Metal halide perovskite nanowires (PNWs) have attracted great attention not only because they have some advantages, such as long carrier diffusion length, high absorption coefficient, and low defect state density, etc.[1-3], but also because the nanowires perform efficient charge carrier transporting and possess large surface-to-volume ratio, driving PNWs to be applied in solar cells[4, 5], lasers[6], and photodetectors (PDs)[7, 8]. Up to now, the high-performance PNW devices are all based on single nanowire or nanowire arrays[7, 8]. The complexity of the preparation process, the poor reproducibility and the high cost block the large-area fabrication and commercial application.
Owing to the easy fabrication, solution-processed perovskite nanowire networks (PNNs) show the potential in commercialization compared with single nanowire or nanowire arrays counterparts[4, 9, 10]. For example, Singh et al. reported MAPbI3 PNN-based solar cells[4], indicating that the partially-grown PNNs in the active layer yielded a power conversion efficiency (PCE) of 18.7%. Besides, a PNN PD was developed by Asuo et al. via solution processing under ambient conditions[10]. By adding thiocyanate, the PDs showed a good performance with 50 μs response time (Fig. 1(a)), 0.23 A/W responsivity and specific detectivity of 7.1 × 1011 Jones at 490 nm under 2 V bias. Despite these progresses in PNN-based devices, the micro-interfaces among PNNs increased exciton transport barrier and affected the charge generation and transport, limiting the enhancement of device performance.

class="figure_img" id="Figure1"/>
Download
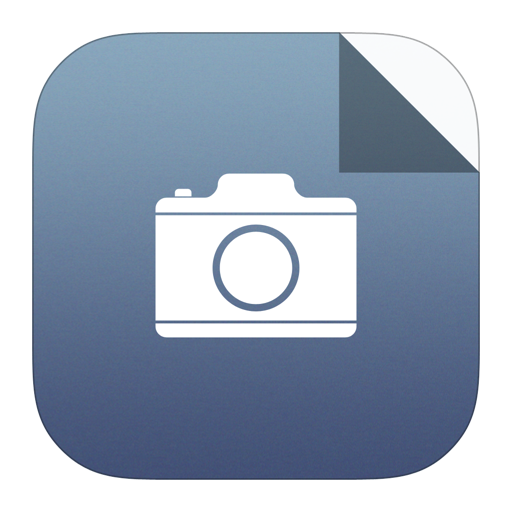
Larger image
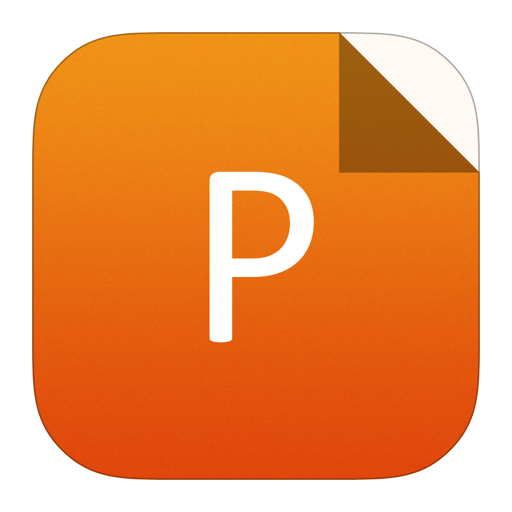
PowerPoint slide
Figure1.
(Color online) (a) SEM image for PNNs and the response time of the PDs. Reproduced with permission[10], Copyright 2018, Elsevier. (b) The schematic of a PD (top) and photograph of network PD arrays (bottom). Reprinted with permission[11], Copyright 2015, American Chemical Society. (c) Schematic of charge transfer pathways at the interface of PNNs/TMDC heterostructures upon 405 nm laser photoexcitation; side view of PNNs/TMDC heterostructure interface. Reprinted with permission[12], Copyright 2018, American Chemical Society. (d) Enhancing device performance by using the welding strategy. Reprinted with permission[13], Copyright 2020, American Chemical Society. (e) Cross-sectional SEM image for perovskite NW PD. The scale bar is 500 nm. Reprinted with permission[15], Copyright 2018, Elsevier. (f) Absorption spectra for Br-rich perovskite NWs with different Sn2+ concentrations. Reproduced with permission[14], Copyright 2020, American Chemical Society.
Deng et al.[11] reported flexible and transparent PNN PD made on poly(ethylene terephthalate) (PET) by regulating perovskite crystallization (Fig. 1(b)). The trade-off between device performance and transparency was studied. The device offered switching ratio, detectivity, and equivalent dark current derived shot noise of 300, 1.02 × 1012 Jones, and 4.73 × 10–15 A/Hz1/2, respectively. Furthermore, the perovskite network PD arrays made on PET substrates presented good flexibility. The perovskite networks protected with hydrophobic polymers improved device stability by improving surface passivation and moisture isolation.
Fang et al.[12] demonstrated mixed-dimensional van der Waals (vdW) heterostructures between one dimensional (1D) CsPbBr3 PNNs and two-dimensional (2D) transition metal dichalcogenides (TMDCs). CsPbBr3/MoS2 and CsPbBr3/WSe2 were type-I and type-II heterostructures (Fig. 1(c)), respectively. Charge transfer from CsPbBr3 to MoS2 showed an efficiency of 71%, while holes transferred from CsPbBr3 to WSe2 with an efficiency of 70%. The excellent charge transfer in 1D/2D perovskite?TMDC heterostructures enables potential application in optoelectronic devices.
For boosting device performance and stability, Wu et al.[13] demonstrated a welding strategy to enhance the crystallinity of MAPbI3 PNNs and the radial transport of charge carriers, resulting in better device performance and stability (Fig. 1(d)). The PNN PDs delivered an on/off ratio and detectivity of 2.8 × 104 and 4.16 × 1012 Jones, respectively, similar to the performance of single-NW or NW array PDs. The devices presented good stability in air (humidity 55%?65%). The flexible PNN PDs could make 1000 bending cycles with no performance deterioration.
Except PNN devices with the MSM structure, a self-powered CsPbBr3 PNN PD with a vertical structure was also reported (Fig. 1(e))[14]. Before coating the hole-transport layer, poly(methyl methacrylate) was coated onto perovskite NW film to fill the voids, passivating surface defects and reducing dark current. The devices offered a dark current density of 4.0 nA/cm2 and a photocurrent density of 22.9 mA/cm2 under a laser illumination (473 nm, 641 mW/cm2), yielding a high linear dynamic range of 135 dB. The devices also presented >106 on/off ratio, 0.3 A/W responsivity, and 1013 Jones detectivity at 0 V.
Lead toxicity retards the commercialization of lead perovskite-based devices. Tang et al.[15] used a two-step solution method for making tin-based PNNs PDs. The dark current of CsPbxSn1?x(BryI1?y)3 PNN PDs increased with the increase of tin content (Fig. 1(f)). The lowest dark current (0.672 nA at 1 V) was obtained. The best device delivered a linear dynamic range of 120 dB, a rise/fall time of 4.25/4.82 ms, and a detectivity of 2 × 1010 Jones. Moreover, Sn-based PNN devices could keep performance after being stored in air for 30 days.
The above reports clearly present the structures and the performance for PNN-based photodetectors. However, deep understanding on the performance enhancement is still required. More efforts should be put onto new materials development and device engineering.
Acknowledgements
This work was supported by the National Natural Science Foundation of China (11874143, 51972101) and the Natural Science Foundation of Hubei Province (2019CFB508). L. Ding thanks the National Key Research and Development Program of China (2017YFA0206600) and the National Natural Science Foundation of China (51773045, 21772030, 51922032, 21961160720) for financial support.