Shuli Liu1, Guangming Zhang2,3and Jie Zhang2
(1. School of Environmental and Municipal Engineering, North China University of Water Resources and Electric Power, Zhengzhou 450000, China; 2.School of Municipal and Environmental Engineering, Harbin Institute of Technology, Harbin 150090, China;3. School of Environment and Resource, Renmin University of China, Beijing 100872, China)
Abstract:
In order to enhance the degrading protein capability of purple non-sulfur bacteria (PNSB), an effective strain, L2,was used to co-culture with Rhodobacter sphaeroides ATCC17023. The effects of added strain on protein removal of R. sphaeroides were investigated. Results showed that strain L2, being identified as Bacillus thuringiensis/cereus, had a high potential for producing protease with a production of 295 U/mL.The optimal B. thuringiensis/cereus(40 μL) could significantly increase protein degradation of R. sphaeroides. Protein removal and biomass production were improved by 483% and 67%, respectively. R. sphaeroides/total biomass production was more than 95%. Theoretical analysis revealed that R. sphaeroides syntrophically interacted with B. thuringiensis/cereus. Protein degradation of B. thuringiensis/cereus provided small molecule substrates (VFAs) for R. sphaeroides growth and cells materials synthesis.
Key words: Rhodobacter sphaeroides protein degradation protease Bacillus thuringiensis/cereus
DOI:10.11916/j.issn.1005-9113.2016.05.010
Clc Number:X703
Fund:
Shuli Liu, Guangming Zhang, Jie Zhang. Isolation, Identification of Bacillus Thuringiensis/Cereus and Its Enhancemen on Protein Wastewater Treatment by Rhodobacter Sphaeroides[J]. Journal of Harbin Institute of Technology, 2016, 23(5): 69-75. DOI: 10.11916/j.issn.1005-9113.2016.05.010.

Fund Sponsored by the National Natural Science Foundation of China (Grant No.51278489) Corresponding author Guangming Zhang, E-mail: zgm@ruc.edu.cn Article history Received: 2015-04-23
Contents Abstract Full text Figures/Tables PDF
Isolation, Identification of Bacillus Thuringiensis/Cereus and Its Enhancemen on Protein Wastewater Treatment by Rhodobacter Sphaeroides
Shuli Liu1, Guangming Zhang2,3


1. School of Environmental and Municipal Engineering, North China University of Water Resources and Electric Power, Zhengzhou 450000, China;
2. School of Municipal and Environmental Engineering, Harbin Institute of Technology, Harbin 150090, China;
3. School of Environment and Resource, Renmin University of China, Beijing 100872, China
Received: 2015-04-23
fund: Sponsored by the National Natural Science Foundation of China (Grant No.51278489)
Corresponding author: Guangming Zhang, E-mail: zgm@ruc.edu.cn
Abstract: In order to enhance the degrading protein capability of purple non-sulfur bacteria (PNSB), an effective strain, L2, was used to co-culture with Rhodobacter sphaeroides ATCC17023. The effects of added strain on protein removal of R. sphaeroides were investigated. Results showed that strain L2, being identified as Bacillus thuringiensis/cereus, had a high potential for producing protease with a production of 295 U/mL.The optimal B. thuringiensis/cereus(40 μL) could significantly increase protein degradation of R. sphaeroides. Protein removal and biomass production were improved by 483% and 67%, respectively. R. sphaeroides/total biomass production was more than 95%. Theoretical analysis revealed that R. sphaeroides syntrophically interacted with B. thuringiensis/cereus. Protein degradation of B. thuringiensis/cereus provided small molecule substrates (VFAs) for R. sphaeroides growth and cells materials synthesis.
Key words: Rhodobacter sphaeroides protein degradation protease Bacillus thuringiensis/cereus
1 IntroductionPurple non-sulfur bacteria (PNSB) can be applied to wastewater treatment for its favorable characteristics. For example, PNSB could degrade various small organic molecules by using them as hydrogen donors and carbonsources under light or dark conditions[1].So many kinds of wastewaters could be treated effectively by PNSB[2-5].During the process of removing pollutants from wastewater, PNSB also completed itscell growth. Valuable biomass containing single cell protein, bacteriochlorophylls, biopolymers, 5-aminolevulinic acid, carotenoids, and CoQ10were simultaneously produced[6-9]. Many wastewaters, including food-processing wastewater, brewing wastewater, and grain, oil processing wastewater, contain an abundance of organic molecules and nutrients. For the wastewaters above, traditional biological technologies only aimed to degrade and remove the contaminants, such as carbon, nitrogen, and phosphorus resources. Further, PNSB wastewater treatment could turn contaminants into bacterial cells and produce valuable biomass at the same time.However, one disadvantage of this technology is that PNSB cannot degrade organic macromolecules efficiently, such as protein, polysaccharose, and lipids. Most wastewaters are rich in an abundance of macromolecules, which has formed a bottleneck in the industrial application resource recycling of PNSB. Therefore, present research interest is focused on how to use PNSB to degrade macromolecule organics and recycleresources efficiently.
Many methods, including pretreatment and co-culture technology[10-11], have been explored to enhance biological degradation of certain macromolecules. In co-culture system, there were different interspecific interactions among different microorganisms.Usually added microorganism stimulated catabolic activity of the original microbial population by providing substrates, electron acceptors, electron donors, metabolic products, or enzymes. In previous studies, there were various interactions between added bacteria and the initial bacteria. Added bacteria could intensify the function of the initial bacteria by providing substrates, secreting enzymes, and changing the metabolic pathways of the initial bacteria.In various co-cultures, it was reported that Chlamydomas sp. MGA161 could provide more acetic acid and ethanol as electron donors for hydrogen production of the photosynthetic bacterium W-1S. Kawaguchi et al.[12] found that the hydrogen production of Rhodobium marinum A-501 was increased, because added Lactobacillus amylovorus processed amylase activity to produce lactic acid as an electron donor. The studies of Ding et al.[13] showed that photosynthetic bacteria syntrophically interacted with Clostridium butyricum, using acetate and butyrate to produce hydrogen. Therefore, it is attractive to find an efficient microbial strain that can enhance PNSB to treat macromolecule wastewater, achieving simultaneous wastewater purification and bio-resource recycling.
However, there are no relevant reports about enhancing macromolecule degradation by introducing microbial protease in PNSB wastewater treatment. Hence, this study aimed to isolate and achieve an efficient strain that could be applied to co-culture with PNSB to treat protein wastewater. And it aims to determine the optimal added dosage of an efficient strain and investigate the potential action mechanism on co-culture of an efficient strain and PNSB.
2 Materials and Methods2.1 Isolation and Screening of Protease Producing Strains2.1.1 Microbial isolation mediumsThe bacterial strains used in this study were isolated from a PNSB wastewater treatment process with a mixed culture. Microbial enrichment and isolation were both performed in a sterile 802 culture medium, which consists of 10 g/L polypepton, 2 g/L yeast extract, and 1 g/L MgSO4·7H2O. The pH of the medium was adjusted to 7.0.
2.1.2 Synthetic wastewaterSynthetic protein wastewater was used in this study, and its main characteristics were described as follows: the initial pH of the synthetic wastewater was around 7.0. The chemical oxygen demand (COD), total nitrogen (TN), total phosphorous (TP), and total protein contents were 7 994, 641, 34 and 5 032 mg/L, respectively. The synthetic wastewater used in the experiment was treated by filtration and autoclaving, avoiding environmental contamination (121 ℃, 30 min).
2.2 Screening for an Efficient Strain Enhancing the Protein Degradation of PNSBA strain of R. sphaeroides(ATCC17023)(the density was 6.8×108 CFU/mL at its logarithmic phase), which was obtained from the China General Microbiological Culture Collection Center, was used for protein wastewater treatment experiment. Bioreactors were 250 mL glass flasks, and they were sterilized at 121 ℃ for 30 min before use. 92 mL of wastewater and 8 mL of R. sphaeroides were added into each bioreactor. Six strains (L1, L2, L3, L4, L5 and L6) of different dosages were then added.In the control group, only R. sphaeroides was added.In the experimental groups, the volume of each strain with 20, 40, 80 and 120 μL was added. The initial concentration of R.sphaeroides was 360 mg/L, and the added strain was 0.3-1.8 mg/L.Therefore, the initial biomass in the systems was regarded as the biomass of R.sphaeroides. The bioreactors were in static cultivation at a temperature (30±1) ℃ under sterile conditions. Light and oxygen conditions were described as follows: the light intensity was controlled between 1 000-2 000 lx by adjusting the distance between the bioreactor and the incandescent lamp; the dissolved oxygen (DO) concentration in the bioreactor was kept within 0.5-1.0 mg/L, which was adjusted through an aeration pump[11].
2.3 Optimal Dosage of an Efficient Strain for Protein Degradation in PNSB Wastewater TreatmentThe operation was the same with the method in the section above. Different volumes of the best strain were 5, 10, 20, 40 and 80 μL in the experimental groups. The corresponding ratio of R. sphaeroides/added strain was 1 600, 800, 400, 200 and 100 (volume ratio), respectively.
2.4 Analysis Methods2.4.1 Measurement of water quality indexesAll water quality indexes were measured according to APHA standard methods[16]. The TN concentration was detected by a SHIMADZU-VCPNTOC Analyzer (TOC-VCPN, Shimadzu Corporation Company, Japan) and the protein concentration was measured by a Lowry method of protein determination kit (SHANGHAI LABAIDE).
2.4.2 Protease activity and biomass detectionThe protease activity of the bacterial strains was detected according to previously used methods[17]. A microscopic blood counting chamber was used to observe the number of bacteria present. The total biomass (R.sphaeroides and added strain) was measured by weighing the dry weight (10 ℃, overnight). The R.sphaeroides biomass was obtained following Zhao's method of using an Agilent 1200 HPLC (Agilent Technologies, Inc.) with a C18 column[18].
2.4.3 Identification of the strainThe best strain was selected due to its potential of protease production and its enhancement on protein degradation in PNSB wastewater treatment. The best strain was identified according to the morphological and metabolic characteristics, and 16S rDNA sequence analysis. The morphological characteristic of the bacteria was determined by using an Atomic Force Microscopic(BioscopeTM, USA). The metabolic fingerprints of the bacteria was determined according to carbon source utilization using a Biolog MicroStationTMSystem (GEN Ⅲ, BIOLOG). In this study, the acceptance of the Biolog system identification was based on the evaluation criteria[19].
In the 16S rDNA sequence analysis, DNA isolation was operated by the standard of a PowerSoil ® DNA Isolation Kit. The V3 region of the 16S rDNA was amplified by PCR using universal bacterial primers (27F, 5′-AGAGTTTGATCCTGGCTCAG-3′ and 1492R, 5′-GGTTACCTTGTTACGACTT-3′)[20]. The PCR amplification started with an initial denaturation (5 min at 94 ℃), followed by 32 cycles of denaturation (1 min at 94 ℃), primer annealing (30 s at 56 ℃) and extension (1 min at 72 ℃), and a final extension (7 min at 72 ℃). Amplified gene products were visualized on a 1.5% agarose gel under UV at 254 nm. The PCR amplified product was purified using an AxyPrepTM PCR Clean-up Kit (Axygen Biotechnology, Shanghai). PCR products were then cloned and were sequenced by Sangon sequencing company. The resulting 16S rDNA gene sequences were initially analyzed in public databases at http://blast.ncbi.nlm.nih.gov/Blast.cgi.Phylogenetic analysis of the 16S rDNA sequences was conducted using a neighbor-joining tree with MEGA 5.0.
3 Results and Discussion3.1 Effects of Different Strainson COD and Protein Removalsin PNSB Wastewater TreatmentDifferent dosages (0, 20, 40, 80 and 120 μL) of six strains (L1-L6) were added into R. sphaeroides wastewater treatment systems. After 96 h, the COD removal, protein removal, R.sphaeroides biomass ratio, and total biomass production were measured. As shown in Figs. 1(a) and 1(b), the COD removal and protein removals exhibited a similar trend. Both figures show that the COD and protein removals increases with the increases of bacterial strain dosage. Besides, compared to the control, there are no obvious effects on the COD and protein removals in the experimental groups with the addition of strains L4 and L6. The maximum COD removals are 38% and 36%, respectively when strains L4 and L6 are added, and the control is 33%. The maximum protein removals are 30% and 29% when strain L4 and L6 are added compared to the control (23%). However, when strain L1, L2, L3, and L5 is added, the COD removal and protein removal both display an increasing trend at different levels compared to the control. The COD removals show an 87%, 145%, 118%, and 52% increase in comparison to the control. Moreover, the corresponding protein removals are improved by 139%, 249%, 159%, and 166%, respectively.This demonstrates that adding the bacterial strain can increase the COD and protein removal efficiency of R. sphaeroides. Additionally, strain L2 greatly enhances the efficiency of protein degradation in R. sphaeroides wastewater treatment.
Figure 1
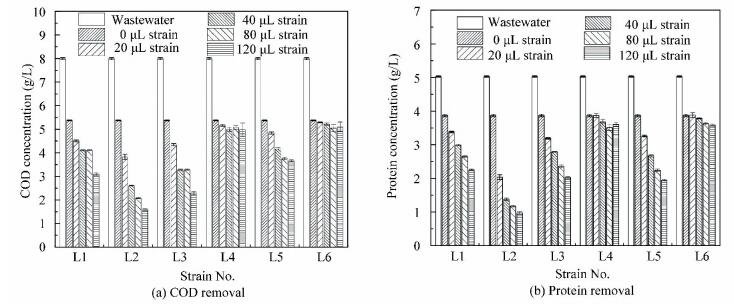
3.2 Protease Activity of Different StrainsAs shown in Fig. 2, the quantitative test of extracellular protease in liquid medium demonstrates that different strains display protease activity at varied levels. The highest protease activity (295 U/mL) is achieved by strain L2. The other three strains (strain L1, L3, and L5) have high protease activity, and the corresponding values are 129, 147 and 170 U/mL. However, strain L4 and L6 have low protease activity (45 and 42 U/mL). The results are consistent with the protein degradation in the co-culture systems (Fig. 1(b)).
Figure 2
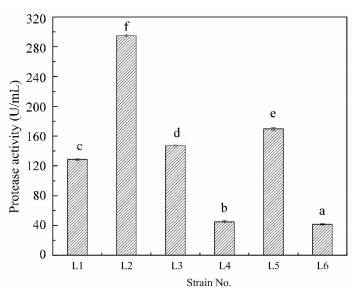
3.3 Effects of Different Strains on Biomass ProductionFigs. 1(a) and 1(b) show that the COD and protein removals increase when strain L1, L2, L3, and L5 are added, and they increases with the increase of strain dosage. The COD and protein removals are the highest when the dosage of each strain is 120 μL. The more strain is added, the higher COD and protein removals are achieved. Strain L1, L2, L3, and L5 not only increase pollutants removal, but also improve the biomass production. As demonstrated in Fig. 3, the total biomass production increases with the increase of strain dosage.
Figure 3
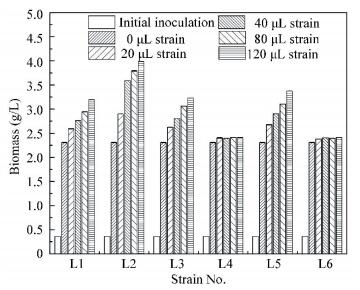
However, it is notable that R. sphaeroides biomass/total biomass increase with the increase of strain L2 dosage of when the dosage is less than 80 μL.The corresponding ratios are all greater than 80%(Table 1). The peak ratios are achieved at a dosage of 40 μL. When the dosage is 120 μL, the ratios of R. sphaer oides biomass/total biomass sharply decrease, even though the total biomass still increases. This show that strain L2 can enhance protein removal to increase R. sphaeroides biomass under the maximum extremum (dosage≤80 μL) and keep its superiority in the co-culture system. But R. sphaeroides cannot keep its superiority if strain L2 dosage is excessive ( > 80 μL). Hence, strain L2 dosage should not exceed 80 μL strain / 8 mL R. sphaeroides in the co-culture system. In addition, other strains (L1, L3, L4, L5 and L6) display no obvious advantages as strain L2.
表 1
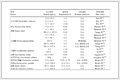
Strain No.The ratio of R. sphaeroides biomass/ total biomass
0 μL 20 μL 40 μL 80 μL 120 μL
L1 99.84±4.15 93.97±0.40 89.99±3.75 79.96±0.60 61.98±0.70
L2 99.84±4.15 97.49±0.20 98.52±1.05 95.01±3.90 79.99±0.20
L3 99.84±4.15 94.99±0.30 91.98±0.20 82.98±1.65 64.99±0.20
L4 99.84±4.15 57.55±0.75 50.74±3.60 49.87±0.15 51.70±0.80
L5 99.84±4.15 95.97±0.85 95.04±0.15 79.90±0.15 69.29±0.35
L6 99.84±4.15 64.92±0.20 59.96±0.20 60.02±4.40 58.99±0.20
Table 1 Ratio of R. sphaeroides biomass over the total biomass
From the results above, strain L2 is selected as an efficient strain for the following experiments.
3.4 Optimization of Strain L2 Dosage in Enhancing Protein Degradation by PNSB Wastewater TreatmentFrom Figs. 1, 2 and 3, strain L2 has the highest potential of enhancing protein degradation, protease activity and biomass production. It is necessary to determine its optimal dosage. As protein provides most of COD in protein wastewater (protein/ COD > 77%), the optimal dosage of strain L2 is investigated according to the protein degradation efficiency.Fig. 4 shows that the protein removal is only 14.0% in the control group. Strain additions of different dosages all increase the protein removals, and the corresponding protein removals are 35%, 47%, 58%, 82% and 79%. They show a 149%, 233%, 315%, 483% and 464% increase in comparison to the control. However, there is no obvious increase in R. sphaeroides biomass production from 40 μL to 80 μL. Therefore, the preferred addition ratio of strain L2 is 40 μL/ 8 mL R. sphaeroides.
Figure 4
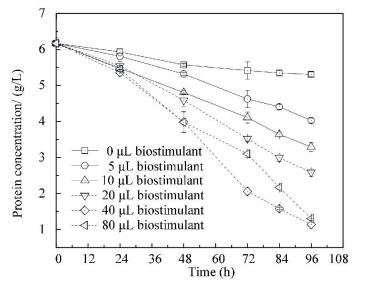
3.5 Identification of Strain L23.5.1 Morphology characteristics of strain L2The detailed morphology characteristics of strain L2 are presented by the Atomic Force Microscopic photo (Fig. 5). Strain L2 is a typical bacillus, and the diameter of a single bacterial cell is about 1.3 μm × 3.3 μm.
Figure 5
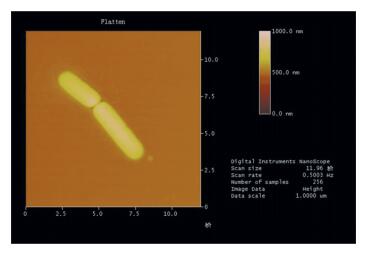
3.5.2 Carbon source utilizationThe result shows that strain L2 is identified as Bacillus thuringiensis/cereus in comparison to the typical strain in the database of the Biolog system with SIM > 0.5. Strain L2 utilizes 35 carbon sources (Table 2). With the exception of D-Glucuronic Acid utilization, strain L2 has higher metabolic activity than the type strain in the database.The higher metabolic activity shows more than 40% in the case of 14 carbon sources.
表 2
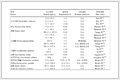
Carbon source Species
Bacillus sp.a Bacillus sp.b
Dextrin 84 88
D-Maltose 82 108
D-Trehalose 83 119
D-Cellobiose 66 67
D-Salicin 41 129
N-Acetyl-D-Glucosamine 84 125
α-D-Glucose 81 108
D-Fructose 83 109
L-Fucose 17 114
Inosine 82 82
Glycerol 75 119
D-Glucose-6-PO4 84 129
D-Fructose-6-PO4 84 152
D-Serine 77 48
Gelatin 85 135
L-Histidine 79 89
L-Serine 83 117
Glycyl-L-Proline 61 61
L-Alanine 59 81
L-Arginine 44 81
L-Aspartic Acid 57 83
L-Glutamic Acid 70 75
D-Gluconic Acid 81 123
Glucuronamide 10 156
D-Galacturonic Acid 23 100
D-Glucuronic Acid 0 139
D-Lactic Acid Methyl Ester 20 104
L-Lactic Acid 81 76
Citric Acid 58 75
α-Keto-Glutaric Acid 45 83
Acetoacetic Acid 56 119
Tween 40 59 60
Propionic Acid 37 81
Acetic Acid 54 83
Formic Acid 52 111
Values in italics indicate notable ( > 40%) differences between type and field strains; aData of type strain in the Biolog system; bData of strain L2 in this study.
Table 2 Utilization of different carbon sources by the studied strain compared to data of typical strains in the Biolog database
3.5.3 Phylogenetic analysis of strain L2As an important identification method of bacteria, determination of the phylogenetic relationship based on 16S rDNA sequencing has been widely accepted[21]. The 16S rDNA-based phylogenetic analysis results show that the sequence of strain L2 has more than a 99.0% similarity with other species of the genus Bacillus, which demonstrates that the strain belongs to this genus. The phylogenetic tree (Fig. 6) constructed from the sequence data shows the distinct relationship of strain L2 with other species within the genus. The closest phylogenetic neighbor of stain L2 is Bacillus thuringiensis (AM779000.1). The phylogenetic result of strain L2 displays high coherence with the analysis of Biolog system.
Figure 6
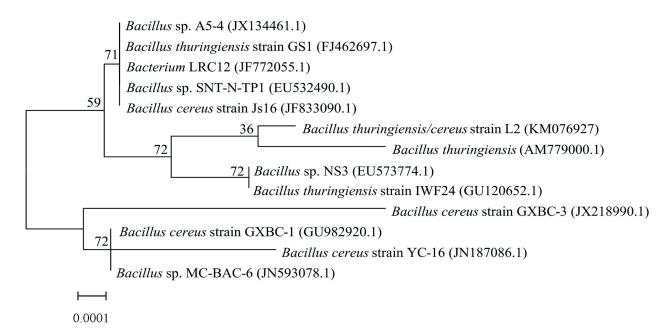
Hence, strain L2 is named “Bacillus thuringiensis/cereus L2”. Moreover, the 16S rDNA partial sequence is submitted in GenBank with accession number KM076927.
3.6 Mechanism AnalysisSeveral Bacillus species involved in protease production are B. cereus, B. sterothermophilus, B. mojavensis, B. megaterium and B. Subtilis. Moreover, Bacillus proteases are predominantly extracellular and can be secreted into the fermentation medium. Protein was degraded by proteases into small substrates. Among, volatile fatty acids (VFAs) concentrations were typically main products[22-24].
In order to investigate the mechanism of B. thuringiensis/cereus on protein degradation of R. sphaeroides further, total VFAs concentrations in wastewater were detected under different dosages of strain L2. Moreover, the corresponding ratios of VFAs in total protein were analyzed. As shown in Fig. 7, the VFAs concentrations increase with the increase of dosage when added strain L2 is not more than 40 μL. The peak value is 992.2 mg/L at the optimal dosage of 40 μL. At this condition, the corresponding ratio of CODVFAs in CODProtein is the highest of 87.2%. This shows that most macromolecule protein in wastewater is degraded into micromolecule VFAs. The results show that strain L2 has the highest protease activity in Fig. 2. VFAs are favorable for R. sphaeroides growth. Hence, at the optimal dosage (40 μL), protein in wastewater is degraded into small ones by protease producing from strain L2, which provides for the best R. sphaeroides growth.The stable and harmony interaction between B. thuringiensis/cereus and R. sphaeroides in the co-culture system enhances protein degradation efficiency, biomass production of R. sphaeroides wastewater treatment.
Figure 7
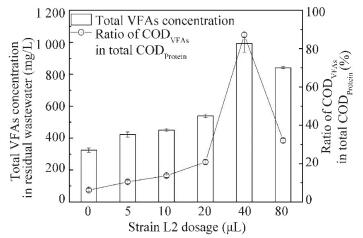
4 ConclusionsIn R. sphaeroides protein wastewater treatment, a B. thuringiensis/cereus strain L2 could successfully enhance the protein degradation efficiency and biomass production of R. sphaeroides. The optimal dosage was 40 μL, the corresponding biomass production of R. sphaeroides was 3 537 mg/L, and the protein removal was 82%, which were increased by 67% and 438%, respectively. However, excessive strain addition could not ensure the superiority of R.sphaeroides in the co-culture system.
Mechanism analysis demonstrated that trace B. thuringiensis/cereus had high protease activity (295 U/mL), which could degrade more protein into small substrates (VFAs) for R. sphaeroidesgrowth.This co-culture technology of B. thuringiensis/cereus and R.sphaeroides solved the problem that PNSB degrade protein at low efficiency.
References
[1] Staley J T.Bergey's Manual of Systematic Bacteriology.Baltimore:The Williams & Wilkins Co, 1989. 1658-1682. (

[2]Eroglu E, Gunduz U, Yucel M, et al. Photosynthetic bacterial growth and productivity under continuous illumination or diurnal cycles with olive mill wastewater as feedstock.Int J Hydrogen Energy, 2010, 35: 5293-5300.DOI:10.1016/j.ijhydene.2010.03.063(

[3]Kaewsuk J, Thorasampan W, Thanuttamavong M, et al. Kinetic development and evaluation of membrane sequencing batch reactor (MSBR) with mixed cultures photosynthetic bacteria for dairy wastewater treatment.J Environ Manage, 2010, 91: 1161-1168.DOI:10.1016/j.jenvman.2010.01.012(

[4]Tim H, Damien J B, Jürg K. Phototrophic bacteria for nutrient recovery from domestic wastewater.Water Res, 2014, 50: 18-26.DOI:10.1016/j.watres.2013.10.051(

[5]Wu P, Zhang G, Li J, et al. Effects of Fe2+ concentration on biomass accumulation and energy metabolism in photosynthetic bacteria wastewater treatment.Bioresour Technol, 2012, 119: 55-59.DOI:10.1016/j.biortech.2012.05.133(

[6]Jeya M, Moon H J, Lee J L, et al. Current state of coenzyme Q10 production and its applications.Appl Microbiol Biotechnol, 2010, 85(6): 1653-1663.DOI:10.1007/s00253-009-2380-2(

[7]Kang Z, Zhang J, Zhou J, et al. Recent advances in microbial production of δ-aminolevulinic acid and vitamin B12.Biotechnol Adv, 2012, 30(6): 1533-1542.DOI:10.1016/j.biotechadv.2012.04.003(

[8]Kien N B, Kong I S, Lee M G, et al. Coenzyme Q10 production in a 150-l reactor by a mutant strain of Rhodobacter sphaeroides.J Ind Microbiol Biotechnol, 2010, 37(5): 521-529.DOI:10.1007/s10295-010-0699-4(

[9]Kuo F S, Chien Y H, Chen C J. Effects of light sources on growth and carotenoid content of photosynthetic bacteria Rhodopseudomonas palustris.Bioresour Technol, 2012, 113: 315-318.DOI:10.1016/j.biortech.2012.01.087(

[10]Fang H H, Zhu H, Zhang T. Phototrophic hydrogen production from glucose by pure and co-cultures of Clostridium butyricum and Rhodobacter sphaeroides.Int J Hydrogen Energy, 2006, 31(15): 2223-2230.DOI:10.1016/j.ijhydene.2006.03.005(

[11]Lu H, Zhang G, Dai X, et al. Photosynthetic bacteria treatment of syntheticsoybean wastewater: Direct degradation of macromolecules.Bioresour Technol, 2010, 101(9): 7672-7674.(

[12]Kawaguchi H, Hashimoto K, Hirata K, et al. H2 roduction from algal biomass by a mixed culture of Rhodobium marinum A-501 and Lactobacillus amylovorus.J Biosci Bioeng, 2001, 91(3): 277-282.DOI:10.1016/S1389-1723(01)80134-1(

[13]Ding J, Liu B F, Ren N Q, et al. Hydrogen production from glucose by co-culture of Clostridium Butyricum and immobilized Rhodopseudomonas faecalis RLD-53.Int J Hydrogen Energy, 2009, 34(9): 3647-3652.DOI:10.1016/j.ijhydene.2009.02.078(

[14]Kirschbaum M, Schultze-Mosgau S, Pfister W, et al. Mixture of periodontopathogenic bacteria influences interaction with KB cells.Anaerobe, 2010, 16(4): 461-468.DOI:10.1016/j.anaerobe.2010.03.009(

[15]Miura Y, Saitoh C, Matsuoka S, et al. Stably sustained hydrogen production with high molar yield through a combination of a marine green alga and a photosynthetic bacterium.Biosci Biotechnol Biochem, 1992, 56(5): 751-754.DOI:10.1271/bbb.56.751(

[16] Clesscerl L S, Greenberg A E, Eaton A D. Standard Methods for the Examination of Water and Wastewater.20 th ed. Washington, D C: APHA-AWWA-WEF, 1998. (

[17]Ayaz N O. Formation of proteases from newly isolated strain isolated from Saudi Arabia.J Appl Pharm Sci, 2012, 2(8): 190-193.(

[18]Zhao W, Zhang G. Optimization of photosynthetic bacteria wastewater treatment and study of microbial species diversity.Desalination and Water Treatment, 2014, 52: 5357-5365.DOI:10.1080/19443994.2013.815688(

[19]Miller J M, Rhoden D L. Preliminary evaluation of Biolog, a carbon source utilization method for bacterial identification.J Clin Microbiol, 1991, 29(6): 1143-1147.(

[20]Moreno C, Romero J, Espejo R T. Polymorphism in repeated 16S rRNA genes is a common property of type strains and environmental isolates of the genus Vibrio.Microbiology, 2002, 148: 1233-1239.DOI:10.1099/00221287-148-4-1233(

[21]Mukherjee A K, Rai S K. A statistical approach for the enhance production of alkaline protease showing fibrinolytic activity from a newly isolated gram-negative Bacillus sp. strain AS-S20-I.New Biotechnology, 2011, 28(2): 182-189.DOI:10.1016/j.nbt.2010.11.003(

[22]Beg Q K, Gupta R. Purification and characterization of an oxidation-stable, thiol-dependent serine alkaline protease from Bacillus mojavensis.Enzyme Microb Technol, 2003, 32(2): 294-304.DOI:10.1016/S0141-0229(02)00293-4(

[23]Shumi W, Towhid H M, Anwar M N. Proteolytic activity of a bacterial isolate Bacillus fastidiosus den Dooren de Jong.J Biol Sci, 2004, 4(3): 370-374.DOI:10.3923/jbs.2004.370.374(

[24] Soares V F, Castilho L R, Bon E P, et al.High-yield Bacillus subtilis protease production by solid-state fermentation. Twenty-Sixth Symposium on Biotechnology for Fuels and Chemicals. Springer, 2005. 311-319. (
