Organic solar cell (OSC) has attracted great interests due to its potential applications[1–9]. To date, 18% power conversion efficiency (PCE) has been achieved in single-junction OSC[10-13], indicating the feasibility of commercialization. This photovoltaic technology currently faces the performance gap between laboratory cells and large-area modules. The development of high-PCE devices with scalable coating/printing methods is critical. Most of the high-PCE OSCs made with coating/printing methods are processed with hazardous halogenated solvents and additives[14-16]. In mass production, the use of halogenated solvents will pose safety, health, and environmental issues. Non-halogenated solvents (e.g. o-xylene) are considered as "green" solvents to replace conventional solvents. However, the realization of high-PCE OSCs processed with green solvents is via spin coating, rather than coating and printing methods[17]. When using different solvents and different deposition methods, the solvents present different removal rates, and the optimal morphology is quite different[18-20]. It is still challenging to make high-PCE OSCs with green solvents through coating and printing methods[21-25]. Previously, Li et al. reported a polymer donor PTQ10 (Fig. S1)[26, 27]. In this work, we used a PTQ10’s derivative (PHT4) and acceptor IT-4Cl (Fig. 1(a)) to make blade-coated OSCs from o-xylene. We investigated the vacuum-assisted drying from o-xylene solution (Fig. 1(b)). A PCE of 13.4% was achieved. When PM6:Y6:PC71BM solar cells[28] were made through the same process, the PCE reached 17.01% (certified 16.45%).

class="figure_img" id="Figure1"/>
Download
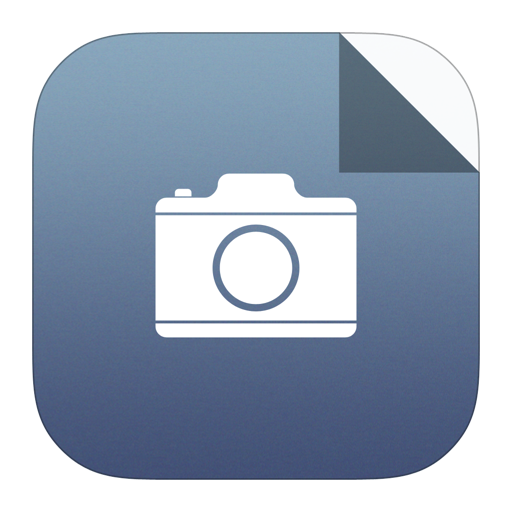
Larger image
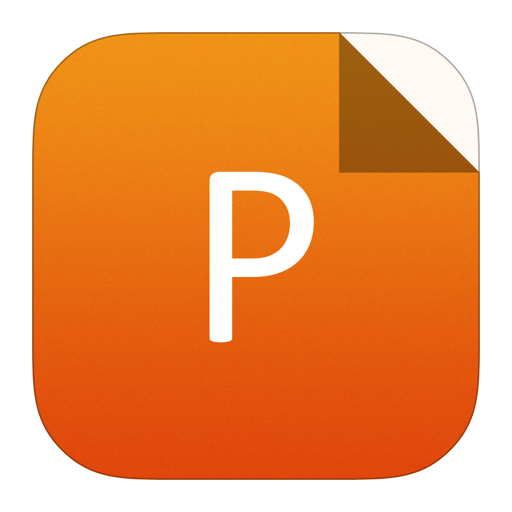
PowerPoint slide
Figure1.
(a) Chemical structures of PHT4 and IT-4Cl. (b) Schematic of the blade-coating process with (below) and without (top) vacuum-drying. (c) Light reflectance vs drying time curves for PHT4:IT-4Cl films. (d) AFM images (size: 4 × 4 μm2). (e) Line-cut profiles of GIWAXS along the out-of-plane and in-plane directions. (f) J–V curves for devices made with and without vacuum-drying.
To describe how vacuum-assisted drying affects the dynamic drying process of the active layer[29], we used an in situ transient monitoring technique to track the drying process of PHT4:IT-4Cl film. The drying time was greatly reduced from 524 to 12 s by vacuum treatment (Fig. 1(c)). According to atomic force microscopy (AFM) images in Fig. 1(d), the topography for PHT4:IT-4Cl film is significantly affected. Granular topography was observed in the film dried in air. The surface of the film dried in vacuum is much smoother. The root-mean-square roughness is reduced from 21.9 to 5.6 nm. A smoother surface suggests fine phase separation, which might favor exciton spliting[30].
Fig. 1(e) presents the line-cut profiles of grazing incidence wide-angle X-ray scattering (GIWAXS) patterns (Fig. S2). For both cases, a prominent peak located at 1.8 ?–1 is found in out-of-plane profile, which comes from the π–π stacking of molecules. The corresponding d-distance is 3.4 ?. Both pristine PHT4 and IT-4Cl have π–π stacking peak at 1.8 ?–1 (Fig. S3). For the film dried in vacuum, the out-of-plane line-cut profile shows a slightly stronger π–π stacking signal than the film dried in air, the corresponding coherence length (CL) increased from 25.6 ? (air) to 27.8 ? (vacuum). The stronger π–π stacking favors charge transport[12], thus improving short-circuit current density (Jsc).
The solar cells with a structure of ITO/ZnO/PFN-Br/PHT4:IT-4Cl/MoO3/Ag were made. The energy level alignment in the device is shown in Fig. S4. The blade-coated active layer dried in air gave a PCE of 7.4%. When vacuum-assisted drying was applied, the cell gave a much higher PCE of 13.4% (Fig. 1(f) and Table S1). The external quantum efficiency (EQE) increased over entire spectrum (Fig. S5). The EQE reaches to 83% at ~ 790 nm, which corresponds to the response of IT-4Cl (Figs. S6 and S7). The integrated Jsc from EQE is 22.54 mA/cm2, which is consistent with the measured Jsc. The increase of Jsc could result from the finer phase separation and better charge transport. The Voc for PHT4:IT-4Cl device almost remains unchanged. The rectification ratio (at ± 2 V) for device in dark was increased by about one order of magnitude compared to that dried in air (Fig. S8).
When using the vacuum-drying method, the charge carrier lifetime (τ) in the device was extended from 3.8 to 12.7 μs, which was deduced from transient photovoltage (TPV) data (Fig. S9(a)). The average carrier lifetime (τavg) calculated from impedance spectroscopy (IS) is consistent with that from TPV, and it was prolonged from 4.68 to 7.39 μs (Fig. S10 and Table S2). The charge extraction time (τex) was decreased from 2.8 to 0.6 μs (Fig. S9(b)), deduced from transient photocurrent (TPC). The equivalent carrier mobility was increased from 7.9 × 10–5 to 2.8 × 10–4 cm2/(V·s) (Fig. S11 and Table S3), deduced from photoinduced charge carrier extraction by linearly increasing voltage (Photo-CELIV). The blade-coated active layers with vacuum drying treatment led to better charge transport and extraction. By using the same processing, PM6:Y6:PC71BM solar cells (Fig. S12) delivered a 17.01% PCE (Fig. S13(a) and Table S1), which was certified to be 16.45% in South China National Center of Metrology (Fig. S14). This is a new PCE record for the green-solvent-processed blade-coated solar cells. The device with 1 cm2 area gave a PCE of 11.30% (Fig. S13(b)).
In conclusion, the effect of vacuum-drying method on the performance of blade-coated OSCs was thoroughly investigated. For PHT4:IT-4Cl device, a 80% increase in PCE was achieved. The vacuum-drying method leads to smoother film surface, longer charge carrier lifetime, decreased charge extraction time, and increased carrier mobility. By using this method, PM6:Y6:PC71BM solar cells gave a 17.01% PCE (certified 16.45%). This approach may help further developing efficient solar modules from green solvents.
Acknowledgements
The authors thank National Natural Science Foundation of China (61774077, 61804065, 51673070, 51521002, 21704082, 21875182, 21534003, 61705090 and 51320105014), National Key Research and Development Program of China (2016YFA0200700), China Postdoctoral Science Foundation (2017M623162), 111 Project 2.0 (BP2018008), the Basic and Applied Basic Research Fund of Guangdong Province (2019B1515120073), the Research and Development Program in Key Areas of Guangdong Province (2019B090921002 and 2019B010132004), the Fundamental Research Funds for the Central Universities (21618308), the State Key Laboratory of Luminescent Materials and Devices (2019-skllmd-05) and the Guangzhou Key Laboratory of Vacuum Coating Technologies and New Energy Materials Open Fund (KFVE20200006) for financial support. L. Ding thanks the National Key Research and Development Program of China (2017YFA0206600) and the National Natural Science Foundation of China (51773045, 21772030, 51922032, and 21961160720) for financial support.
Appendix A. Supplementary materials
Supplementary materials to this article can be found online at