In recent years, perovskite solar cells (PSCs) have attracted tremendous attention due to their high power conversion efficiencies (PCEs) and tailorable optoelectronic properties. Up to now, the certified PCE of lab-scale PSCs has climbed up to 25.5% (see
The nucleation and crystal growth are two processes in the formation of perovskite films. The nucleation is triggered by the supersaturation of the precursor solution (Fig. 1(a))[1]. Reaching a high nucleation rate before crystal growth is critical in forming high-quality perovskite films. A variety of approaches to facilitate the supersaturation of perovskite precursor solution appeared to accelerate the nucleation[4-6]. Cheng et al. and Seok et al. simultaneously proposed the anti-solvent method[4, 7]. The introduction of an antisolvent (e.g. toluene, chlorobenzene, or diethyl ether) during the spin-coating process induced instant supersaturation of the precursor solution, causing fast nucleation for uniform, ultra-smooth perovskite thin films (Fig. 1(b)). Other solvent engineering methods were introduced to achieve a similar effect such as preparing perovskite film in an antisolvent bath[5] and adopting solvent mixture[8]. In addition to these solvent engineering methods, other strategies were also applied to instantly create supersaturation of the perovskite solute. Gr?tzel et al. reported a vacuum flash-assisted solution process (VASP) to accelerate nucleation, yielding shiny, smooth and crystalline perovskite films over large areas (Fig. 1(c))[6]. Analogously, a high-temperature thermal annealing method was developed by Kim et al. to accelerate supersaturation of the perovskite solute, obtaining a smooth and uniform film with an average grain size of 1 μm[9].

class="figure_img" id="Figure1"/>
Download
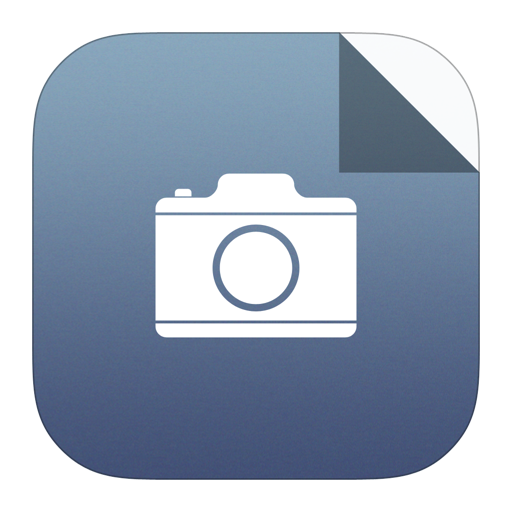
Larger image
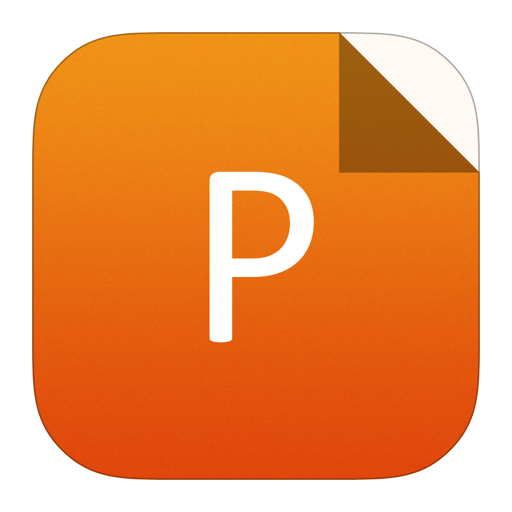
PowerPoint slide
Figure1.
(Color online) (a) Nucleation rate vs supersaturation ratio curves for homogeneous and heterogeneous nucleation. Reproduced with permission[1], Copyright 2019, American Chemical Society. (b) Antisolvent engineering method. Reproduced with permission[4], Copyright 2014, Wiley-VCH. (c) Vacuum-flash assisted solution process. Reproduced with permission[6], Copyright 2016, Science (AAAS). (d) C3-SAM-induced permanent dipole formation and involvement of the C3-SAM in perovskite crystalline structure. Reproduced with permission[12], Copyright 2015, American Chemical Society. (e) The water contact angle (left) and SEM top-view images (right) of MAPbI3 grown on varied HTLs. Reproduced with permission[13], Copyright 2015, Springer Nature. (f) Possible crystallization from nucleation sites at liquid-air interface. Reproduced with permission[15], Copyright 2018, Springer Nature.
The nucleation can be divided into homogeneous and heterogeneous modes, which have different sensitivities to the supersaturation of solution due to different nucleation energy barrier[1]. Particularly, the heterogeneous nucleation probably dominates the growth mechanism because that nucleation generally occurs on foreign surfaces or via nuclei. As the heterogeneous nucleation rate is limited by the total area of the foreign surface, this nucleation can be promoted by increasing the surface roughness of substrate or employing surface treatment. Yang et al. modeled that the heterogeneous nuclei preferred to form in the concave regions on rough substrate[10]. The perovskite films on a mesoporous structure or on a layer of nanoparticle with a proper thickness are generally more compact and uniform with less pinholes and smaller crystals.
Various methods were utilized to modulate electron-transport layers (ETLs) and hole-transport layers (HTLs). Recently, Gr?tzel et al. reported that MXene quantum dots-modified SnO2 ETL could rapidly induce perovskite nucleation in precursor solution, forming an intermediate perovskite phase upon anti-solvent treatment[11]. Chen et al. modified ZnO-coated substrate with hydrophilic 3-aminopropanioc acid (C3-SAM) in one-step perovskite deposition (Fig. 1(d))[12]. The facilitated heterogeneous nucleation on a hydrophilic substrate surface contributed to increase grain size and high crystallinity. However, in two-step perovskite deposition, the pre-nucleation of PbI2 will take place if the substrate is hydrophilic, which is unfavorable for forming high-quality perovskite films. To suppress the heterogeneous nucleation of PbI2, Huang et al. investigated the effects of hydrophobicity of various HTLs on perovskite film morphology (Fig. 1(e))[13]. With the increase of hydrophobicity of HTLs, the perovskite crystal size significantly increased from 200 nm to >1.5 μm. Moreover, appropriate substrate selection can initiate the epitaxial growth of perovskite single crystals. Switzer et al. reported epitaxial growth of CsPbBr3 single crystal on SrTiO3 substrate[14]. The chemical bonding between SrTiO3 and perovskite layer with similar crystal structures can lower the energy barrier of heterogeneous nucleation and the surface energy.
Control over the nucleation location is critical for manipulating the crystallization process, especially in 2D perovskites. Choi et al. reported a formation mechanism for vertically orientated 2D perovskite in which the nucleation arised from the liquid-air interface (Fig. 1(f))[15]. Only vertical stacking provided a direct pathway for charge transport through the perovskite, and the subsequent templated growth from heterogeneous nucleation resulted in preferential crystal orientation, boosting the efficiency of resulted solar cells. In terms of doctor blading method, Yuan et al. used hot-air blowing to accelerate DMF volatilization on precursor solution surface. The nucleation on top of solution triggered a downward growth of BA2MA3Pb4I13 perovskite with out-of-plane orientation[16]. Moreover, seeding growth is regarded as a new strategy to manipulate the morphology evolution. Heterogeneous nucleation sites are intentionally introduced into the precursor solution, which can effectively control the concentration of nucleation center. Sargent et al. developed a perovskite seeding method with Cs-containing perovskite as the seed source[17]. The perovskite seeds facilitated heterogeneous nucleation, yielding large grain and preferential crystal orientation of perovskite film.
Crystal growth occurs after the formation of stable nuclei. For obtaining efficient solar cells, high-quality perovskite films with large grains are prerequisite. Generally, slow crystallization results in high-quality perovskite films. Based on this cognition, a variety of thin-film engineering approaches are used to retard the crystallization. Lewis acid-base adduct approach is a commonly used strategy to slow down perovskite crystal growth[18]. PbI2 is a Lewis acid, which can form adduct with Lewis base molecules containing S, N and O atoms. This method was reported by Park et al., in which DMSO was chosen as the Lewis base[19]. DMSO was added into the solution of CH3NH3I and PbI2 in DMF during the dissolution (Fig. 2(a)). The Lewis acid-base adduct CH3NH3I·PbI2·DMSO was evidenced by infrared spectroscopy, eventually leading to a highly uniform MAPbI3 layer. The formation of solvated phase can retard the crystallization of 3D perovskite. Solvent with high donor number is often added into precursor solution to form stable solvated phase. Yang et al. introduced a Lewis base additive, urea, into a perovskite precursor solution, leading to increase of grain size and reduction of defect density[20]. Urea could slow down the crystal growth and passivate defects at the grain boundary (Fig. 2(b)).

class="figure_img" id="Figure2"/>
Download
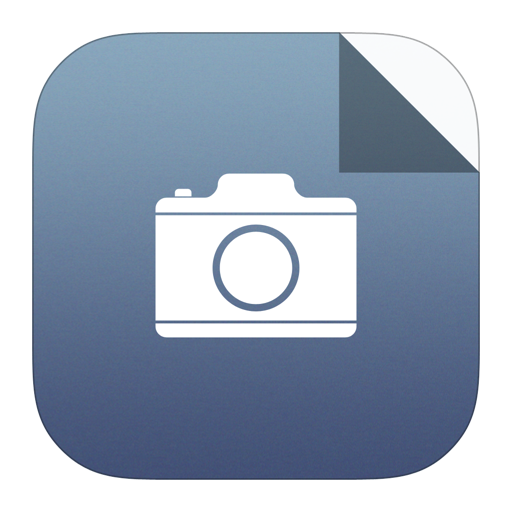
Larger image
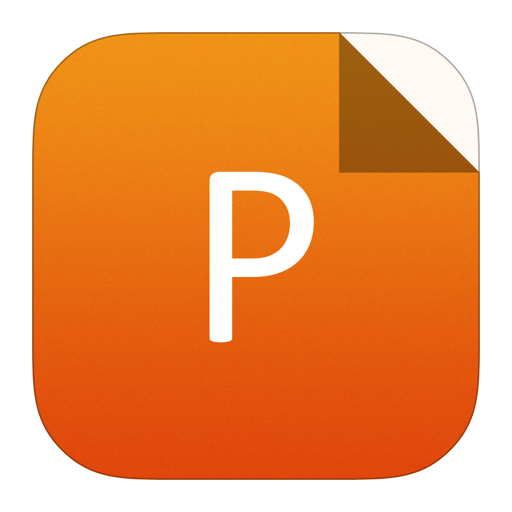
PowerPoint slide
Figure2.
(Color online) (a) The fabrication process and SEM images for MAPbI3 films made by one-step spin-coating of DMF solution with and without DMSO. Reproduced with permission[19], Copyright 2015, American Chemical Society. (b) MAPbI3 crystallization without and with urea. Reproduced with permission[20], Copyright 2017, Elsevier. (c) Illustration of the retarded crystal growth by Rb+ binding on perovskite surface. Reproduced with permission[21], Copyright 2020, Wiley-VCH. (d) Crystal growth at the crystal surface where DPSI molecules modulate the diffusion of metal ions to reach perovskite surface. Reproduced with permission[22], Copyright 2021, Springer Nature. (e) Methylamine-induced defect-healing (MIDH) of MAPbI3 films. Reproduced with permission[24], Copyright 2015, Wiley-VCH.
Moreover, additive engineering is regarded as one of the effective ways to retard the crystal growth of perovskite films. The commonly used additives include 1,8-diiodooctane (DIO), hydrohalic acids and chlorine-based additives, which can form chelation with Pb2+. Yuan et al. revealed that the incorporation of AX salts (such as NH4Cl, MACl, NH4I, MAI and MABr) into precursor solution could effectively suppress the nucleation rate of PbI2-based solvated phase, further retarding the crystal growth[16]. They also reported that the growth speed of phenylethylammonium-based Ruddlesden-Popper (RP) perovskite was gradually lowered by adding increasing amount of Rb+ ions into the precursor solution[21]. Rb+ ions accumulated at crystal growing front could be used as a superficial crystal growth inhibitor, properly retarding the adsorption and diffusion of constituent ions (Fig. 2(c)). Huang et al. introduced 3-(decyldimethylammonio)-propane sulfonate inner salt (DPSI) into perovskite precursor solution as an additive to regulate the growth of perovskite single crystals[22]. DPSI ligands anchoring with Pb2+ on perovskite crystal surfaces could tune the growth rate of different facets (Fig. 2(d)).
Ostwald ripening is another effective method to manipulate the crystal growth of perovskite film. Huang et al. reported solvent annealing to effectively increase the crystallinity and grain size of CH3NH3PbI3 film[23]. During the solvent annealing process, DMF embedded in perovskite precursor films could induce Ostwald ripening of perovskite grains. The grains with various sizes were dissolved by DMF to different degrees. Consequently, large grain coarsening occurred upon the adsorption of small grains. In addition to Ostwald ripening, other post treatments, like VASP method[6], thermal annealing[9] and CH3NH2 gas[23], were also adopted to optimize the crystal growth of perovskite film. Cui et al. reported methylamine (CH3NH2) induced defect-healing (MIDH) of CH3NH3PbI3 thin films based on ultrafast, reversible chemical reaction with CH3NH2 gas at room temperature[24]. During perovskite-gas interaction, an intermediate CH3NH3PbI3·xCH3NH2 liquid phase was formed (Fig. 2(e)). MIDH process can heal the defects in perovskite film, thus forming a dense film with high crystallinity.
In summary, modulating crystal nucleation and growth in perovskite films is extremely crucial for pushing the efficiency of PSCs to the theoretical limit. Based on this cognition, a variety of thin-film morphological engineering approaches are highlighted, including adjusting nucleation rate and nuclei density, manipulating nucleation region and retarding crystal growth. More efforts are needed to make large-area perovskite films with high quality and high performance.
Acknowledgements
L. Ke thanks the Natural Science Foundation of Hunan Province (2019JJ50776). L. Ding thanks the National Key Research and Development Program of China (2017YFA0206600) and the National Natural Science Foundation of China (51773045, 21772030, 51922032, 21961160720) for financial support.