The efficient utilization of solar energy and the decomposition of water into oxygen and hydrogen are the current hot spots in the community of research, with great application promise. Hydrogen is an important clean fuel and widely used in the fuel cell. Oxygen is a necessary gas for human survival. It is of significance for manned space exploration to achieve efficient oxygen acquisition and gas recycling in confined space.
As early as the 1970s, Fujishima et al. proposed a scheme of using TiO2 to decompose water by photoelectrochemistry[1]. However, TiO2 has such a large bandgap that it can only absorb UV light, which merely accounts for a small part of solar radiation. It’s difficult to meet the comprehensive requirements of high efficiency, low cost and high stability for TiO2. For this reason, researchers have adopted various approaches to improve the separation efficiency of charge carriers, focusing on morphology, electronic structure and interface characteristics of materials[2, 3].
Although solar water splitting uses abundant resources such as solar energy and water, it is difficult to reach the level of industrial application for the current energy conversion efficiency. The bottleneck of its development is the insufficient light absorption in visible light and near infrared region and the low efficiency of photocatalytic reaction. Modification of the surface and interface of photocatalyst can overcome these limitations effectively[4]. In recent years, in order to improve the efficiency of surface/interface carrier generation and separation, researchers have focused on the design of semiconductor multilayer heterojunctions and nanostructures to improve the efficiency of hydrogen production from solar water splitting. Nanostructures are designed to reduce the diffusion length of minority carriers. The size and morphology of nanostructures are important factors affecting the photocatalytic activity of catalysts, where nanostructures with high surface area can provide more photocatalytic sites[1, 5]. There are still many problems in improving the photocatalytic performance of nanomaterials[6].
If H2 and O2 with a stoichiometric ratio of 2 : 1 can be efficiently produced by overall water splitting without the dependence on sacrificial agent, hydrogen could actually replace conventional fossil fuels, and this is the ultimate goal researchers are trying to achieve. Onishi's group used SrTiO3–NiO to decompose water vapor into hydrogen and oxygen under the ultraviolet light produced by high-pressure mercury lamps, which achieved overall water splitting for the first time and started the research upsurge[7]. Zou et al. used Ni doped InTaO4 to achieve hydrogen production from overall water splitting in visible light, where the quantum efficiency is of 0.66%[8]. Domen’s group prepared GaN:ZnO solid solution with visible light response to achieve stable overall water splitting under visible light, which opened the avenue of non-oxide photocatalyst for overall water splitting[9]. Kang’s group prepared the metal-free nanocomposite of carbon dot and carbon nitride for photocatalytic overall water splitting under visible light, where the quantum efficiency at 420 nm was as high as 16% and the solar energy conversion efficiency in the full-spectrum was up to 2%[10].
Although some researchers have achieved overall water splitting with visible light response, the efficiency and stability still need to be improved. The EQE needs to be close to 100% and solar conversion efficiency needs to be over 10% for the industrialized application of solar water splitting. As the drawbacks of energy potential barrier of chemical reaction and the according reverse reaction, the efficiency and stability of water splitting can not be as high as solar cells. To this end, Domen et al. used traditional SrTiO3 as the model to achieve photocatalytic water splitting with a quantum efficiency of almost unity[11], which can make a close step towards industrialized application of it.
As the overall water splitting is an uphill reaction (Gibbs energy of +237 kJ/mol) consisting of multiple charge carriers transfer processes, to achieve a 100% IQE (internal quantum efficiency), the HER (hydrogen evolution reaction) and OER (oxygen evolution reaction) must proceed without any reverse reaction. Herein, Domen et al. constructed highly active HER and OER cocatalysts on Al doped SrTiO3 site-selectively to increase the EQE to the upper limit by a stepwise photodeposition method. As shown in Fig. 1, the results showed that the EQE values of the optimized sample at 350, 360, and 365 nm were determined to be 95.7%, 95.9% and 91.6%, respectively, which are the highest values reported so far for photocatalytic water splitting. The IQE should be close to 100% in the wavelength region of 350–360 nm on account of that the EQE exceeded 95%. In this case, a Rh core/Cr2O3 shell structure was formed on the surface of Al doped SrTiO3 through a two-step photodeposition, where the Rh promotes both the HER and the oxygen reduction reaction (ORR, a major backward electron transfer process), whereas the Cr2O3 shell inhibits only the ORR by blocking the access of evolved O2 to the surface of the Rh core[12]. Another cocatalyst of CoOOH promotes the OER, thus, the efficiency of the optimized photocatalyst of Rh/Cr2O3/CoOOH/SiTiO3:Al is boosted.

class="figure_img" id="Figure1"/>
Download
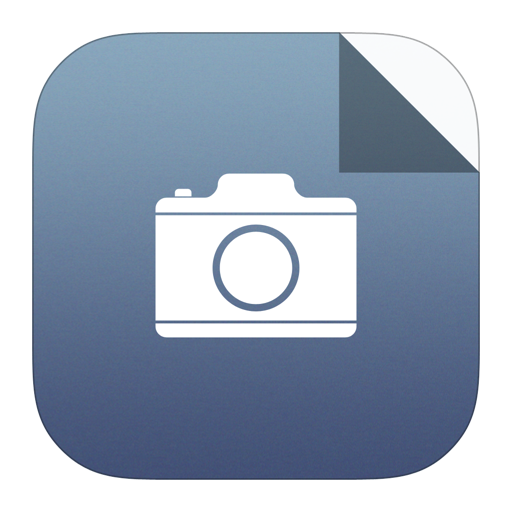
Larger image
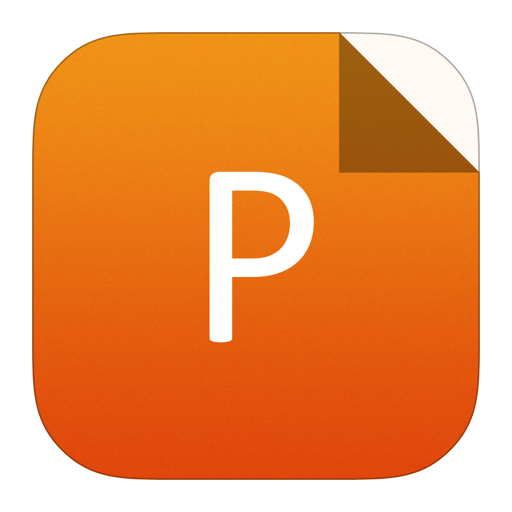
PowerPoint slide
Figure1.
Photocatalytic water-splitting activities. (a) Time course of H2 and O2 evolution on SrTiO3:Al loaded with various cocatalysts during photoirradiation. Left, loaded with Rh (0.1 wt%)/Cr2O3 (0.05 wt%) by two-step photodeposition. Middle, loaded with Rh (0.1 wt%)/Cr2O3 (0.05 wt%)/CoOOH (0.05 wt%) by three-step photodeposition. Right, loaded with Rh (0.1 wt%)-Cr (0.1 wt%) oxide by co-impregnation. (b) Ultraviolet-visible diffuse reflectance spectrum of bare SrTiO3:Al (black solid line) and wavelength dependence of external quantum efficiency (EQE) during water splitting on Rh (0.1 wt%)/Cr2O3 (0.05 wt%)/CoOOH (0.05 wt%)-loaded SrTiO3:Al (red symbols).
The Rh/Cr2O3 cocatalyst was found to be preferentially deposited on the (100) crystal facets, while the CoOOH cocatalyst was primarily located in the (110) direction, as illustrated in Fig. 2. The observed anisotropic deposition of cocatalysts can be attributed to a charge rectification effect inside each photocatalyst particle induced by an internal electric field, which originates from the work function difference between the respective facets. Increasing the work function difference obviously increases the extent of anisotropic charge separation, as well as the concentration of electrons at the {100} facets and of holes at the {110} facets, as seen from Fig. 3. Surface dipoles resulting from unbalanced cation/anion ratios on distinct facets may induce this work function difference even without forming junctions or composites.

class="figure_img" id="Figure2"/>
Download
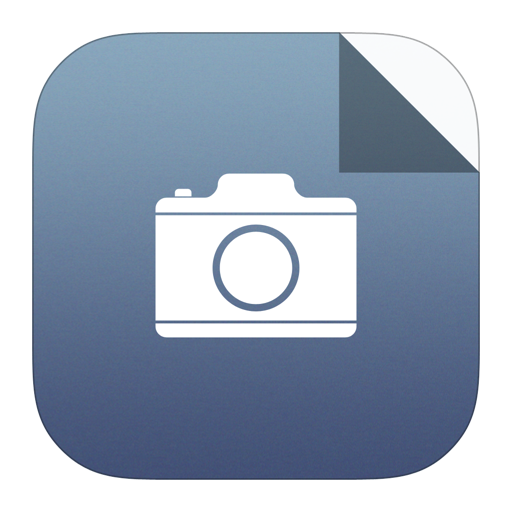
Larger image
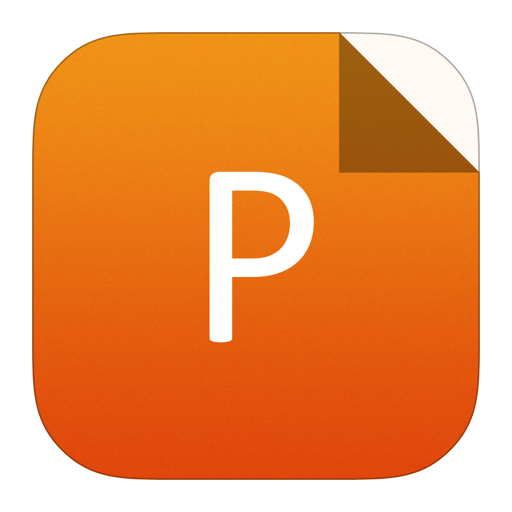
PowerPoint slide
Figure2.
Transmission electron microscopy. (a) Selected-area electron diffraction pattern obtained from SrTiO3:Al loaded with Rh (0.1 wt%)/Cr2O3 (0.05 wt%)/CoOOH (0.05 wt%). (b) Corresponding transmission electron microscopy image of a particle. (c) Particle morphology and crystal orientation.

class="figure_img" id="Figure3"/>
Download
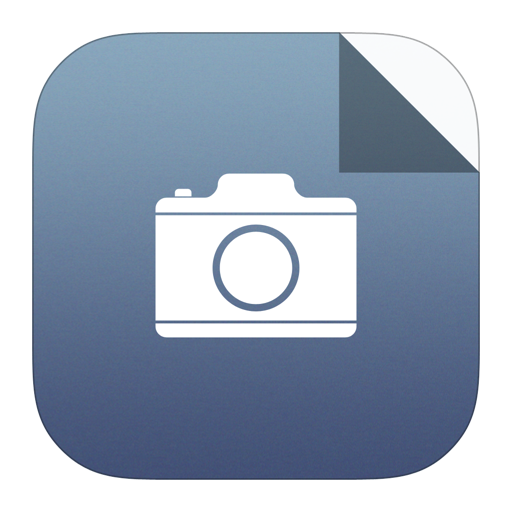
Larger image
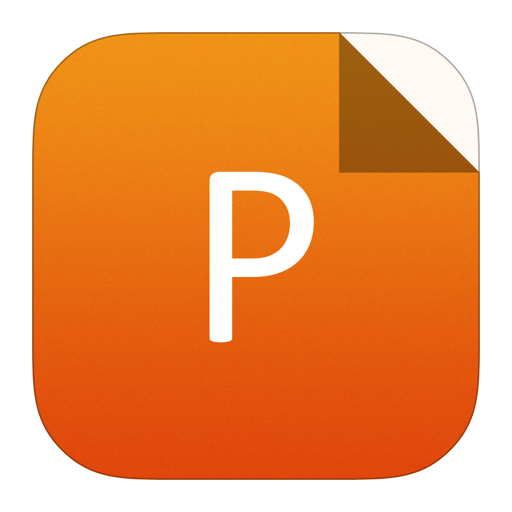
PowerPoint slide
Figure3.
Simulations of photocarrier distributions in SrTiO3:Al particles. (a) Mapping of conduction-band energy, Ec. (b) Density of electrons (e-), n. (c) Density of holes (h+), p. (d) Energy band diagram. (e) Electron and hole densities as functions of position (x′, y′) with work function difference ΔWel = 0.2 eV. (f) Effect of ΔWel on electron-to-hole-density ratio at the {100} and {110} facets.
This study demonstrates overall water splitting with an IQE close to unity by applying aluminium doping for defect suppression, flux treatment to improve the crystallinity, and a Cr2O3 shell to inhibit ORR, in addition to facet engineering, and thus gives a definitive answer to this problem. The selection of high-performance cocatalysts for the HER and OER is another important aspect. Recently, Ta3N5 and Y2Ti2O5S2 have been reported to split water into hydrogen and oxygen under visible light[13, 14]. These materials absorb visible light with wavelengths of up to 600 and 640 nm, respectively, and the STH (solar-to-hydrogen) efficiency can reach 10% once the EQE is improved to a level similar to that of SrTiO3:Al.