Inorganic perovskites (CsPbX3, X = halide anion), containing no volatile organic cations, exhibit better thermal stability than organic–inorganic hybrid perovskites[1, 2]. Among inorganic perovskites, α-CsPbI3 (cubic phase) possesses a relatively suitable bandgap of ~1.7 eV. CsPbI3 perovskite solar cells (PSCs) have demonstrated power conversion efficiencies (PCEs) over 20%[3-5]. However, either in ambient atmosphere or in glovebox, α-CsPbI3 tends to transform into a non-photoactive δ-phase (orthorhombic phase) at room temperature, leading to degraded device performance[6-8]. Replacing I– with smaller Br– can stabilize the cubic phase due to the increased Goldschmidt tolerance factor. Yet the bandgap will increase from ~1.7 eV (CsPbI3) to ~2.3 eV (CsPbBr3), resulting in insufficient light absorption[9-12]. Therefore, taking both phase stability and light-harvesting ability into account, CsPbI2Br (bandgap ~1.9 eV) becomes a superior choice for solar cells compared with other inorganic perovskites[13]. In the past 5 years, many efforts have been devoted to enhancing the performance of CsPbI2Br PSCs, such as interface engineering[14, 15], element doping[16], crystallization optimizing[17, 18], and additive engineering[19-21]. Inspiringly, PCEs over 17% were achieved for CsPbI2Br PSCs[22, 23]. However, CsPbI2Br films are usually prepared by spin-coating, which wastes precursor solution and is not compatible with fast and continuous manufacturing. Therefore, spin-coating is not suitable for low-cost and high-productivity industrial production. Blade-coating was used to prepare CsPbI2Br films, but the PCE is below 15%[24]. Recently, high-quality organic–inorganic hybrid perovskite films were made by drop-coating[25-27]. Unlike spin-coating, the substrate in drop-coating method is motionless, leading to different film-forming conditions and film quality. Drop-coating to make inorganic perovskite films has not been investigated yet. In this work, we used drop-coating to make CsPbI2Br films. Isopropanol (IPA) was added into the precursor solution to improve the wettability of the solution, resulting in improved film morphology, reduced trap states, and enhanced performance of PSCs. A PCE of 16.27% was achieved.
The preparation of CsPbI2Br films by drop-coating is illustrated in Fig. 1(a). A drop of precursor solution is dropped onto a preheated substrate, the solution can spread spontaneously, forming a round wet film, which is dried by N2 blowing. Only 1 μL CsPbI2Br solution is needed for a 1.49 × 1.49 cm2 substrate. Unlike the solution of 2D perovskites, the spreading of CsPbI2Br solution is nonuniform, leading to poor film quality (Fig. S1). Adding a small amount of IPA into CsPbI2Br solution can improve the wettability of the solution, leading to improved film quality (Fig. S1).

class="figure_img" id="Figure1"/>
Download
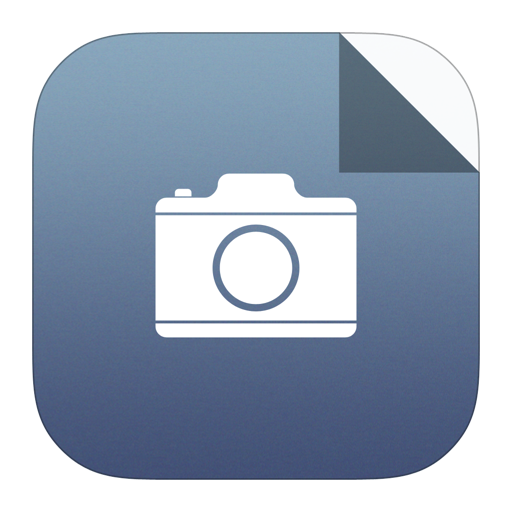
Larger image
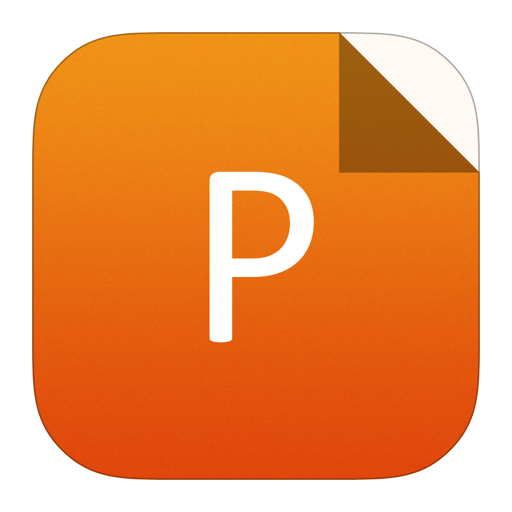
PowerPoint slide
Figure1.
(Color online) (a) Illustration for the drop-coating process. (b) XRD patterns for CsPbI2Br films made without or with IPA. (c) SEM image for the film made without IPA. (d) SEM image for the film made with IPA. (e) Dark I–V curves for the electron-only devices made without or with IPA. (f) J–V curves for the best cells made without or with IPA.
The X-ray diffraction (XRD) patterns for CsPbI2Br films are shown in Fig. 1(b). The film presents three main peaks at 14.7°, 20.9°, and 29.8°, assigned to the (100), (110), and (200) planes of α-phase CsPbI2Br, respectively. The film made from the solution with IPA has smaller full width at half maximum (FWHM) for the (100) and (200) peaks (Table S1), indicating improved crystallinity. Moreover, the much higher intensity ratios of the peaks (Table S2) for the film prepared from the solution with IPA suggest a higher degree of preferred orientation, which is beneficial for charge transport[28, 29].
The film morphology was studied by scanning electron microscopy (SEM) (Figs. 1(c) and 1(d)) and atomic force microscopy (AFM) (Fig. S2). Some pinholes (highlighted by red circles in Fig. 1(c)) were observed in the film made from the pristine solution. The pinholes could become shunt pathways in solar cells, thus decreasing photocurrent. The film made from the solution with IPA was pinhole-free, with larger grain, leading to less grain boundaries and reduced charge recombination. The film made from the solution with IPA presents reduced root-mean-square (RMS) roughness (11.1 nm) than that of the film made from the pristine solution (25.8 nm). The film made from the solution with IPA shows higher absorbance (Fig. S3(a)) due to the improved coverage and crystallinity, and it presents a bandgap of 1.91 eV (Fig. S3(b)).
The trap-state density was studied by using the space-charge limited current (SCLC) method. Electron-only devices with the structure of ITO/SnO2/ZnO/CsPbI2Br/PC61BM/Ag were made, and the dark current was measured (Fig. 1(e)). The films made with or without IPA show trap-filled limit voltages (VTFL) of 0.14 and 0.30 V, respectively, corresponding to a trap-state density of 6.37 × 1015 and 1.12 × 1016 cm?3, respectively. The reduced trap-state density results from the enhanced crystallinity, increased grain size, and improved film morphology, leading to enhanced photoluminescence (PL) and prolonged PL lifetime (Fig. S4).
Solar cells with a structure of ITO/SnO2/ZnO/CsPbI2Br/D-PTAA/MoO3/Ag (Fig. S5) were made to investigate the photovoltaic performance. The charge-transport layers and CsPbI2Br layer can be seen clearly from the cross-section SEM image of the device (Fig. S6). The amount of IPA in the solution and the drying temperature were optimized to obtain the best device performance (Tables S3 and S4). Compared with the cells made without IPA, the average PCE for the cells made with IPA increased from 13.92% to 15.57%, and the reproducibility was improved obviously (Fig. S7), which is due to the improved CsPbI2Br film quality. The best PCE of the cells increased from 15.15% to 16.27% (Fig. 1(f)), mainly due to the enhanced Jsc and FF. The external quantum efficiency (EQE) was also improved (Fig. S8), due to the enhanced absorbance and reduced trap states in CsPbI2Br films. The improved film morphology can reduce charge recombination and facilitate charge transport, leading to enhanced Jsc[30-32]. An integrated current density of 15.08 mA/cm2 is obtained from the EQE spectrum of the best cell, consistent with the Jsc (15.68 mA/cm2) from J–V measurement. PSCs via spin-coating were also made for comparison, which gave a lower PCE of 15.18% (Fig. S10 and Table S5).
In summary, drop-coating was used to make CsPbI2Br perovskite films. The wettability of CsPbI2Br solution was improved by adding IPA, leading to increased crystallinity, improved film morphology, reduced trap states, and enhanced solar cell performance. The best device delivered a PCE of 16.27%.
Acknowledgements
We thank the National Key Research and Development Program of China (2017YFA0206600), the National Natural Science Foundation of China (51773045, 21772030, 51922032 and 21961160720), and the Fundamental Research Funds for the Central Universities (2020CDJQY-A055) for financial support.
Appendix A. Supplementary materials
Supplementary materials to this article can be found online at