Organic solar cells (OSCs) have received considerable attention and demonstrated great potential as flexible, lightweight, semitransparent, and low-cost energy sources. Flexible OSCs have practical applications in wearable electronics, portable chargers for back bags and tents, solar airships, etc. Many efforts have been made to improve the performance of flexible OSCs, including the development of flexible transparent electrodes, new organic materials, and optimization of the device structure. Progresses have been achieved in the last few years, and power conversion efficiencies (PCEs) of 16.1%[1], 16.5%[2], 13.61%[3], and 10.09%[4] for 0.04 cm2 single-junction device, 0.04 cm2 tandem device, 1 cm2 single-junction device, and 25 cm2 module were reported.
Flexible transparent electrodes are the essential components for flexible OSCs, which should possess high electrical conductivity, transparency, and excellent mechanical flexibility. Flexible ITO is the most widely used transparent electrode. Because of the brittleness, high sheet resistance and high cost of flexible ITO electrodes[5], cost-effective and flexible transparent electrodes are still highly needed. Various ITO-free electrodes, such as metal mesh, metal nanowires, graphene, carbon nanotube, were developed and applied in flexible OSCs. Fig. 1 shows the comparison of the conductivity and light transparency of various transparent electrodes. The metal electrodes, including the thin metal, metal grid, and metal nanowire have good conductivity. However, the thin metal can give a relative low transmittance around 40%–60%. The carbon-involved transparent electrode, i.e. CNT and graphene, and PEDOT:PSS electrode have good optical transmittance around 70%–80%. However, they show relative lower electrical conductivity with sheet resistance as high as 100 Ω/□. Overall, among these electrodes, metal grid and Ag nanowires are among the best choices due to their good average visible transmittance (AVT 80%) and relatively low sheet resistance (Rs ~10 Ω/□) [6].

class="figure_img" id="Figure1"/>
Download
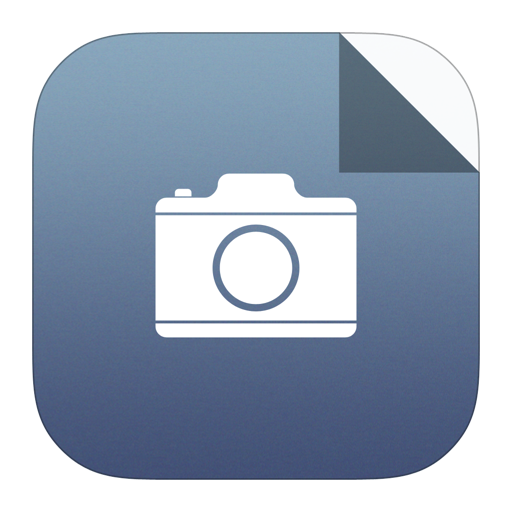
Larger image
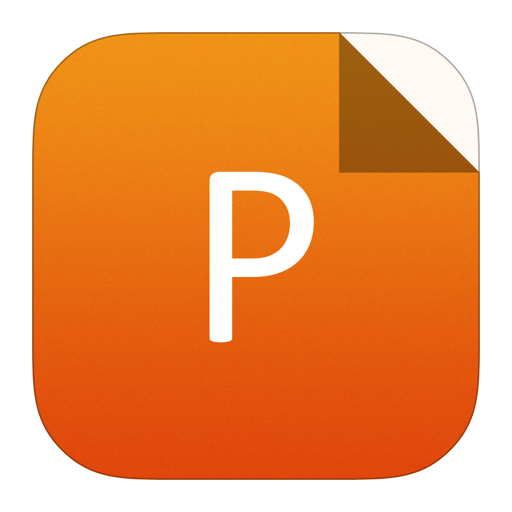
PowerPoint slide
Figure1.
(Color online) Transparency and sheet resistance of the transparent conducting electrodes (reproduced with copyright permission from SPIE publisher)[6].
The metal mesh electrodes were made by thermal evaporation[7], flexo printing[8], or inkjet printing[7, 9]. These mesh electrodes have lower sheet resistance of several Ω/□. However, the light transmittance was relatively low (< 80%) because the grid width are usually larger than 10 μm. In contrast, the metal mesh electrodes made by nanoimprinting method have advantages both in conductivity and transparency. The line width of the metal mesh can be reduced to < 3 μm, which is invisible to the naked eyes and ensures a good transparency. This electrode showed an average transmittance > 85%. Since a thick Ag layer (~ 3 μm) was embedded in the substrate, the electrode has excellent conduction with sheet resistance < 5 Ω/□. The performance of OSCs is highly sensitive to the conductivity of the transparent electrode, especially when the cell area is > 1 cm2[10], so this type of electrode is quite suitable for large-area flexible solar cells. Chen et al. first reported the use of metal grid electrodes in flexible OSCs[11], and an efficiency of 5.85% was achieved for a 1.21 cm2 OSC[10]. Following this, Tan[12] and Wu et al.[13] reported OSCs with these electrodes. To smooth the surface of the silver nanogrid and to increase the conductivity of the final electrode, Su et al. developed new silver nanogrid/copper (Ag-NG/Cu) or silver nanogrid/Ni composite electrode by applying Cu or Ni electroplating and the following surface polishing steps[14], and a lowest sheet resistance of 0.03 Ω/□ was obtained. The highest figure of merit (FOM) reached 8 × 104[15]. Ma et al. reported the flexible OSCs with Ag-NG/Cu electrode. By modifying electrode surface, power conversion efficiencies (PCEs) of 8.75%, 7.79% and 7.35% for 2.4, 4.0, and 9.0 cm2 cells were achieved, respectively, which are higher than that of devices with flexible ITO electrode (6.61% and 5.88% for 2.4 and 4.0 cm2 cells, respectively) (Fig. 2)[16]. The Ag/Cu composite grid-involved flexible cells could maintain 91.7%, 81.7%, and 77.0% efficiency of the 1 cm2 cell as the area increased to 2.4, 4.0, and 9.0 cm2, respectively, while the efficiency for flexible ITO device decreased to 74.6% (for 2.4 cm2) and 66.4% (for 4 cm2) of the 1 cm2 cells, clearly demonstrating that lowering the sheet resistance of the transparent electrode is helpful in achieving high performance for large-area solar cells.

class="figure_img" id="Figure2"/>
Download
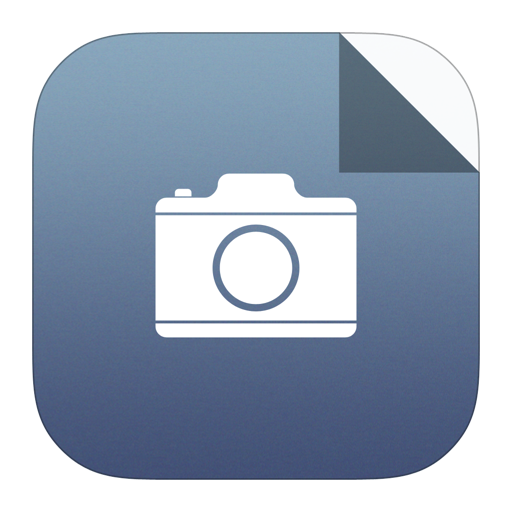
Larger image
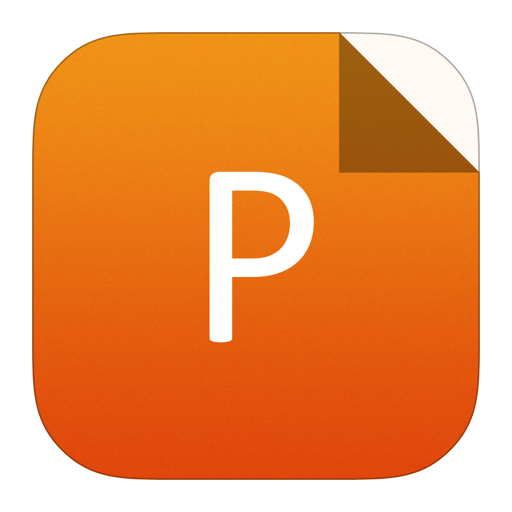
PowerPoint slide
Figure2.
(Color online) (a) Photographs of the large-area flexible OSCs. (b) J–V characteristics and (c) EQE spectra of the large-area flexible solar cells with PET/Ag/Cu grid electrodes. (d) J–V characteristics and (e) EQE spectra of the large-area flexible solar cells with PET/ITO electrodes (reproduced with copyright permission from Wiley-VCH)[16].
Very recently, Wei et al. reported flexible OSC module with Ag-NG electrode via slot-die coating (Fig. 3). Owing to the difference in film formation kinetics between spin-coating and slot-die coating, controlling of the film morphology is critical for large-area fabrication. By applying hot substrates and non-halogen solvent, a PCE of > 10% was achieved for the printed cell with an area of 25 cm2, which is the highest PCE for a flexible cell with > 10 cm2 area.

class="figure_img" id="Figure3"/>
Download
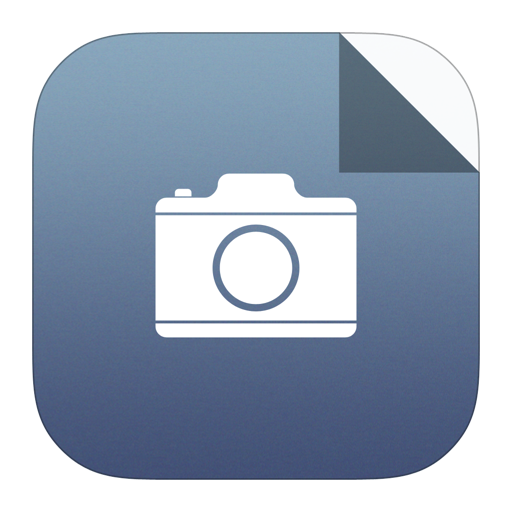
Larger image
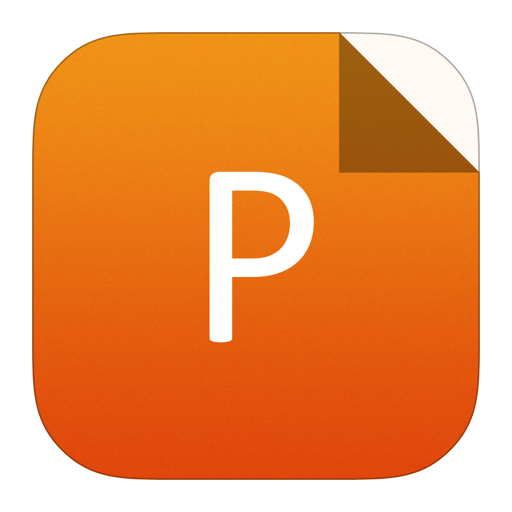
PowerPoint slide
Figure3.
(Color online) (a) Sketch of spin coating and slot-die coating. (b) The small-area rigid device and large-area flexible device. (c) The chemical structures of PTB7-Th, PC71BM, and COi8DFIC. (d) Optical microscopy and SEM images of the PET/silver-grid substrate. (e) Comparison of this work with reported PCEs for flexible devices made by slot-die coating (reproduced with copyright permission from Wiley-VCH)[4].
In addition to silver nanogrid electrode, nanowires (AgNW) networks are also good candidates for the preparation of large-area flexible solar cells due to their balanced light transparency and conductivity. Both AgNW and AgNW composite electrodes[17] were prepared through various solution-processed methods, including spin-coating[18, 19], slot-die coating[20], brush printing[21]. The sheet resistances of AgNW electrode are generally 10–20 Ω/□, and transmittance can be as high as 85%–92%. The conductivity of AgNW electrode is determined by the connection of Ag nanowires. The removal of polyvinylpyrrolidone (PVP) surfactant and the welding of the nanowire can improve the conductivity[22]. Choy et al. developed an one-step multifunctional chemical treatment for AgNW/PEDOT:PSS composite electrode[23].
ITO is widely used in optoelectronic devices. The flexible ITO electrode is not good at mechanical and conductive properties. Though the sheet resistance of ITO can reach to 10 Ω/□, the flexible ITO has higher sheet resistance (40–60 Ω/□). The use of flexible ITO electrode in OSCs with area > 1 cm2 was rarely reported, especially in recent years. Tables 1 and 2 show the development of flexible OSCs (> 1 cm2 single cells and the flexible modules). A PCE of 5.25% for 80 cm2 flexible OSC modules with flexible ITO electrode was reported[24].
Year | Area (cm2) | PCE (%) | Electrode | Device structure | Fabrication technique | Ref. |
2005 | 1.2 | 1 | PEDOT:PSS | PEDOT:PSS/α-NPD/C60/BCP/Mg:Al | Spin coating | [36] |
2007 | 10 | 1.6 | ITO | PET/ITO/PEDOT:PSS/P3HT:PCBM/Al | Doctor blading | [37] |
2009 | 1 | 2.7 | ITO | PET/ITO/ZnO/P3HT:PCBM/PEDOT:PSS/Silver | Screen printing | [38] |
2010 | 4 | 1.93 | Ag grid | PEN/Ag grid/HC-PEDOT/P3HT:PCBM/LiF/Al | Spin coating | [39] |
2013 | 1.21 | 1.36 | Ag grid | PET/Ag grid/PH1000/PEDOT:PSS-4083/P3HT:PCBB-C8/LiF/Al | Spin coating | [11] |
2014 | 1.21 | 5.85 | Ag grid | PET/Ag grid/PH1000/ZnO/PFN/PTB7:PC71BM/MoO3/Ag | Spin coating | [10] |
2015 | 4 | 7.09 | Ag | PET/Ag/PFN/PTB7-Th:PC71BM/MoO3/Ag/MoO3 | Spin coating | [34] |
2017 | 1.25 | 8.28 | Ag grid | PET/Ag grid/PTB7-Th:p-DTS(FBTTH2)2:PC71BM/MoO3/Ag | Slot-die | [40] |
2018 | 2.03 | 7.6 | Ag/TiOx | Ag/TiOx/ZnO/PTB7-Th:ITIC/PEDOT:PSS | Doctor blading | [41] |
2019 | 1 | 12.26 | Ag/Cu grid | PET/Ag/Cu grid/E100/ZnO/PBDB-TF:IT-4F/MoO3/Al | Spin coating | [16] |
2020 | 1 | 13.6 | Ag NWs | PET/Ag NWs/ZnO/PM6:Y6/MoO3/Al | Spin coating | [3] |
Table1.
The performance data for large-area flexible OSCs (> 1 cm2) (Fig. 5).
Table options
-->

Download as CSV
Year | Area (cm2) | PCE (%) | Electrode | Device structure | Fabrication technique | Ref. |
2005 | 1.2 | 1 | PEDOT:PSS | PEDOT:PSS/α-NPD/C60/BCP/Mg:Al | Spin coating | [36] |
2007 | 10 | 1.6 | ITO | PET/ITO/PEDOT:PSS/P3HT:PCBM/Al | Doctor blading | [37] |
2009 | 1 | 2.7 | ITO | PET/ITO/ZnO/P3HT:PCBM/PEDOT:PSS/Silver | Screen printing | [38] |
2010 | 4 | 1.93 | Ag grid | PEN/Ag grid/HC-PEDOT/P3HT:PCBM/LiF/Al | Spin coating | [39] |
2013 | 1.21 | 1.36 | Ag grid | PET/Ag grid/PH1000/PEDOT:PSS-4083/P3HT:PCBB-C8/LiF/Al | Spin coating | [11] |
2014 | 1.21 | 5.85 | Ag grid | PET/Ag grid/PH1000/ZnO/PFN/PTB7:PC71BM/MoO3/Ag | Spin coating | [10] |
2015 | 4 | 7.09 | Ag | PET/Ag/PFN/PTB7-Th:PC71BM/MoO3/Ag/MoO3 | Spin coating | [34] |
2017 | 1.25 | 8.28 | Ag grid | PET/Ag grid/PTB7-Th:p-DTS(FBTTH2)2:PC71BM/MoO3/Ag | Slot-die | [40] |
2018 | 2.03 | 7.6 | Ag/TiOx | Ag/TiOx/ZnO/PTB7-Th:ITIC/PEDOT:PSS | Doctor blading | [41] |
2019 | 1 | 12.26 | Ag/Cu grid | PET/Ag/Cu grid/E100/ZnO/PBDB-TF:IT-4F/MoO3/Al | Spin coating | [16] |
2020 | 1 | 13.6 | Ag NWs | PET/Ag NWs/ZnO/PM6:Y6/MoO3/Al | Spin coating | [3] |
Year | Area (cm2) | PCE (%) | Electrode | Device structure | Fabrication technique | Ref. |
2005 | 16.8 | 0.04 | PEDOT:PSS | PET/PEDOT:PSS/MDMO-PPV:PCBM/Al | Doctor blading | [42] |
2007 | 17.1 | 1.5 | ITO | PET/ITO/PEDOT:PSS/P3HT:PCBM/Al | Doctor blading | [37] |
2008 | 53 | 2.52 | ITO | PET/ITO/ PEDOT:PSS/P3HT:PCBM/ LiF/Al | Spin coating | [43] |
2009 | 120 | 2.1 | ITO | PET/ITO/ZnO/P3HT:PCBM/PEDOT:PSS/Silver | Screen printing | [38] |
2013 | 66 | 1.6 | Ag grid | PET/Ag grid/PEDOT:PSS/ZnO/P3HT:PCBM/PEDOT:PSS/Ag grid | Slot-die | [44] |
2014 | 8 | 3 | Ag grid | PET/Ag grid/PEDOT/ZnO/PDTSTTz-4:PCBM/PEDOT/Ag | Slot-die | [45] |
2016 | 35 | 4.2 | FTO | PET/FTO/PBTZT-stat-BDTT-8:PCBM/PEDOT:PSS/Ag | Slot-die | [46] |
2017 | 10.5 | 6.5 | Ag | PES/Ag/PEI/P3HT:ICBA/PEI:m-PEDOT:PSS/PTB7-Th:PCBM/PEDOT:PSS/Ag grid | Spin coating | [47] |
2019 | 15 | 8.9 | ITO | PET/ITO/ZnO/active layer (PTB7-Th: PC71BM or PBDB-T: ITIC)/MoO3/Ag | Slot-die | [27] |
2020 | 25 | 10.09 | Ag grid | PET/Ag grid/ZnO/PTB7-Th:COi8DFIC:PC71BM/MoO3/Ag | Slot-die | [4] |
Table2.
The performance data for flexible OSC modules (Fig. 5).
Table options
-->

Download as CSV
Year | Area (cm2) | PCE (%) | Electrode | Device structure | Fabrication technique | Ref. |
2005 | 16.8 | 0.04 | PEDOT:PSS | PET/PEDOT:PSS/MDMO-PPV:PCBM/Al | Doctor blading | [42] |
2007 | 17.1 | 1.5 | ITO | PET/ITO/PEDOT:PSS/P3HT:PCBM/Al | Doctor blading | [37] |
2008 | 53 | 2.52 | ITO | PET/ITO/ PEDOT:PSS/P3HT:PCBM/ LiF/Al | Spin coating | [43] |
2009 | 120 | 2.1 | ITO | PET/ITO/ZnO/P3HT:PCBM/PEDOT:PSS/Silver | Screen printing | [38] |
2013 | 66 | 1.6 | Ag grid | PET/Ag grid/PEDOT:PSS/ZnO/P3HT:PCBM/PEDOT:PSS/Ag grid | Slot-die | [44] |
2014 | 8 | 3 | Ag grid | PET/Ag grid/PEDOT/ZnO/PDTSTTz-4:PCBM/PEDOT/Ag | Slot-die | [45] |
2016 | 35 | 4.2 | FTO | PET/FTO/PBTZT-stat-BDTT-8:PCBM/PEDOT:PSS/Ag | Slot-die | [46] |
2017 | 10.5 | 6.5 | Ag | PES/Ag/PEI/P3HT:ICBA/PEI:m-PEDOT:PSS/PTB7-Th:PCBM/PEDOT:PSS/Ag grid | Spin coating | [47] |
2019 | 15 | 8.9 | ITO | PET/ITO/ZnO/active layer (PTB7-Th: PC71BM or PBDB-T: ITIC)/MoO3/Ag | Slot-die | [27] |
2020 | 25 | 10.09 | Ag grid | PET/Ag grid/ZnO/PTB7-Th:COi8DFIC:PC71BM/MoO3/Ag | Slot-die | [4] |
Spin-coating, slot-die coating and brush printing are not pre-patternable, and a laser scribing process is needed for making a structured electrode. Very recently, Ma et al.[3] reported the preparation of large-area patterned AgNW electrode through a gravure printing process. Fig. 4(a) shows the schematic diagram of gravure printing process. The printing process contains three steps: (1) the doctor blade forces the ink to fill the gravure cavities; (2) the ink is transferred from the cavities of the gravure roller to the substrate; (3) ink leveling on the substrate. The AgNW electrode can be easily patterned by using a pre-patterned gravure roller. In addition, gravure printing is extremely suitable for large-scale fabrication because of its high printing speed. To meet the requirement of gravure printing, the ink needs to be optimized to have suitable surface tension, volatilization rate, and viscosity. After careful ink formulation and printing process optimization, the gravure-printed AgNW electrode showed a high light transmittance of 95.4% (excluding PET substrate) and low sheet resistance of ~ 10 Ω/□ with excellent homogeneity. An efficiency of 13.61% (certified 12.88%) was achieved for 1 cm2 cells with printed AgNW electrode (Fig. 4(b)). Interestingly, the cells with gravure-printed AgNW electrode (AgNW-GV) presented much narrower PCE distribution than the cells with spin-coated AgNW electrode (AgNW-SP), which might be due to the homogenously distributed cavities.

class="figure_img" id="Figure4"/>
Download
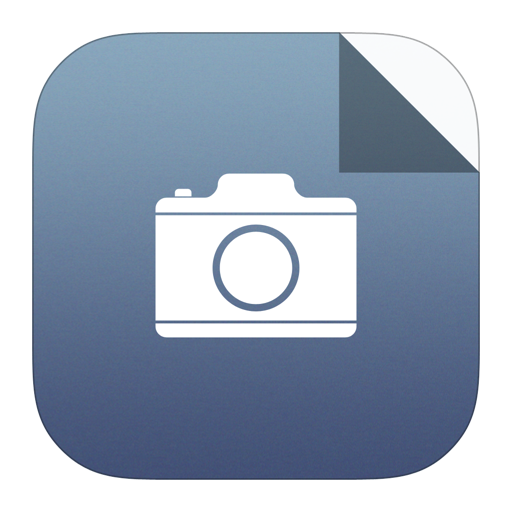
Larger image
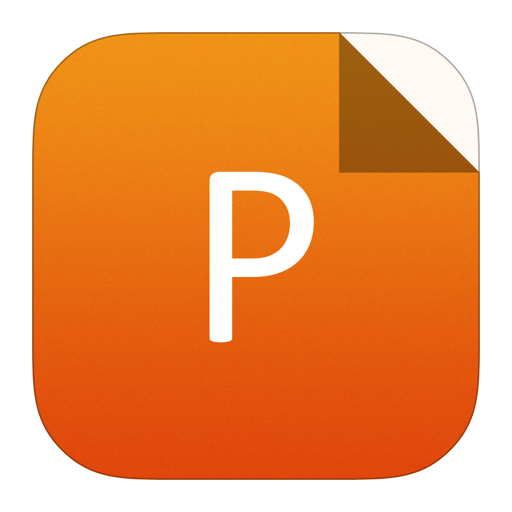
PowerPoint slide
Figure4.
(Color online) (a) Schematic diagram for the high-speed gravure printing process used to print silver nanowire electrodes. (b) Efficiency distribution diagram for the devices with PET/AgNWs-GV, PET/AgNWs-SP, and glass/ITO electrodes (reproduced with copyright permission from Wiley-VCH)[3].
Since the first report on flexible OSCs in 2004, the efficiency for small-area flexible OSCs (< 1 cm2) has increased from ~1% to 16.5%. The efficiency of middle-sized cells (≥ 1 cm2) is lower than that of the small cells (Fig. 5), due to the unavoidable increase of series resistance of the electrode. Interestingly, PCEs of both small and middle-sized flexible OSCs increase fast after 2016, which is due to the development of high-performance non-fullerene acceptors IT-4F[25] and Y6[26], and also the development of high-performance flexible metal-nanogrid and nanowire electrodes. From Tables 1 and 2, the large-area flexible single OSCs and the flexible OSC modules usually used Ag nanowire and Ag grid as the flexible electrodes, demonstrating the great potential of these flexible electrodes in upscaling flexible OSCs. Higher PCE is expected to be achieved in next few years with the fast development of transparent electrodes and new methods for preparing flexible solar modules[24, 27].

class="figure_img" id="Figure5"/>
Download
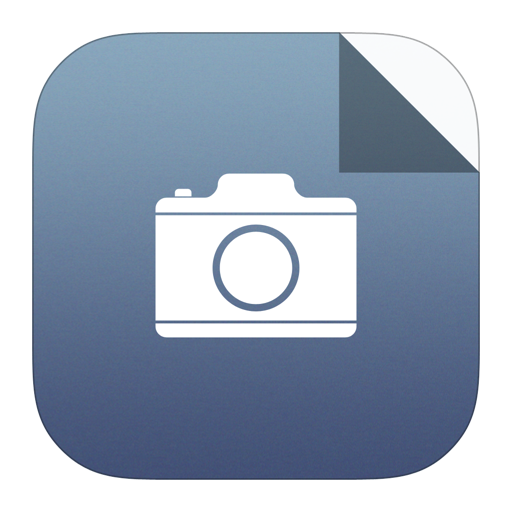
Larger image
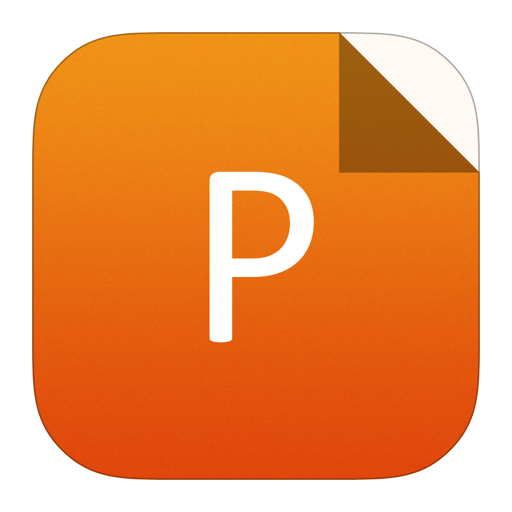
PowerPoint slide
Figure5.
(Color online) The PCEs for small-area OSCs[1, 2, 28–35], large-area OSCs (>1 cm2)[3, 10, 11, 16, 36–41], and flexible OSC modules[4, 27, 37, 38, 42–47].
Many advances have been made in flexible OSCs in the last few years, and > 10% PCE was realized for the flexible solar modules, moving one big step toward the real application of flexible OSCs. However, there exist two critical issues: scaling-up and encapsulation. As the layer thickness and nanomorphology of the photoactive layer might be different at different sites (e.g. the edge vs the center), a good way to control the drying process of the printed organic thin films is very important. Sequential deposition technology[48], temperature control on solution and substrate[49], etc were applied in slot-die coating and blade coating. For the upscaling, the homogeneity controlling is another challenge. The homogeneity is not only affected by the drying process, but also by the interface contact[50]. Thus, the electrode, the quality of the interface layer and the active layer should be well controlled. Gravure printing could be a promising method for making homogeneous large-area OSCs. Very recently, Kim et al.[51] reported the preparation of flexible perovskite solar cells, indicating the potential of gravure printing. As for thin film encapsulation, experiences from OLED industry could be helpful, where iterative organic/inorganic thin films showed excellent blocking function to water vapor and oxygen. Thin polymer layers ensure a good flexibility for the encapsulation film, while the condense inorganic layer act as barrier against water vapor and oxygen. Applying all these strategies well, we expect that flexible OSC modules will be in the market soon.
Acknowledgements
We thank National Natural Science Foundation of China (51773224), the Youth Association for Promoting Innovation (CAS) (2019317), and the Strategic Priority Research Program of Chinese Academy of Sciences (XDA09020201) for financial support. L. Ding thanks the National Key Research and Development Program of China (2017YFA0206600) and the National Natural Science Foundation of China (51773045, 21772030, 51922032, 21961160720) for financial support.