Perovskite solar cells (PSCs) have attracted great attention due to excellent power conversion efficiency (PCE), low cost and simple solution processing. The certified PCE has reached 25.5% from the initial efficiency of 3.8%, being comparable to that of commercial crystalline silicon solar cells[1, 2]. The efficiency boosting is mainly ascribed to the excellent properties of halide perovskite materials, including suitable bandgaps, high absorption coefficient, long carrier diffusion length and high defect tolerance[3]. Moreover, through the composition and interface engineering, the operational stability of PSCs can exceed 1000 h under continuous illumination[4]. Therefore, PSCs show a great promise for commercialization.
Self-assembled monolayers (SAMs) as 2D interfacial layers in optoelectronic devices can modulate the morphology and charge transport. The molecules of SAMs consist of three parts, i.e., anchor groups, chain linkers, and terminal groups[5]. The anchor groups can anchor onto the substrates through chemical interaction to passivate the surface defects. The chain linkers establish the spacer length and build barrier layers. The terminal groups can change the interfacial properties via chemical bonding, form dipole moment to enlarge built-in potential and tune the energy level. More importantly, the terminal groups can alter the wettability of the interlayer to produce high-quality films with enhanced crystallinity and morphology.
In PSCs, SAMs were initially introduced to combine perovskite and metal oxide layers via functional groups. Usually, metal oxides (such as n-type TiO2, SnO2, ZnO, and p-type NiOx) are used as charge-transport materials due to their creditable stability, excellent transparency, and simple synthesis. And the perovskite layers are directly deposited onto metal oxide layers with ohmic contact. Undoubtedly, the bonding between perovskite and buried layers is unfirm, there are lots of interfacial defects, and the interface is chemically unstable[6]. The surfaces for most metal oxides are covered with hydroxyl (–OH) groups, which can degrade perovskite structure. In addition, the surface defects of metal oxide films further induce high charge recombination. SAM can be spontaneously formed upon immersing a metal oxide-coated substrate into a solution containing amphifunctional molecules, which can combine perovskite and buried layers and enable the interface to fulfill mutilfunction[7].
Recently, 3-iodopropyl trimethoxysilane, Si(OCH3)3(CH2)3I, with –Si(OCH3)3 anchor group, ?(CH2)3? chain linker, and –I terminal group, was designed as SAMs in SnO2-based PSCs[8]. First, the SAMs (Inset in Fig. 1(a)) can self-assemble and cross-link readily on SnO2 surface by –Si(OCH3)3 groups and react with surface –OH groups to create anchoring O–Si bonds. Second, the –I terminal groups can form electrostatic bonds with perovskite film. Third, the alkyl chain can enhance the lyophilicity for solution-deposited perovskite film. More importantly, I-termination enhances the strength of bonding with perovskite surface substantially, and it is more favorable than other halogen terminations, leading to a 50% increase in adhesion strength at SnO2/perovskite interface and enhanced mechanical reliability (1.91 ± 0.48 to 2.83 ± 0.35 J·m?2, Fig. 1(b)). However, the bonding with Si(OCH3)3(CH2)3H was weak (1.72 ± 0.54 J·m?2). Theoretically, at PbI2-terminated α-FAPbI3 (001) surface with I-SAM, there is quite strong adhesion (Figs. 1(c) and 1(d)). The iodine atoms on H(CH2)4I gain electrons from both the undercoordinated Pb2+ and coordinated I? on PbI2-terminated α-FAPbI3 (001) surface (Fig. 1d). Naturally, there is no such bond at the interface with H(CH2)4H (Fig. 1(c)). The PCE for I-SAM-based device increased from 20.2% to 21.4% mainly due to an increased open-circuit voltage, and the device showed a reduced hysteresis (1.8% for H-SAM and 2.9% for I-SAM versus 8.8% for without SAMs) (Figs. 1(e) and 1(f)), and an improved operational stability under continuous 1-sun illumination. This work indicates that smart molecular design for SAM interlayer affects the device performance significantly.

class="figure_img" id="Figure1"/>
Download
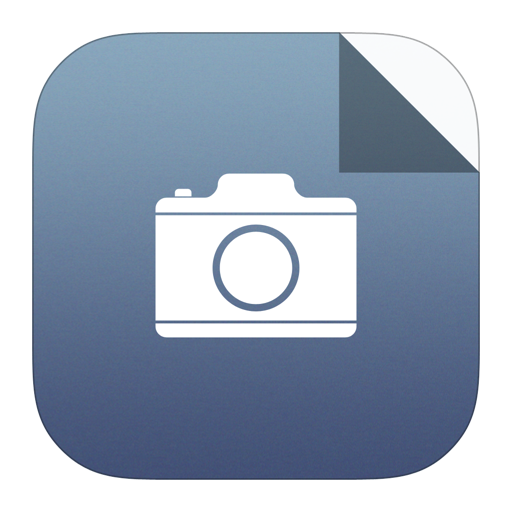
Larger image
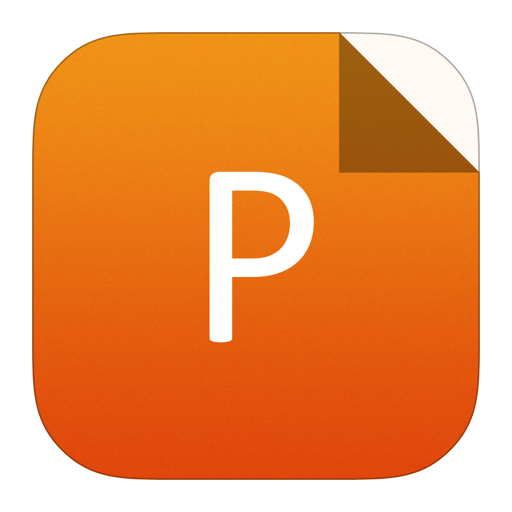
PowerPoint slide
Figure1.
(Color online) (a) Schematic illustration of the toughness testing. Inset: idealized I-SAM. (b) Toughness of SnO2/perovskite interface without SAMs and with H-SAM or I-SAM. (c) and (d) DFT calculations. Bonding between PbI2-terminated α-FAPbI3 (001) surface and H(CH2)3H (“H-SAM”) (c) or H(CH2)3I (“I-SAM”) (d). (e) The n–i–p planar PSCs with SAMs. (f) J–V curves from reverse (R) and forward (F) scans of champion PSCs without SAMs and with H-SAM or I-SAM. Reprinted with permission[8], copyright 2021, Science. (g) The effect of a surface dipole on perovskite band diagram. Reprinted with permission[12], copyright 2021, Royal Society of Chemistry.
Perovskite films are usually sandwiched between hole-transport and electron-transport layers, which extract free charge carriers. Interestingly, a charge-transport layer-free PSC was fabricated, and the SAMs made built-in potential at metal/perovskite interface[9]. The open-circuit voltage (~600 mV) is equal to the workfunction difference induced by the SAM layers. A photocurrent reaches 12.1?mA·cm?2 under 1-sun illumination. The results indicate that the SAMs can act as charge-transport layers. For instance, SAM of siloxane-functionalized fullerene molecules on FTO electrode was introduced into metal oxide-free perovskite solar cells, minimizing material consumption[10]. Recently, a novel p-type SAM was designed as hole-selective contact in p–i–n PSCs, where the terminal group with 1,3-dimethoxybenzene moiety provides a miscible interface for perovskite solution processing[11]. The new SAMs kept 80% of their initial 21% PCE for 250 h at 85 °C under 1-sun illumination.
Dipolar SAMs are rarely assembled directly on perovskite surface to optimize the energy level alignment, mainly because of the uncontrolled surface chemistry. In 2021, it was granted to tune the surface workfunction, valence and conduction bands of halide perovskite films by using SAM dipole moments[12]. The perovskite workfunction can be changed by several hundreds meV via controlling the surface coverage, while avoiding the risk of uncontrolled surface chemistry when employing strong dipolar molecules (Fig. 1(g)). The combination of the intrinsic dipole of SAM molecules and the dipole created by the interaction between the molecules and the surface causes a shift in perovskite energetics. The total dipole can point towards (positive) or outside (negative) the perovskite surface. In view of a SAM molecule with the desired binding mode and dipole direction, the workfunction of perovskite films can be shifted just by changing the deposition parameters. Further, the energy level alignment of perovskite and spiro-OMeTAD layers was characterized by combining experimental data and drift-diffusion simulations, and the effect of the workfunction shift of perovskite films on device performance was investigated.
In summary, SAMs are favorable candidates for interface engineering in PSCs. On the buried oxide layers, the SAMs can passivate defects, create more energy level alignment, modulate morphology and crystallinity of perovskite layer, enhance mechanical reliability and overcome interface redox. On the perovskite films, the workfunction can be controlled by modulating the dipole of SAMs. Moreover, SAMs can replace the charge-transport layers. Therefore, suitable SAM molecules can modify the interfaces in PSCs to enhance PCE and stability.
Acknowledgements
This work was supported by the National Natural Science Foundation of China (11804166) and the China Postdoctoral Science Foundation (2018M630587). L. Ding thanks the National Key Research and Development Program of China (2017YFA0206600) and the National Natural Science Foundation of China (51773045, 21772030, 51922032, 21961160720) for financial support.