), the effluent TPh was still high to 15-25 mg/L[8-9].
Bioaugmentation is one of the most straightforward strategies for accelerating the removal and biodegradation of undesired pollutants[10-11]. Specialized bacteria inoculation was one of the most common methods for bioaugmentation. Phenol degrading bacteria (PDB) have been studied for decades, which showed prospective impact on enhancing the efficiency of various reactors[12-14]. However, bioaugmentation with special bacteria in a full-scale biotreatment system does not always work due to various reasons, such as complex operation conditions, different packing materials, sludge discharge and so on.
In this paper, bioaugmentation with PDB (isolated from our laboratory)[15]was employed in biological contact oxidation tank (BCOT) of China Coal Longhua Harbin Coal Chemical Industry Co. Ltd. Effect of operation conditions on phenol degradation ability of PDB was investigated. Meanwhile, the performance of BCOT, anoxic-oxic (A/O), coagulation sedimentation (CS) and biological aerated filter (BAF) before and after augmentation was evaluated. Furthermore, effect of bioaugmentation on chemicals consumption and cost was also assessed.
2 Materials and Methods 2.1 PDB for Bioaugmentation The PDB (FF1, FF2, FF3) which is capable of converting refractory phenolic compounds into easily-biodegradable compounds was previously isolated[15]. The fermentation and enrichment of PDB was on the behalf of YanTai North Chemical Technology Research Institute. The inoculation dosage of PDB was 0.55‰ mL per 1 L wastewater. The fermented agent of PDB was mixed (according to volume ratio, FF1: FF2:FF3=1:1:1) and activated with diluted LCGW for 24 h before augmentation.
In order to investigate effect of operation conditions on phenol degradation ability of PDB, series batch tests were carried out with phenol as sole carbon source. Due to volatilization of phenol, sealing bottles with inlet and outlet air pipe were required. Narrowed end of the outlet pipe which could realize physical cooling was the special place. Condensate recovery was the key for reducing volatilization of phenol. Moreover, high concentration of phenol (300 mg/L) was chosen to exam the effect on phenol degradation ability of PDB. Thus, the loss of phenol due to volatilization could be ignored. The cultures were incubated at the conditions of macro-nutrients, with temperature, DO and pH in the range of 20-40 ℃, 0.5-5.5 mg/L and 6-8, respectively. The phenol removal was determined to exam the effect on phenol degradation ability of PDB.
2.2 Operation Characteristics of BCOT China Coal Longhua Harbin Coal Chemical Industry Co. Ltd, located between Longitude 129°11′50″-130°11′40″ and latitude 45°51′40″-46°39′20″, belongs to the frigid zone of continental monsoon climate. The BCOT was operated under a continuous flow rate of 360 m3/h and a hydraulic retention time (HRT) of 36 h. The environmental temperature from summer to winter was significantly different. The treatment processes investigated were shown in Fig. 1(a). Seven monitoring points were sited in the inlet and outlet of each compartment (Fig. 1(b)). Table 1 summarizes temperature, DO and pH value of BCOT in summer and winter, respectively.
Figure 1
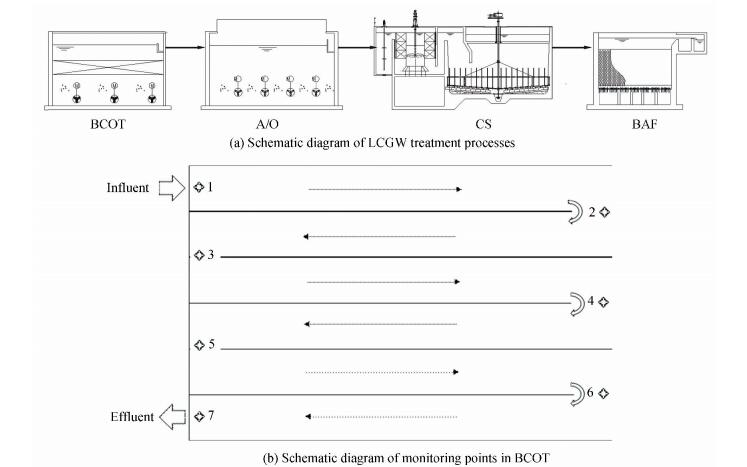
表 1
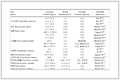
Summer Winter Summer Winter Summer Winter
Point 1 38.90±1.120 38.80±1.200 0.42±0.173 0 0.50±0.120 7.48±0.020 0 7.42±0.243 0
Point 2 36.20±0.960 34.20±1.080 0.54±0.080 0 0.63±0.152 6.30±0.120 0 6.60±0.080 0
Point 3 33.00±0.800 30.90±0.654 0.92±0.054 0 1.32±0.180 6.70±0.098 0 6.90±0.158 0
Point 4 30.00±0.800 27.60±0.837 1.50±0.137 0 2.20±0.100 7.02±0.100 0 7.10±0.137 0
Point 5 29.80±1.230 24.10±1.253 2.30±0.253 0 3.23±0.230 7.03±0.050 0 7.12±0.173 0
Point 6 27.80±0.686 22.30±0.784 3.06±0.184 0 4.10±0.186 7.10±0.028 3 7.26±0.074 8
Point 7 25.90±1.213 20.40±0.557 4.63±0.157 4 5.22±0.213 7.12±0.153 0 7.23±0.107 4
Table 1 Operation characteristics of BCOT in different seasons
2.3 Start up and Operation of BCOT Augmented with PDB As shown in Table 2, from day 1 to day 6, the influent of BCOT was controlled at low organic loading (COD 0.12 kg/(m3·d), TPh 0.12 kg/(m3·d) and NH3-N 0.02 kg/(m3·d)). During this period, the activated PDB was inoculated to BCOT in five times within the first five days. Afterwords, the organic loading of influent was increased stepwise every 7 days. At the end of this phase, the organic loading of COD, TPh and NH3-N were increased to 1.22 kg/(m3·d), TPh 0.17 kg/(m3·d) and NH3-N 0.1 kg/(m3·d), with the corresponding concentration on average of 2 240, 264.7 and 220.5, respectively.
表 2
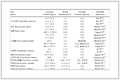
Phase COD TPh NH3-N
Day 1-6 0.12 0.10 0.020
Day 7-12 0.46 0.12 0.035
Day 13-18 0.93 0.17 0.060
Day 19-30 1.22 0.21 0.100
Table 2 COD, TP and NH3-N load of the influent in different phases
2.4 Analysis Methods DO and pH values were determined with a hybrid meter (HACH, USA). For organic analysis, the pretreated sample was detected by GC-MS according to the previous method[16]. COD, TPh, NH3-N and SS were measured according to Standard Methods[17]. Bacterial community structure were conducted according to PCR-DGGE analysis in our previous study[15].
3 Results and Discussion 3.1 Effect of BCOT Operation Conditions on Phenol Degradation Ability of PDB Based on the data in Table 1, temperature, DO and pH in the range of 20-40 ℃, 0.5-5.5 mg/L and 6-8 were chosen to exam the effect on phenol degradation ability of PDB.As shown in Fig. 2(a), phenol removal was enhanced with temperature increased from 20 ℃ to 30 ℃. After that, no phenol could be detected with temperature increasing to 40 ℃. This suggests that satisfactory performance of bioaugmentation with PDB would be achieved more possibly with BCOT operated in summer. Moreover, phenol degradation ability of PDB could be improved in compartment 1 to 3 in summer and compartment 1 to 2 in winter with temperature in the range of 30-40 ℃. Fig. 2(b) and (c) demonstrated that DO and pH alternation did not influence phenol degradation ability of PDB. Phenol was under detectable level with DO and pH in the range of 0.5-5.5 mg/L and 6-8 respectively. Therefore, it could be concluded that DO and pH in the range of BCOT operation conditions were not limit factors for bioaugmentation.
Figure 2
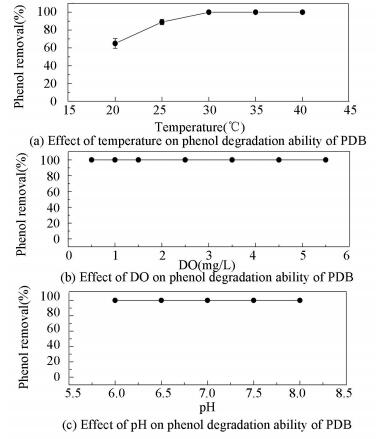
3.2 Effect of Bioaugmentation on BCOT, A/O, CS and BAF 3.2.1 Effect of bioaugmentation on BCOT As shown in Fig. 3, the performance of BCOT was significantly enhanced after the augmentation, with the removal efficiencies of COD, TPh and NH3-N reached 82.3%, 86.6% and 27.3%, respectively, compared to 78.5%, 80% and 25.1% before augmentation. The pollutants removal efficiencies in full-scale were noticeably higher than those in laboratory. As shown in the previous results in laboratory, the bioaugmentation of PDB improved the removal of COD, TPh and NH3-N, with efficiencies from 58%, 66% and 5% to 78%, 80% and 25%, respectively. Compared to four compartments in laboratory, six compartments in full-scale plant which supplied more bio-contact oxidation might be the critical reason. Due to more TPh removed ahead, the followed-up compartments with less toxic compounds was favorable for accumulation of ammonia oxidation bacteria. The results also indicated that the enhancement of bioaugmentation in full-scale was not as good as in laboratory. The reason was complicated and intertwined, such as relatively complex operation conditions, different packing materials, sludge discharge and so on, which would be investigated in further experiments.
Figure 3
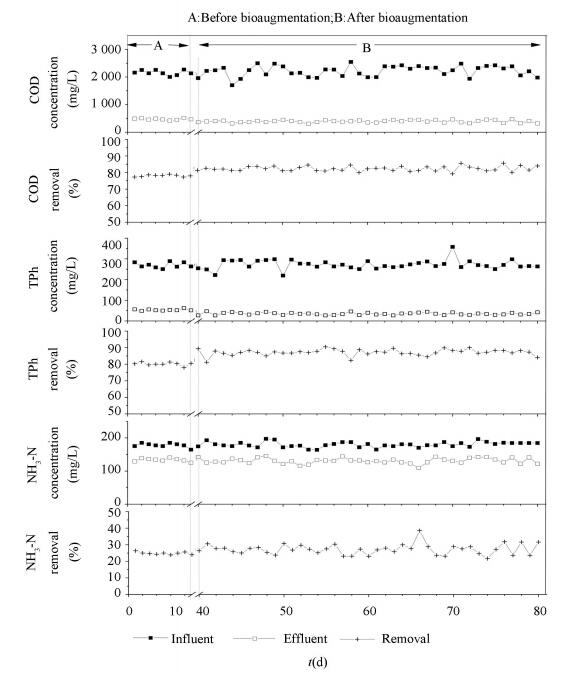
Fig. 4(a) and (b) showed a DGGE profile of bacterial community from BCOT before and after augmentation. The results were analysis with operational taxonomic units (OTUs), relative distributions (evenness) and Shannon diversity index (as shown in Table 3). The difference of OTUs indicated that the samples after augmentation showed more rich microbial community. Although the values of Shannon diversity index showed little difference, several intensive bands arising in the samples after augmentation could be observed. It was demonstrated that ecological stability and the ability of toxic resistance attributed to the structure improvement of bacterial community[18].
Figure 4
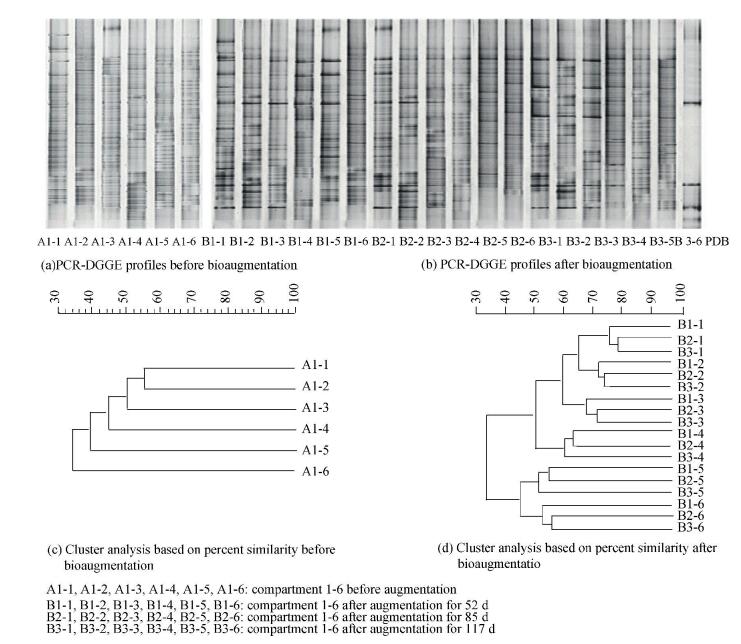
表 3
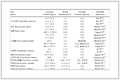
A1-1 26 4.700 0.962 B2-1 30 4.907 0.967
A1-2 27 4.755 0.963 B2-2 31 4.954 0.968
A1-3 28 4.807 0.964 B2-3 35 5.129 0.971
A1-4 30 4.907 0.967 B2-4 36 5.170 0.972
A1-5 31 4.954 0.968 B2-5 36 5.170 0.972
A1-6 32 5.000 0.969 B2-6 37 5.209 0.973
B1-1 28 4.807 0.964 B3-1 28 4.807 0.964
B1-2 30 4.907 0.967 B3-2 30 4.907 0.967
B1-3 29 4.858 0.966 B3-3 31 4.954 0.968
B1-4 32 5.000 0.969 B3-4 32 5.000 0.969
B1-5 36 5.170 0.972 B3-5 35 5.129 0.971
B1-6 38 5.248 0.974 B3-6 36 5.170 0.972
Table 3 Biodiversity estimation based on 16S rRNA clone libraries
In order to investigate long-term performance of bioaugmentation with PDB, the samples collected on day 52, 85 and 117 were compared with percent similarity (Fig. 4(c) and (d)). The results showed that the bacterial community in each compartment of BCOT was in a highly similar percent. This indicated that augmentation with PDB has had a long-lasting effect on diversity improvement and pollutants removal[19].
3.2.2 Effect of bioaugmentation on further treatment process The average removal efficiencies of COD, TPh and NH3-N in each unit were shown in Fig. 5. After augmentation with PDB, the A/O, CS and BAF showed considerable improvement in TPh removal, with average efficiency from 67.1%, 20% and 25% to 72.5%, 25% and 32%, respectively. Meanwhile, the COD removal efficiency in A/O, CS and BAF increased from 70.1%, 24.7% and 53.4% to 73.9%, 29.1% and 55.9%, respectively. However, for NH3-N, the removal efficiency was improved slightly. This was possibly attributed to reduction of methanol dosage in A/O after augmentation[20].
Figure 5
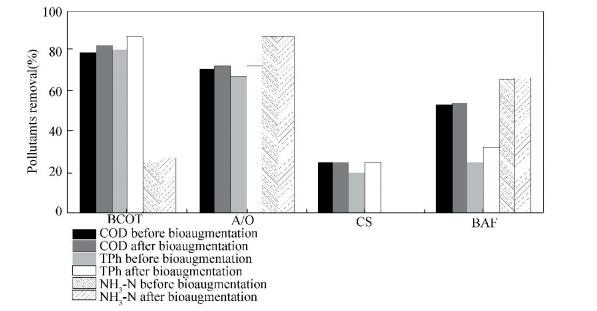
3.2.3 Effect of bioaugmentation on organic composition of effluent Effect of bioaugmentation on organic pollutants removal was analyzed by comparison on organic composition of influent and effluent of BCOT, A/O, CS and BAF before and after augmentation. As Fig. 6 shown, the relative amounts of phenols, benzenes, naphthalene and furan was significantly decreased, which were refractory to aerobic microorganism. Moreover, the relative amounts of alkanes which could not be removed sufficiently were also decreased after augmentation. The inefficient degradation of organics above was the main reason for excessive chemicals consumption in A/O and CS. And the relative amount of ethers, alkene and amide increased in CS and BAF, which could be attributed to more refractory compounds and alkanes removal in the pretreated processes of BCOT and A/O.Thus, it can be illustrated that bioaugmentation with PDB was helpful in enhancing pollutants removal for the whole processes of BCOT, A/O, CS and BAF.
Figure 6
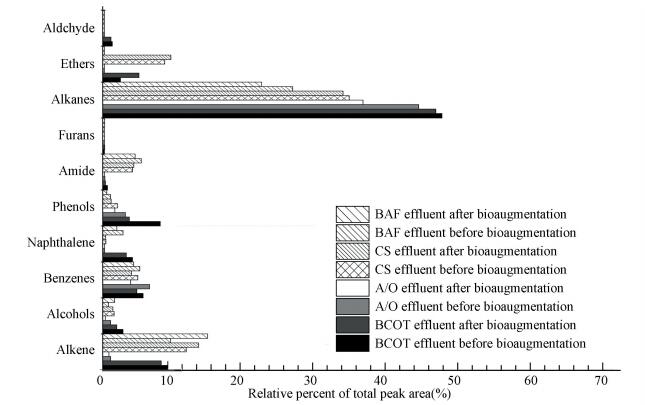
3.3 Effect of bioaugmentation on Chemicals Consumption 3.3.1 Effect of bioaugmentation on methanol consumption As shown in Fig. 3, the average removal efficiencies of TPh and COD in BCOT reached 72.5% and 73.9%, respectively. Most of toxic and refractory organic compounds were decomposed in BCOT, which were benefit for ammonia nitrogen process in A/O[21]. Moreover, the effluent biodegradability of BCOT was improved, with BOD5/COD value increasing from 0.35 to 0.39 (Fig. 7). This also provided a relative favorable environment for ammonia nitrogen process[22]. Thus, similar removal efficiency of ammonia nitrogen was achieved in A/O, with methanol dosage decreased from 100 mg/L to 85 mg/L after augmentation.
Figure 7
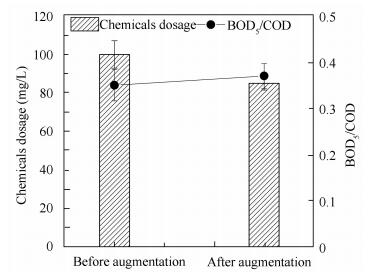
3.3.2 Effect of bioaugmentation on coagulant, coagulant aid and bleaching agent consumption As shown in Fig. 8, the dosage of chemicals was significantly reduced after augmentation. The dosage of coagulant, coagulant aid and bleaching agent after augmentation were 70.6, 57.8 and 45.7 mg/L respectively, compared to 100.0, 80.0 and 60.0 mg/L before augmentation. Although chemicals consumption was reduced after augmentation, a significant increasein the organic removal was also achieved. This could be caused by effective removal of organic compounds in BCOT and A/O after augmentation.
Figure 8
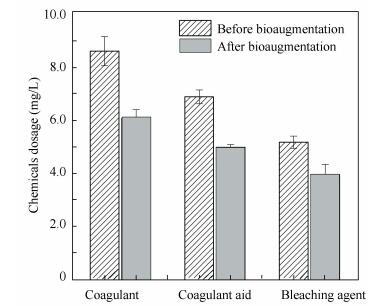
3.3.3 Economic analysis of bioaugmentation with PDB Chemicals consumption was evaluated with the system running more than 80 days. As shown in Table 4, the consumption of methanol, coagulant, coagulant aid and bleaching agent after augmentation was significantly reduced, with dosage from 864.0, 864.0, 691.0 and 518.8 kg/d to 734.4, 610.0, 500.0 and 395.0 kg/d, respectively. The corresponding average cost reduced from 1 728.0, 1 728.0, 6 910.0 and 3 112.0 yuan/d to 1 468.8, 1 360.0, 5 000.0 and 2 460.0 yuan/d, respectively. Thus, the cost reduced by less chemical consumption was 3 189.2 yuan/d and 114.8×104 yuan/a. The cost increased by bioaugmentation was 6.2×104yuan/a, which was calculated in accordance with PDB agent of 478.9 yuan/m3, dosage of volume ratio 0.55‰ and adding frequency of half a year. Therefore, it can be calculated that bioaugmentation with PDB for LCGW treatment could achieve cost saving of 108.6×104 yuan/a.
表 4
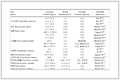
(yuan/t) Before augmentation After augmentation
Consumption (kg/d) Cost (yuan/d) Consumption (kg/d) Cost (yuan/d)
Methanol 2 000.0 864.0 1 728.0 734.4 1 468.8
Coagulant 2 000.0 864.0 1 728.0 610.0 1 360.0
Coagulant aid 10 000.0 691.0 6 910.0 500.0 5 000.0
Bleaching Agent 6 000.0 518.8 3 112.0 395.0 2 460.0
Total - 2 937.8 13 478.0 2 239.4 10 288.8
Table 4 Chemicals consumption and cost in LCGW treatment
4 Conclusions 1) DO (0.5-5.5 mg/L) and pH (6-8) in the range of BCOT operation conditions were not limit factors for bioaugmentation. Due to the limitation of low temperature ( < 30 ℃), bioaugmentation with PDB applied in BCOT should be carried out in summer.
2) Bioaugmentation with PDB was helpful in enhancing pollutants removal for the whole processes of BCOT, A/O, CS and BAF, with COD and TPh removal efficiencies increment from 82.3%, 70.1%, 24.7% and 53.4% to 86.6%, 73.9%, 29.1% and 55.9%, from 78.5%, 67.1%, 20% and 25% to 80%, 72.5%, 25% and 32%, respectively.
3) Microbial community analysis demonstrated that augmentation with PDB has had a long-lasting effect on diversity improvement and the PDB embodied as dominant populations in the bacteria community, which conversely enhanced the performance of the whole process.
4) Chemicals used for denitrification and coagulation sedimentation showed a considerable reduction after bioaugmentation with PDB, which could achieve cost saving of 108.6×104yuan/a.
5) Therefore, bioaugmentation via the enhancement of PDB will be expected to be a promising strategy for improving pollutants removal and chemicals saving.
References
[1] Zhuang H F, Han H J, Ma W C, et al. Advanced treatment of biologically pretreated coal gasification wastewater by a novel heterogeneous Fenton oxidation process. Journal of Environmental Science, 2015, 33: 12-20. DOI:10.1016/j.jes.2014.12.015 (

[2] Jia S Y, Han H J, Hou B L, et al. Advanced treatment of biologically pretreated coal gasification wastewater by a novel integration of three-dimensional catalytic electro-Fenton and membrane bioreactor. Bioresource Technology, 2015, 198: 918-921. DOI:10.1016/j.biortech.2015.09.080 (

[3] Zhuang H F, Han H J, Zhuang H F, et al. Advanced treatment of biologically pretreated coal gasification wastewater by a novel integration of catalytic ultrasound oxidation and membrane bioreactor. Bioresource Technology, 2015, 189: 426-429. DOI:10.1016/j.biortech.2015.04.062 (

[4] Zhuang H F, Han H J, Hou B L, et al. Heterogeneous catalytic ozonation of biologically pretreated Lurgi coal gasification wastewater using sewage sludge based activated carbon supported manganese and ferric oxides as catalysts. Bioresource Technology, 2014, 166: 178-186. DOI:10.1016/j.biortech.2014.05.056 (

[5] Wang W, Han H J, Yuan M, et al. Treatment of coal gasification wastewater by a two-continuous UASB system with step-feed for COD and phenols removal. Bioresource Technology, 2011, 102: 5454-5460. DOI:10.1016/j.biortech.2010.10.019 (

[6] Zhao Q, Han H J, Xu C Y, et al. Powdered activated carbon technology on short-cut nitrogen removal for coal gasification wastewater. Bioresource Technology, 2013, 142: 179-185. DOI:10.1016/j.biortech.2013.04.051 (

[7] Wang W, Ma W C, Han H J, et al. Thermophilic anaerobic digestion of Lurgi coal gasification wastewater in a UASB reactor. Bioresource Technology, 2011, 102: 2441-2447. DOI:10.1016/j.biortech.2010.10.140 (

[8] Xu P, Han H J, Zhuang H F, et al. Advanced treatment of biologically pretreated coal gasification wastewater by a novel integration of heterogeneous Fenton oxidation and biological process. Bioresource Technology, 2015, 182: 389-392. DOI:10.1016/j.biortech.2015.02.019 (

[9] Zhao Q, Han H J, Fang F, et al. Performance of the full-scale loop hybrid reactor treating coal gasification wastewater under different recirculation modes. Journal of Harbin Institute of Technology, 2015, 22(5): 31-37. (

[10] Tale V P, Maki J S, Zitomer D H. Bioaugmentation of overloaded anaerobic digesters restores function and archaeal community. Water Research, 2015, 70: 138-147. DOI:10.1016/j.watres.2014.11.037 (

[11] Chen M X, Fan R, Zou W H, et al. Bioaugmentation for treatment of full-scale diethylene glycol monobutyl ether (DGBE) wastewater by Serratia sp. BDG-2. Journal of Hazardous Materials, 2016, 309: 20-26. DOI:10.1016/j.jhazmat.2016.01.076 (

[12] Herrero M, Stuckey D C. Bioaugmentation and its application in wastewater treatment: A review. Chemosphere, 2015, 140: 119-128. DOI:10.1016/j.chemosphere.2014.10.033 (

[13] Jemaa Z, Suárez-Ojeda M E, Pérez J, et al. Partial nitritation and o-cresol removal with aerobic granular biomass in a continuous airlift reactor. Water Research, 2014, 48: 354-362. DOI:10.1016/j.watres.2013.09.048 (

[14] Monsalvo V M, Tobajas M, Mohedano A F, et al. Intensification of sequencing batch reactors by cometabolism and bioaugmentation with Pseudomonas putida for the biodegradation of 4-chlorophenol. Journal of Chemical Technology and Biotechnology, 2012, 87: 1270-1275. DOI:10.1002/jctb.v87.9 (

[15] Fang F, Han H J, Zhao Q, et al. Bioaugmentation of biological contact oxidation reactor (BCOR) with phenol-degrading bacteria for coal gasification wastewater (CGW) treatment. Bioresource Technology, 2013, 150: 314-320. DOI:10.1016/j.biortech.2013.09.119 (

[16] Wang J L, Quan X C, Wu L B, et al. Bioaugmentation as a tool to enhance the removal of refractory compound in coke plant wastewater. Process Biochemistry, 2002, 38: 777-781. DOI:10.1016/S0032-9592(02)00227-3 (

[17] AHPA. Standard Methods for the Examination of Water and Wastewater. 20th ed. Washington, D C: American Public Health Association, American Water Works Association, Water Environment Federation, 1998. (

[18] Zhu X B, Liu R, Liu C, et al. Bioaugmentation with isolated strains for the removal of toxic and refractory organics from coking wastewater in a membrane bioreactor. Biodegradation, 2015, 26: 465-474. DOI:10.1007/s10532-015-9748-z (

[19] Zhang J X, Zhang Y B, Quan X, et al. Enhanced anaerobic digestion of organic contaminants containing diverse microbial population by combined microbial electrolysis cell (MEC) and anaerobic reactor under Fe (Ⅲ) reducing conditions. Bioresource Technology, 2013, 136: 273-280. DOI:10.1016/j.biortech.2013.02.103 (

[20] Pan Y T, Ni B J, Philip L, et al. Electron competition among nitrogen oxides reduction during methanol-utilizing denitrification in wastewater treatment. Water Research, 2013, 47(10): 3273-3281. DOI:10.1016/j.watres.2013.02.054 (

[21] Lauchnor E G, Semprini L. Inhibition of phenol on the rates of ammonia oxidation by Nitrosomonas europaea grown under batch, continuous fed, and biofilm conditions. Water Research, 2013, 47: 4692-4700. DOI:10.1016/j.watres.2013.04.052 (

[22] Plüg B D, Cibati A, Trois C. The use of organic wastes at different degrees of maturity as carbon sources for denitrification of landfill leachate. Waste Management, 2015, 46: 3737-379. (
