Organic–inorganic hybrid perovskite solar cell (PSC) is a third-generation photovoltaic technology[1, 2], and the certified power conversion efficiency (PCE) has reached 25.5%
Humidity can deteriorate the performance of PSCs. In-situ encapsulation over perovskite film by using fluorine-substituted fullerene derivatives acquired excellent device stability against humidity destruction. In 2016, Jen et al. reported a fluoroalkyl-substituted fullerene derivative (DF-C60, Fig. 1) and used it as an additive to make inverted fullerene/CH3NH3PbI3 bulk-heterojunction (BHJ) PSCs[11]. DF-C60 passivated the defects of perovskite surface and facilitated charge transfer, yielding a PCE of 18.11%. The unencapsulated device demonstrated high ambient stability, after being stored under 60 ± 5% relative humidity (RH) for one month, the device kept >83% of its initial PCE. DF-C60 was further incorporated into CH3NH3Pb0.5Sn0.5I3 to make graded heterojunction (GHJ) PSCs[12]. The GHJ device offered an improved PCE (15.61%) with reduced photovoltage deficit. Additionally, the perfluoroalkyl chains in DF-C60 can effectively retard moisture penetration into Pb–Sn perovskites and also suppress Sn2+ oxidation. In 2017, novel fluorinated isoxazolino[60]fullerene (IS-1 and IS-2, Fig. 1) and pyrazolino[60]fullerene derivatives (PI-1 and PI-2, Fig. 1) were synthesized by Martín et al., and they were used to construct ETL-free PSCs[13]. The suitable LUMO energy levels contribute to the increased open-circuit voltage (Voc) and the higher PCE of CH3NH3PbI3:IS2 device, while fullerene helped to improve the photostability of the device. Two fluorinated PC61BM derivatives (3F-PC61BM and 5F-PC61BM, Fig. 1) were used as n-type additives to make CH3NH3PbI3 BHJ PSCs[14]. The incorporation of 0.1 wt% 3F-PC61BM can fill pinholes and passivate the defects to form a flat and dense BHJ perovskite film, suppressing moisture permeation under ambient condition. As a result, the device kept ~80% of its initial PCE after 550 h storage in N2, whereas the control device decayed to 80% after 240 h storage. More recently, Yang et al. developed a double-site defect passivation strategy by using a perfluoroalkyl- and pyridine-functionalized fullerene derivative (C60-PyF15, Fig. 1), which was added into CH3NH3PbI3 layer to make inverted fullerene/perovskite BHJ PSCs[15]. The device gave a PCE of 20.10%, and there was coordination between pyridine moiety and Pb2+ and also hydrogen bonding between fluorine atom and CH3NH3+. The improved hydrophobicity and suppressed ion migration endowed the device much better ambient and thermal stabilities. The device can retain 85% and 81% of its initial PCE after exposing for 1400 and 660 h under ambient condition (25 ± 5 °C, ~35% RH) and under heating at 85 °C in N2 without encapsulation, respectively.

class="figure_img" id="Figure1"/>
Download
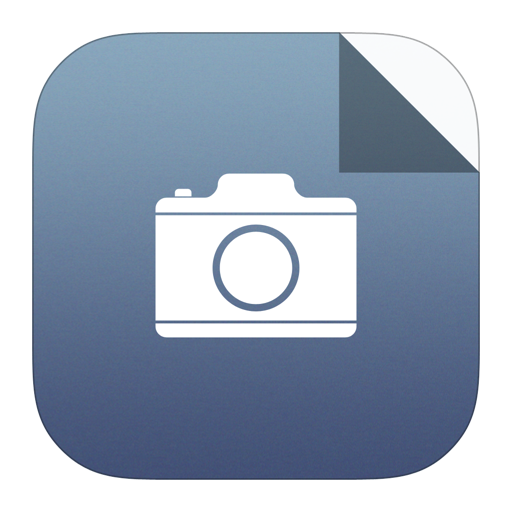
Larger image
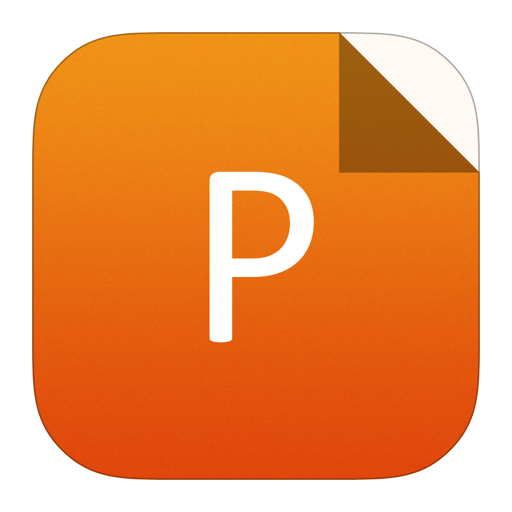
PowerPoint slide
Figure1.
Molecular structures of fluorinated and crosslinkable fullerene derivatives.
Several fluoroalkyl-substituted fullerene derivatives were combined with other fullerene materials to make a moisture-resistant mixed-fullerene interfacial layer for PSCs. In 2016, Jen et al. combined N-methyl-2-(2-perfluorooctylphenyl)-3,4-fullero-pyrrolidine (F-C60, Fig. 1) with bis-adduct of N-methyl-2-(2,3,4-tris(2-(2-methoxyethoxy)ethoxy)phenyl)-3,4-fulleropyrrolidium iodide (bis-C60) to form a robust and efficient cathode interlayer atop of PC61BM in CH3NH3PbCl3–xIx PSCs[16]. Benefitting from the hydrophobicity of F-C60, the device demonstrated a remarkable stability, retaining ~80% of its original PCE after exposing to an ambient condition (20% RH) for two weeks without encapsulation, whereas the control device with bis-C60 cathode interlayer decayed to zero under the same condition. Recently, Deng et al. reported two PC61BM analogues, [6, 6]-phenyl-C61-butyric acid 1H, 1H-trifluoro-1-ethyl ester (3F-PC61BM) and [6, 6]-phenyl-C61-butyric acid 1H, 1H-tridecafluoro-1-heptyl ester (C6F13-PC61BM, Fig. 1)[17], which were used to make hybrid ETLs with PC61BM in inverted CH3NH3PbI3- and (FA0.83MA0.17)0.95Cs0.05Pb(I0.9Br0.1)3-based PSCs, delivering PCEs of 18.37% and 19% for 1 wt% 3F-PC61BM-containing device, respectively. The upward-oriented fluorocarbon chains formed a thin moisture-resistant layer. The 1 wt% C6F13-PC61BM-containing CH3NH3PbI3 PSCs showed a much better ambient stability, keeping over 80% PCE after 33 days storage under 40%–60% RH in air. In 2020, Feng et al. synthesized 2-(perfluorophenyl)-5-phenyl-C60-fulleropyrrolidine (FP-i) and 2,5-bis-(perfluorophenyl)-C60-fulleropyrrolidine (FP-ii, Fig. 1), which were introduced as ETLs in CH3NH3PbCl3–xIx PSCs[18]. The hydrogen bonds between the organic cation of perovskite and F atom within FP-i and FP-ii, as well as the enhanced hydrophobicity led to good passivation and humidity stability, keeping 70%–85% of its initial PCE after 70 h in ambient air.
Though fullerene-based ETLs demonstrate fascinating electron transport/extraction capability and excellent electronic contact with perovskite films, the partial erosion of fullerene films by the solvents (e.g. N, N-dimethylformamide, dimethyl sulfoxide) used for processing perovskite film may yield defective film with shunting paths. Multilayer spin-coating deposition sometimes results in poor device performance. Furthermore, mechanical stress is the primary reason for mechanical failure of PSCs[19]. Introducing crosslinkable fullerene derivatives is a sound solution to these issues.
In 2016, Snaith et al. developed two crosslinkable fullerene derivatives called sol-gel C60 and PCBCB (Fig. 1) to form robust fullerene films during CH3NH3PbI3–xClx preparation[20]. Sol-gel C60 was crosslinked via hydrolysis-condensation reaction, and crosslinked PCBCB ETL was achieved by annealing at 200 °C via ring-opening reaction of benzocyclobutene moiety. Both solvent-resistant fullerene ETLs resulted in reduced shunting paths and improved hole-blocking properties. Another novel thermally crosslinkable styrene-functionalized fullerene derivative MPMIC60 (Fig. 1) was synthesized by Dauskardt et al. to replace fragile PC61BM and C60 ETL in both n–i–p and p–i–n CH3NH3PbI3 PSCs[19]. A solvent-resistant film formed by MPMIC60 exhibited 10-fold and 14-fold enhancement in fracture resistance over PC61BM and C60, respectively. The device gave higher Voc and short-circuit current density (Jsc) than PC61BM device. Later on, Hsu et al. introduced a crosslinkable [6,6]-phenyl-C61-butyric styryl dendron ester (C-PCBSD, Fig. 1) into CH3NH3PbIxCl3–x via anti-solvent method to enhance the crystallization of perovskite films and passivate the defects, as well as improving electron extraction[21]. Crosslinked network of C-PCBSD could prevent the fullerene layer being washed away by subsequent solution processing, and protect the active layer against moisture. The devices can maintain 84.6% of its initial PCE after 180 h storage in ambient (40 ± 5% RH). In 2017, Petrozza et al. used in-situ crosslinked C-PCBSD in n–i–p CH3NH3PbI3 PSCs[22], and C-PCBSD can form a robust and solvent-resistant thin film atop of TiO2 to improve the quality of upper perovskite films, promoting charge extraction. The device exhibited an enhanced PCE close to 19% with a Voc above 1.1 V. In 2018, Liao et al. further developed a composite ETL combining C-PCBSD with π-conjugated graphdiyne (GD), which was sandwiched between TiO2 and perovskite films in n–i–p CH3NH3PbI3 PSCs[23]. The GD introduced into C-PCBSD formed a face-on stacked film through a strong π–π stacking interaction. Besides, the thermally annealed C-PCBSD:GD film provided a robust and adhesive network with excellent solvent resistance to overcome interfacial erosion, which contributed to efficient charge extraction of ETL, improved crystallinity of perovskite films, leading to a PCE of 20.19% and improved stability (retaining 80% PCE after 500 h storage under ambient condition with 25%–30% RH). More recently, Marder et al. mixed PCBCB with a poly(methacrylate) derivative (CL) to form an ETL for p–i–n Cs0.05(FA0.85MA0.15)0.95Pb(I0.9Br0.1)3 PSCs, which can be thermally insolubilized[24]. The device with PCBCB:CL ETL demonstrated improved PCE (18.5%) and remarkable thermal stability, keeping 92% of its stabilized power output after 3000 h storage in N2 at 85 °C.
Another crosslinked fullerene derivative [6, 6]-phenyl-C61-butyric oxetane dendron ester (C-PCBOD, Fig. 1) via photo-curing was developed to modify TiO2 surface in n–i–p PSCs[25]. C-PCBOD was safe in the following deposition of CH3NH3PbI3 precursor solution. It could passivate the trap-states of TiO2 and facilitate the charge transfer, yielding PCEs of 15.9% and 18.3% for devices based on compact TiO2 and mesoporous TiO2 ETL, respectively. To overcome the brittleness of perovskite films, which limits the application in flexible PSCs, in 2019, Liao et al. introduced C-PCBOD as a plasticizer into CH3NH3PbI3 films to form an organic network to passivate the grain boundaries as well as the trap states within perovskite layer[26]. The rigid and flexible devices offered PCEs of 20.4% and 18.1%, respectively. Under a stretching of Δ10.0 nm/μm, the C-PCBOD-containing device retained 62% of its initial PCE, while the control device kept 42%. The robust fullerene network could prevent device decomposition in air, retaining 72% of the initial value after 960 h storage in air (25 ± 5% RH). PC61BM crosslinked by 1,6-diazidohexane (DAZH, Fig. 1) via a UV light curing was reported[27]. The crosslinked PC61BM layer demonstrated improved solvent-resistance, and the FA0.66MA0.34PbI2.85Br0.15 device gave a PCE of 18.4% for small-area and 14.9% for modules, respectively.
To check the details about above-mentioned fullerene derivatives, their application, device performance parameters, and stability, you may find Table S1 in the Supporting Information. By applying fluorinated and crosslinkable fullerene derivatives, scientists successfully improved the efficiency and stability of PSCs. More innovative fullerene materials containing fluorine and crosslinkable moieties are desired.
Acknowledgments
This work was supported by the National Key Research and Development Program of China (2017YFA0402800) and the National Natural Science Foundation of China (51925206, U1932214). L. Ding thanks the National Key Research and Development Program of China (2017YFA0206600) and the National Natural Science Foundation of China (51773045, 21772030, 51922032, 21961160720) for financial support.
Appendix A. Supplementary materials
Supplementary materials to this article can be found online at