1.
Introduction
Semiconductor heterostructures designed by high crystallization materials expand the application for modern microelectronics and optoelectronics with interesting properties[1-4]. Combining different materials to form heterostructures is crucial to manipulate the energy band in semiconductors[5-7], control the lasing dynamics[8, 9] and bring the photogenerated carrier transfer in junction areas[10, 11]. Such novel requirements vital for electro-optics would be otherwise impossible in a single material system. Over the past few decades, various methods including van der Waals (vdW) epitaxy and vapor phase epitaxy (VPE) have been used for heterostructure fabrications[12-16]. The VPE method, as a fancy and efficient technique, is widely used in IV–V, II–VI conventional semiconductor heterostructures. The epitaxial growth technology can effectively gain a high-purity phase with controlled morphology while maintaining materials' favorable intrinsic properties[17]. Promising heterostructure platforms manufactured by the VPE method realize applications for light-emitting diodes (LED)[18, 19], heterostructure transistors[20, 21], double heterostructure lasers[22], p–n heterojunction photodetectors[23] and so on. Recently, with the growing interest attracted to electronics and optoelectronics, the discovery of one class of promising materials as building blocks for heterojunction candidates becomes increasingly important.
All-inorganic cesium lead halide perovskite (CsPbX3, X = Cl, Br, I) has attracted significant exploration in optoelectronic applications, such as photodetectors[24, 25], LEDs[26], laser devices and solar cells[27, 28]. High-quality two-dimensional (2D) perovskite, especially CsPbBr3 with better stability, has been demonstrated to be a promising material for creating heterostructures. Up to now, the perovskite-based heterostructures have been focused on abundant materials, such as CsPbBr3 on layered materials (MoS2, graphene, WS2)[29-32], CsPbBr3/TiO2 nanocrystals[33] and CsPbBr3/SiO2 nanoparticles[34]. Although widespread applications for CsPbBr3-based heterostructures have led to a new approach for band-gap engineering and novel devices, the defects that are caused by lattice mismatches will limit the lifetime of photogenerated electron-hole pairs. The lattice-matching epitaxial growth of CsPbBr3 heterostructures can reduce the defect density and improve the device performance. Recently, core–shell PbSe@CsPbBr3 wire heterostructures were realized by the chemical vapor deposition method[35]. The high-quality conformal CsPbBr3 shell growth on the PbSe core contributed to the high-performance photodetectors. Liu et al. fabricated CsPbBr3/PbS nanoplate heterostructures by vapor transport[36]. Photodetectors based on vertical and lateral heterostructures exhibit clear rectifying characteristics and broadband photodetection. However, epitaxial growth of perovskite heterostructures, especially CsPbBr3 single-crystal thin film heterostructures, which is a prerequisite for the continuous improvement of electronic device performance, still remains a challenge so far.
In this study, we report high-quality CsPbBr3/PbS single-crystal film heterostructures through a two-step vapor phase epitaxy route, where orthorhombic CsPbBr3 single-crystal films epitaxially grow on cubic PbS(100) thin films. X-ray diffraction (XRD) and electron back-scatter diffraction (EBSD) were used to characterize the crystal structures and epitaxial relationships of the as-prepared heterostructures. CsPbBr3/PbS heterostructures reveal broadband light absorption and well-photogenerated electron transfer, which were confirmed by absorption and PL spectra. Owing to its unique optoelectronic properties, photodetectors based on the CsPbBr3/PbS heterostructures reveal superior responsivity, high detectivity, fast response speed and obvious rectification behavior.
2.
Experimental section/methods
2.1
Growth and transfer for PbS single-crystal films
PbS single-crystal films were first grown on NaCl (100) crystals by the chemical vapor deposition method in a quartz tube furnace. In a typical growth, 30 mg PbCl2 powder (99.95%, Alfa Aesar) and 0.5 g sulfur powder (99.95%, Alfa Aesar) were used as sources. Then, a freshly cleaved NaCl (100) crystal as the substrate was positioned on the PbCl2 powder with the surface facing down. The PbCl2 powder was placed in the center of the tube and the sulfur powder was located approximately 20 cm upstream from the heating center. Before heating, the tube was pumped down to expel the air and then purged with high-purity Ar gas. The heating center was then ramped to 580 °C with the heating rate of 30 °C/min and kept for 15 min. Finally, the tube furnace was cooled down to room temperature naturally. During the growth, a 50 sccm Ar gas was used as the carrier. Owing to the high solubility of alkali salts, epitaxial PbS films can be transferred onto SiO2/Si substrates and can maintain good integrity and crystallinity. Then the as-grown PbS films were used as the temple of CsPbBr3 epitaxial growth.
2.2
Epitaxial growth of CsPbBr3/PbS single-crystal film heterostructures
The CsPbBr3/PbS single-crystal film heterostructures were synthesized on SiO2/Si substrates through a two-step growth process in a 1 inch diameter quartz heated by a high-temperature horizontal tube furnace. For CsPbBr3/PbS single-crystal film heterostructure growth, the CsPbBr3 powder, which was synthesized via a solution-processed method[37], was used as the source to synthesize CsPbBr3 films. And the CsPbBr3 films were synthesized using a vapor phase epitaxy method, as illustrated in Fig. 1. In brief, 5 mg CsPbBr3 powder was placed at the heating center of the furnace and the as-grown PbS films were positioned downstream at about 9 cm away from the CsPbBr3 powder. The heating temperature was controlled at about 500 °C and was maintained at this temperature for 40 min. During the whole process, the quartz tube was kept at the pressure of about 10 Pa with a constant Ar flow of 60 sccm. The details can be found in our previous report[25].

class="figure_img" id="Figure1"/>
Download
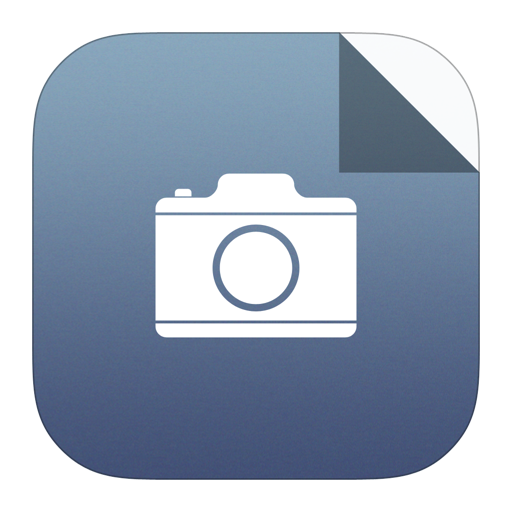
Larger image
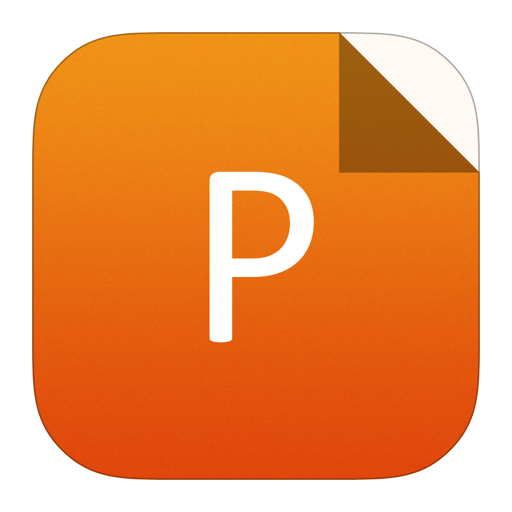
PowerPoint slide
Figure1.
(Color online) Schematic diagram of the tube furnace setup for the growth of CsPbBr3/PbS heterostructures.
2.3
Device fabrication and performance measurement
Morphologies of the sample were characterized using a Hitachi S4800 field emission scanning electron microscope. EBSD data collection was conducted in a JEOL 6500 SEM. XRD patterns were recorded via a Bruker D 8 Focus powder X-ray diffractometer using Cu Kα radiation (λ = 1.5418 ?). PL measurements were performed in a Nikon Eclipse Ti-U Inverted Microscope with a Princeton SP 2358 spectrometer and a405 nm laser was adopted as the excitation source. Steady-state absorption spectra were obtained by a Cary 5000 Ultraviolet-visible-near infrared spectrophotometer with circularlypolarized lamplight and controlled incident light using a polarizer plate.
Photodetectors based on CsPbBr3/PbS heterostructures were fabricated on the SiO2/Si substrates, which were pre-deposited Au electrodes. A copper grid shadow mask with a 200 mesh grid was covered on the surface of SiO2/Si substrates, followed by thermal evaporation of 100 nm Au as the electrodes. Then, the devices were annealed at 250 °C for 2 h in Ar atmosphere to reduce the contact resistance. Photoelectric characterizations were carried out using a Lakeshore probe station and a Keithley-4200 SCS semiconductor parameter analyzer with 450, 532, 785 and 1550 nm laser illumination under atmosphere environment.
3.
Results and discussion
PbS has a NaCl-type structure with a unit cell thickness of 0.594 nm along the [001] direction, which is close to that of NaCl crystal (0.564 nm). From the small lattice mismatch (~5.3%), it can be well inferred that PbS can grow into a single-crystal film on the NaCl-type substrate. Fig. 2(a) displays that the as-synthesized PbS films have a smooth surface with little concave textures, suggesting the growth of the PbS films followed the Volmer–Weber mode. The XRD patterns of PbS films on NaCl (100) are shown in Fig. 2(b). The well-defined peaks located at 30.1° and 62.5° were readily assigned to (200) and (400) planes of cubic PbS by comparing with the standard JCPDS card of PDF#05-0592. The other two peaks related to NaCl can be readily assigned to NaCl (200) and (400) planes (JPCDS, PDF#05-0628). There are only four peaks in the XRD patterns, indicating that there is no other additional phase in PbS films as well as lattice-matching between epilayers and substrates.

class="figure_img" id="Figure2"/>
Download
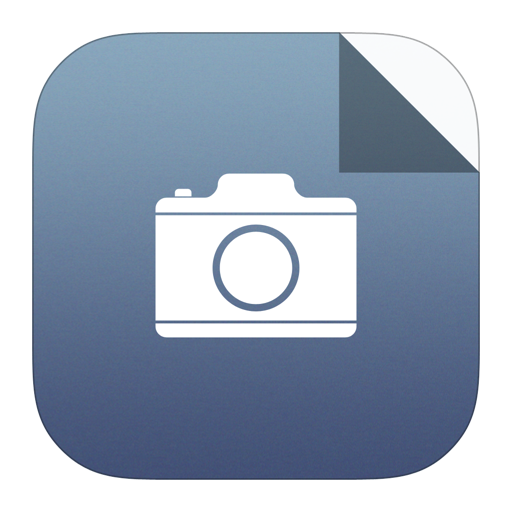
Larger image
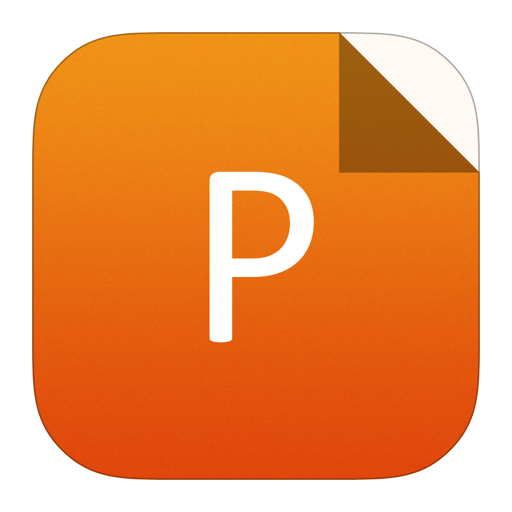
PowerPoint slide
Figure2.
(Color online) (a) SEM image of PbS single-crystal films epitaxially grown on NaCl (100) substrates. (b) XRD patterns of the PbS films on NaCl (100) crystals (red) and reference data (JPCDS, PDF#05-0592, black).
Subsequently, the pre-synthesized PbS films were transferred to SiO2/Si substrates and used as the receiving substrates for the epitaxial growth of CsPbBr3/PbS film heterostructures. Representative cross-section and top-view scanning electron microscope (SEM) images of the CsPbBr3/PbS films are displayed in Figs. 3(a) and 3(b). It can be seen that the as-grown heterostructures have a clear interface and both the upper CsPbBr3 and lower PbS films demonstrate uniformity and conformal characteristics. Some nucleation points were remained on the surface, indicating a typical Volmer–Weber growth process during the epitaxy of CsPbBr3 films as well. XRD results provide further evidence for the crystallographic orientation and crystallinity of the as-synthesized heterostructures, as shown in Fig. 3(c). The peaks related to cubic PbS can be observed of the CsPbBr3/PbS heterostructures, proving excellent crystallinity of transferred PbS single-crystalline films. The epitaxial relationship can be determined as CsPbBr3(110) // PbS(100). In our previous work, the vapor phase epitaxial CsPbBr3 films have been proved to be an orthorhombic structure at room temperature[25]. Therefore, the CsPbBr3 films in this work showed diffraction peaks at 15.21° and 30.70°, which were compared to the (110) and (220) plane of orthorhombic CsPbBr3 (ICSD #97851, space group Pbnm, a = 8.207 ?, b = 8.255 ? and c = 11.759 ?). Fig. 3(d) exhibits the magnified XRD results with three similar peaks. Besides PbS (200) and CsPbBr3 (220), the peak at 30.38° was observed and verified to be the CsPbBr3 (004) plane, which is reliable proof that the upper CsPbBr3 films have orthorhombic structure as well. The peak located at 60.9° was from Si substrates. The absence of other distinct diffraction peaks indicates that no other crystalline phases have been formed.

class="figure_img" id="Figure3"/>
Download
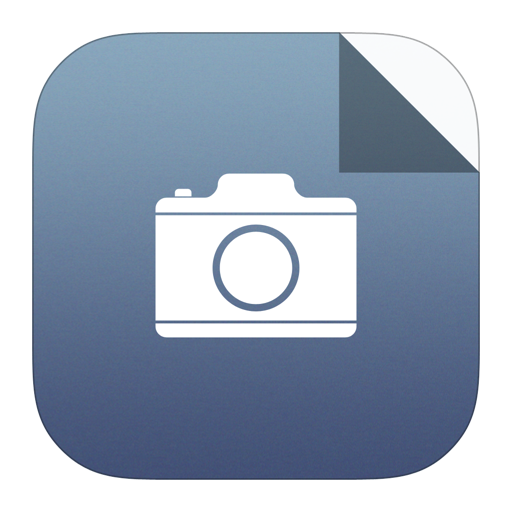
Larger image
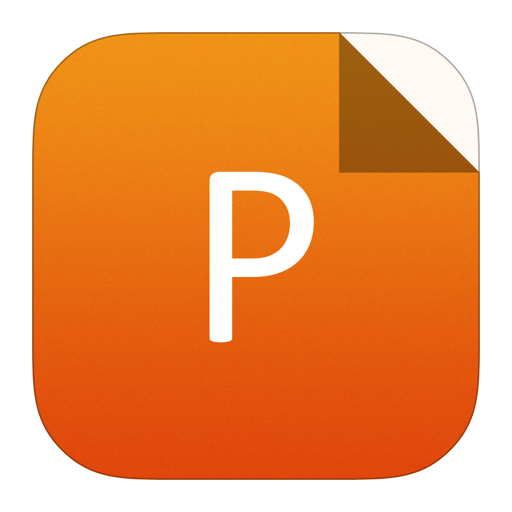
PowerPoint slide
Figure3.
(Color online) (a, b) Cross-section and top-view SEM images of CsPbBr3 films epitaxially grown on PbS (100) films. (c) XRD patterns of the CsPbBr3/PbS heterostructures and standard cards of CsPbBr3 and PbS. (d) Magnified XRD pattern in (a).
The orientation relation and crystal structure of CsPbBr3/PbS heterostructures were then characterized by EBSD. Figs. 4(a) and 4(b) are pole figures of the epilayer CsPbBr3 and PbS substrates. The sharp color contrast of the pole figures confirmed the high crystallinity of samples. Additionally, the measured pole figure of CsPbBr3 in (001)-oriented fits well with the result of the PbS (001)-oriented pole figure, proving the orientation relationship is CsPbBr3(110) // PbS(100), CsPbBr3[


class="figure_img" id="Figure4"/>
Download
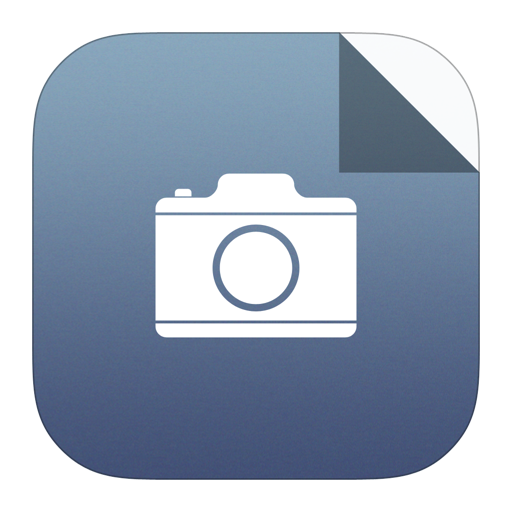
Larger image
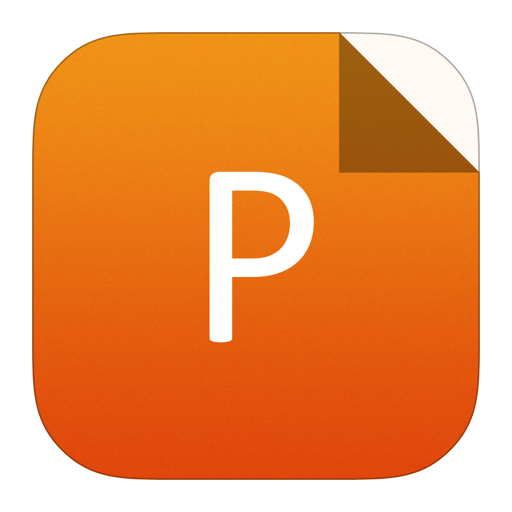
PowerPoint slide
Figure4.
(Color online) (a, b) Experimental results of pole figures from EBSD results for CsPbBr3 and PbS. (c) EBSD orientation map of the CsPbBr3 films epitaxial growth on PbS.
The spectra of UV–vis–infrared absorption and PL were used to study the absorbance and emission of the obtained single-crystal film heterostructures. Fig. 5(a) shows the absorption spectra of pure CsPbBr3 samples and CsPbBr3/PbS heterostructures which were grown under the same conditions. Compared with CsPbBr3, the heterostructures exhibit broad-spectrum absorption from the visible to mid-infrared region, which makes it an appealing material for optoelectronics[36, 38]. Fig. 5(b) shows the PL spectra for the pristine CsPbBr3 films and the CsPbBr3/PbS heterostructures, measured under the 405 nm laser excitation with the same intensity. It is evident that the PL results of heterostructures have strong quenching at the 524 nm wavelength, which is similar to the heterostructures with photogenerated carrier transfer processes[39]. This phenomenon also can be related to the transfer of energy from CsPbBr3 to PbS, which hinders the recombination of photoexcited electron-hole pairs.

class="figure_img" id="Figure5"/>
Download
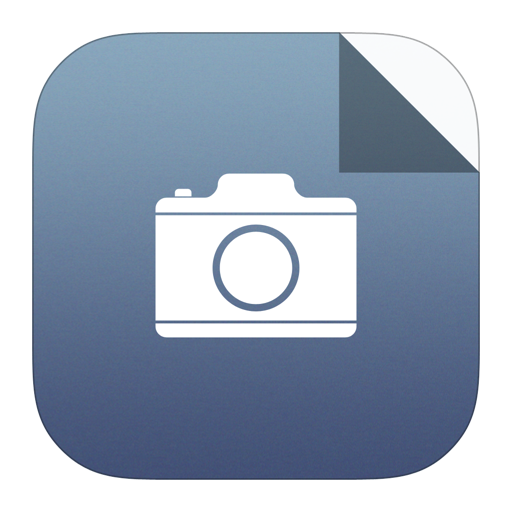
Larger image
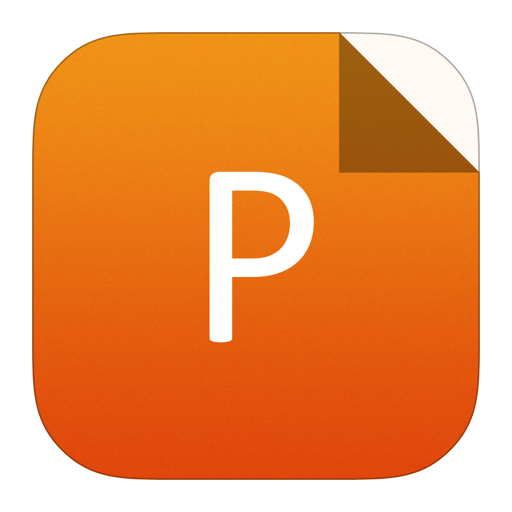
PowerPoint slide
Figure5.
(Color online) (a) UV–visible–IR absorption spectra and (b) PL spectra of pristine CsPbBr3 and CsPbBr3/PbS heterostructures.
The photodetectors based on CsPbBr3/PbS single-crystal film heterostructures were directly fabricated on SiO2/Si substrates with pre-deposition Au electrodes. The inset of Fig. 6(a) shows the schematic model of the device. The as-fabricated devices were then annealed at 250 °C in an Ar atmosphere to improve contact between heterostructures and electrodes and reduce resistance. Fig. 6(a) exhibits the current–voltage (I–V) curves of the photodetectors under 450 nm laser illumination with the light intensity varying from 0 to40 mW/cm2. In contrast to the dark current, the photocurrent
m{ph}} $

m{ph}}}} = {I_{{
m{illuminated}}}} - {I_{{
m{dark}}}}$


class="figure_img" id="Figure6"/>
Download
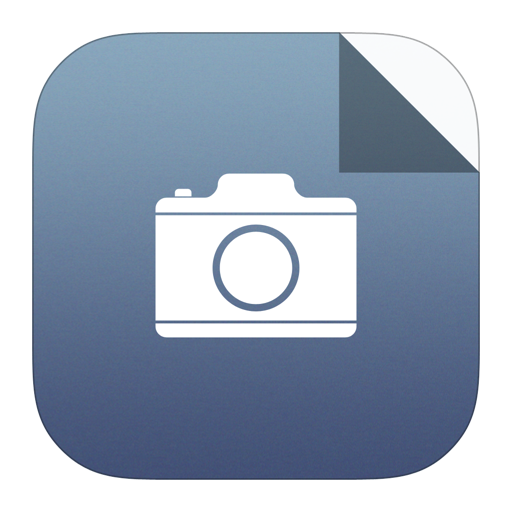
Larger image
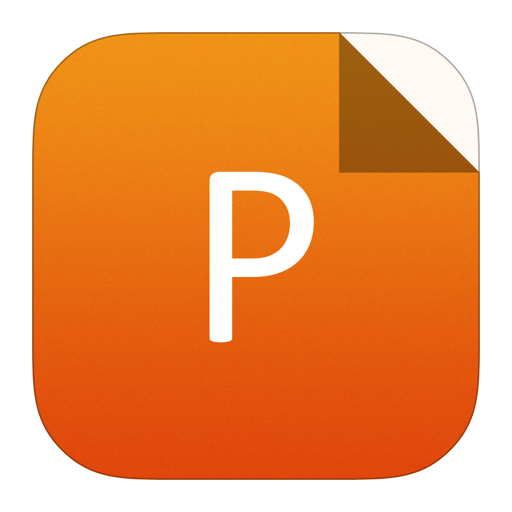
PowerPoint slide
Figure6.
(Color online) Photodetector based on CsPbBr3/PbS film heterostructures. (a) I–V curves of the film photodetector in the dark and under 450 nm laser illumination with different light intensities. The inset is the schematic diagram of the device. (b) Light intensity dependence of the photocurrent at the bias of 5 V and corresponding fitting curve. (c, d) Time-resolved photoresponse and the enlarged portion of the device at the bias of 5 V and light intensity of 40 mW/cm2.
Fig. 6(b) shows the light intensity dependence of the photocurrent. By fitting the experimental data, the photocurrent can be expressed by a power-law equation
m{ph}}} propto {P^{0.92}} $


m{ph}}}/PS$

m{m}}}^{2} $

m{ph}}}/PS propto {P^{0.92}}/PS = {P^{ - 0.08}}/S$


m{ph}} cdot {S}^{1/2}}{P S{ left(2e{I}_{
m{dark}}
ight)}^{1/2}}$



m{rising}}}} = $

m{decay}}}} = $

In previous studies, CsPbBr3 shows p-type conductivity without intentional doping while PbS has n-type conductivity[41, 42]. Therefore, the as-grown CsPbBr3/PbS film heterostructures are expected to form p–n heterojunctions. To investigate the optoelectronic properties of the CsPbBr3/PbS heterostructures, Au electrodes were redeposited on the surface of CsPbBr3 and the bottom of PbS via the same method. The schematic model of the CsPbBr3/PbS p-n heterojunction photodetector is shown in Fig. 7(a). The 532, 785 and 1550 nm laser is used as incident light to illuminate the CsPbBr3/PbS p–n junction region. Fig. 7(b) shows the I–V curves of the photodetector under dark and light irradiation. The results show obvious rectification behavior from the visible to the infrared, indicating that a well-defined p–n junction is formed at the interface of the CsPbBr3/PbS heterojunction. In general, the narrow bandgap materials like PbS induce a high dark current[43]. The CsPbBr3/PbS devices have a dark current of value ~10–9 A, which is lower than that of single PbS photodetectors, attributed to the CsPbBr3 as an electron barrier layer in the heterostructures[35, 44].

class="figure_img" id="Figure7"/>
Download
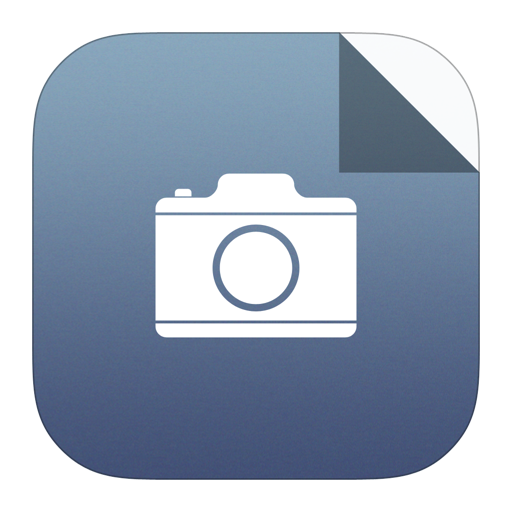
Larger image
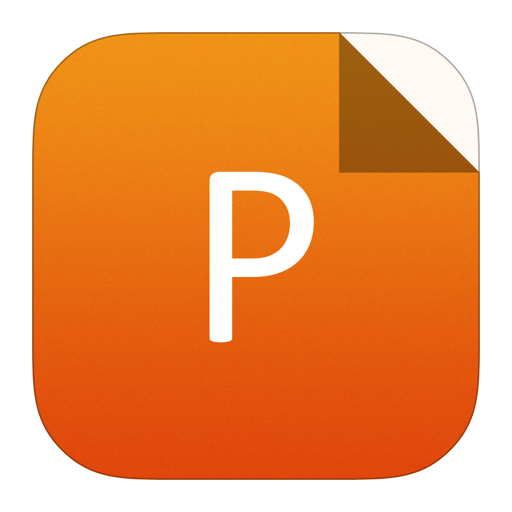
PowerPoint slide
Figure7.
(Color online) (a) Schematic diagram of the CsPbBr3/PbS p–n heterojunction photodetector under laser illumination. (b) Dark current and photocurrent of the CsPbBr3/PbS heterostructure detectors under a 532, 785 and 1550 nm light with a power density of 40 mW/cm2.
4.
Conclusion
In summary, CsPbBr3/PbS single-crystal film heterostructures have been achieved via vapor phase epitaxy. Both the as-synthesized PbS and CsPbBr3/PbS heterostructure films have uniform surface and excellent crystallinity. The epitaxial relationship between the CsPbBr3 epilayers and PbS substrates investigated in detail using XRD and EBSD. The absorption and PL spectra have revealed wide light absorption and photogenerated electrons transfer optical properties of the heterojunctions. Photodetectors based on the CsPbBr3/PbS heterostructures exhibit good light absorption conversion efficiency, fast response speed of ~96 ms, high detectivity of ~2.65×1011 Jones, superior photoresponsivity of 15 A/W, good stability and repeatability. In addition, the devices based on CsPbBr3/PbS p–n heterojunctions show a clear rectification behavior, indicating the construct of functional low-dimensional architectures and a new generation of wide-spectrum optoelectronic devices.
Acknowledgements
This work was supported by the Natural Science Foundation of China (Grant No. 11704389), Scientific Equipment Development Project and Youth Innovation Promotion Association Project of Chinese Academy of Sciences.