1.
Introduction
This report gives three more protonic (in contrast to electronic) semiconductor physics-based models to account for the abnormally high electrical conductivity of pure liquid water (0–100 °C), reported but unexplained for more than 200 years since Volta invented the electrolytic cell or battery at the turn of the century, two centuries ago in 1900. The results of these three more models are summarized in a table containing also the outcomes of our first-round tests[1] of our Melted-Ice-Lattice Model of pure liquid water as a unipolar trappy proton semiconductor, or as a bipolar trappy or trap-free proton-prohol (protonic hole) semiconductor, so that all of our models can be viewed and compared At One Glance (our favorite A1G first used by us in print in our 1991 college textbook[2] and earlier teaching notes).
To test the numerical accuracy and to evaluate the soundness of the physics-bases of our models, our input data are calculated from the empirical-equations used by theengineers to fit the raw experimental data, which were obtained by the pioneer Chemists and Physicists, in pure liquid water[1] (namely, pure water material in its liquid state from 0 to 100 °C at one atmospheric pressure), during the past 80 years, recognized as the world-consensus, and known as the ion product, which is the algebraic product of the concentration of the two assumed mobile-ion species, a positive ion, known as hydronium ion, with the symbol (H3O)+, and a negative ion, known as hydroxide ion, with the symbol (OH)?1 or (HO)?1 and the popular chemical name, pH.
Our second and third input data are the 50-year-young world-consensus empirically calculated experimental data (See above for detailed descriptions.) of the ion mobilities, (which are electrical mobility of the two, just described, ion species), also in the pure liquid water material.
In our tests of goodness of our bipolar and unipolar Protonic Semiconductor Models (PSModels or PSMs, contrasting the well-established Electronic Semiconductor Models, ESModels or ESMs), we ignored the fact that the empirical equations and empirical theories were used to nonlinear least-squared-fit (NLSF) the raw experimental data ('raw' for the data obtained experimentally before any mathematical processing of the data numbers, by the pioneer experimentalists world-wide, which all still contain significant variations and hence uncertainties, about 10%, according to the chemical engineers who did the empirical fits for water vapor and liquid) to give the empirical equations calculated experimental data, which we have used as our input data, to quantitatively test the accuracy and the physics soundness of our bipolar and unipolar PSModels.
All the protonic models we have proposed and tested, including the three newest ones, given in this report, have given %RMS deviations in the liquid water range (0–100 °C), less than or within the confidence limit of about 10% of the 80 years-old (Ion Product or pH) and the 50 years-old (ion mobilities) raw and empirically calculated experimental data. Although this large uncertainty has not hindered the many materials engineering applications, as testified by all the marvelous niceties enjoyed by human today, which have been attained in much less than a century, the uncertainty still led us to the next-round tests, in order to seek the less-empirical physics-based models which are designed to be able to extrapolate into the spacetime regimes outside of the box of validity from the currently used empirical models which can accurately interpolate inside the box, but fail to extrapolate to the regimes outside the box, of new applications, such as the properties of the interfaces between two materials (solid/liquid, liquid/vapor and vapor/solid) including bare surfaces (solid/vacuum), and also including materials in small volumes and clusters, encountered in nanotechnology.
To this end, of seeking the less-empirical and more physics-based spacetime extrapolatable models for our next-round tests, the raw experimental data will be re-evaluated via NLSF to our physics-based model equations, instead of the empirical equations, to evaluate the goodness of the fit parameters’ values against the anticipated values based on our water physics and model of pure liquid water and also vapor water. This will be reported in our next journal article, Studies of Water VII. The present and sixth article, Studies of Water VI. (W6), will extend our model tests described in our previous report, Studies of Water V.[1] (W5) to three groups of models envisioned by classical particle and wave mechanics and its wave and quantum mechanical representations.
2.
Our Three Additional Protonic Models
The only difference between our current models and our previous models is the selection of the NLSF parameters to fit the same input data. In our previous models in W5[1], all the parameters in our physics-based model equations were variable NLSF parameters to fit our physics-based model equations to the input data by iteration to a set of defined convergence criteria.
For the present three additional physics-based models, the value of the three thermal activation energies (at or near thermodynamic equilibrium) are preassigned at the vaporization point, 100 °C, because of the excellent agreement (to within a percent or two) of the three NSLF thermal activation energies, with the three experimental vibrational frequencies of the two hydrogen ions (actually two protons and will from now on designated so) measured by IR in vapor water. The two protons are pair-electron (our gluon) bonded to the oxygen core of isolated water molecule in the vapor phase and its asymptotic highly diluted gas phase (in vacuum and in the dark), whose three vibrational frequencies have been measured to great precision in this century, for example, see Eisenberg and Kauzmann cited in our W5[1].
The binding or bond energy, of the two protons around one oxygen core in the liquid water, were calculated by us using the simple one-dimensional (1-D) Ball-and-Spring (point-mass proton and nearly point-mass oxygen core, while the springs are the pair-electron bonds, our gluons, coined by Crawford in 1963 as the Slinky Toy model, cited in our W5[1], which was simply extended by us in W5[1] to 2-D to include the additional force on the vibrating proton from the lone pair (Since it is no longer lone or lonely due to the presence of the second oxygen core which is at twice the distance away from the proton, we coined it the LONG pair from the longer electron pair bond length between the proton and the second oxygen core, which is about 2 Angstrom, and twice the distance between the proton and the first oxygen core, about 1 Angstrom which is almost the same as proton-oxygen-core distance in the vapor or gas phase of water or water material.). To our most pleasant surprise, although anticipated by our back-the-envelope, actually mental, estimate, this additional force or ‘long’ (longer) spring increases the vibrational frequency or kinetic energy of the vibrating proton in liquid water, just the correct amount, over that of the proton in water vapor (from well-known for a century, experimental IR data taken on vapor water, listed by Eisenberg and Kauzmann[1]) using only the first term of the power series bond length or the length of the spring, and this additional force gives almost exactly the increased thermal activation energy or ionization energy of the expansion of the interionic force, which gives the force constant of the spring, inversely proportional to the pair electron ion-product or pH in pure liquid water to about 540 meV or 4400 cm?1 from that of the isolated water molecule in water vapor observed by IR.
A similar excellent extrapolation of the proton vibration frequencies in vapor to the two thermal activation energies, one each from the two ion mobilities, that resolved the difference of only 20 meV or one proton mass, between 7 and 8, or 8 and 9, even 7 and 9, protons, localized and not-localized, in the hexagonal close packed (HCP) primitive unit cell (PUC), containing four water molecules, used in our melted ice model of liquid water, not the cubic PUC of certain amorphous thin layer of surface ice at very low temperatures, containing only two water molecules, decisively supporting the melted ice model of the vast majority HCP ice.
So, our melted ice model for the liquid water, has two unique features, anticipated and required by basic physics, which provided the existence proofs of our physics based water model, namely, it is a combination of the 1933 Bernal-Fowler ICE Lattice Model which satisfies the Born-Von Karman periodic or cyclic boundary condition, and the older Random Gas Model of sparse number of water molecules and the denser Vapor Water Model that follows the Maxwellian Kinetic energy distribution law whose characterization at and near thermodynamic equilibrium could be quantitively accounted for by an effective medium theory (EMT). In contrast, the closest past models have employed solely the EMT, missing the long-range force to input and output or transport the kinetic energy between the energy source and sink, conveyed by drift and diffusion via the correlated vibrations or vibrational waves, propagating on long strings of vibrational protons on the crystalline lattice. The presence of the crystalline lattice from the melted ice, requires a mental and physical picture of the fluidity properties of the liquid water, whose long range fluidity confined by a harder than water container tube is sufficiently longer-larger (in 3-D) than the correlation or influence length of atomic-molecular primitive unit cells located within a correlation length distance.
3.
Band and Bond Diagrams of Our Models and Underlying Physics
The bond diagrams are shown in Figs. 1 and 2 and described in detail in their captions. They are the pair-electron bonds which glue the charged oxygen cores together to form the hexagonal close packed lattice observed in ice from which our melted ice lattice model for liquid water is derived. They are the proton transport diagrams showing the proton and prohol generation, recombination, drift (and diffusion) transport pathways. Fig. 1 shows the generation-recombination pathways of protons and prohols. Fig. 2 shows the proton trapping-detrapping limited drift and diffusion pathways.

class="figure_img" id="Figure1"/>
Download
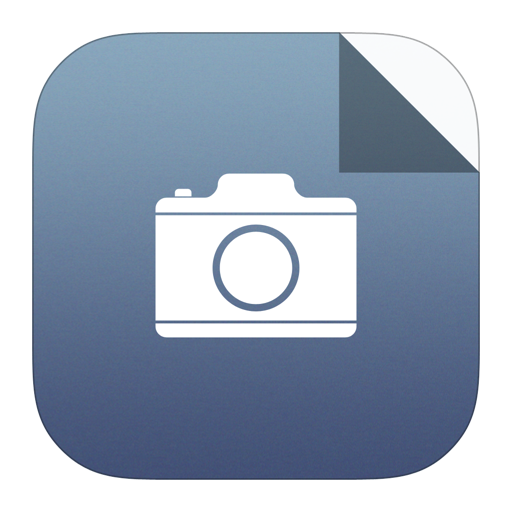
Larger image
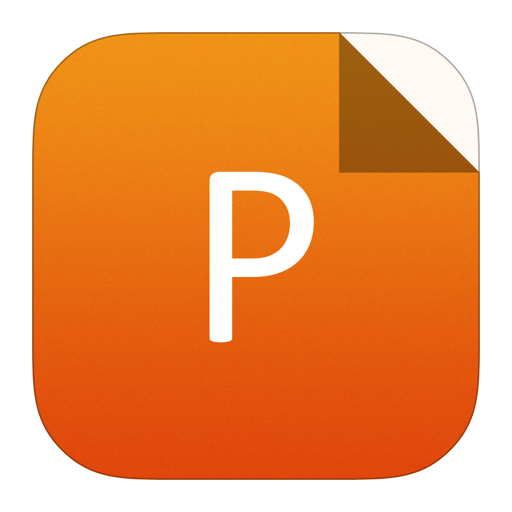
PowerPoint slide
Figure1.
(Color online) Position space diagram of our 3-step (A, B, C) Collision Dynamics (DD Transport and GRT Kinetics: DDGRT ? Drift, Diffusion, Generation, Recombination and Trapping) of point-mass protons in our Born-Von Karman Periodic Lattice Model of the melted ice for pure liquid water from the 1933 Bernal-Fowler Ice Model of Hexagonal Close Packed (hcp) Lattice. The Primitive Unit Cell (PUC), U, contains 4 water molecules in the hcp configuration with four tetrahedrally directed nearest neighbor Oxygen nuclei at 3 Angstroms (300 picometers) distance apart, and two trisector proton trapping sites at 100 pm from the nearest Oxygen nucleus. Only one of two proton trapping sites is occupied by or filled with a proton when water is frozen (T = 0°K) or KE(Kinetic Energy) = 0 meV. The 2-D plane view is the projection along the c-axis of the HCP Ice Lattice. The numerical values are the thermal activation energies and scattering energy changes of the protons and their corresponding absorbed or emitted phonon energies of the vibrating protons or oxygen nuclei.
The one-band (proton conduction band) and three proton trap model employed in the next section to analyze the experimental data can be readily derived from the bond diagrams given in Figs. 1 and 2. In this case, the water dimer, shown in Fig. 1, could be viewed as the bond site to generate the mobile proton and immobile or trapped prohol pair by the absorption of a single 540 meV protonic phonon, ω1 = 540 meV from one of the 8 protons in the hexagonal close packed (HCP) primitive unit cell (PUC) of the melted ice lattice. However, the concentration of the positive point-mass proton, p+, from the pH value, gives an inter-proton distance of about 2400 Angstroms or about 400 times the dimension of the hexagon of the HCP PUC, therefore it would not be the nearest neighbor dimer or two water molecules, rather those labeled A and C in Fig. 1 with the inter-PUC distance of 2400 Angstroms labeled.
Fig. 2 shows the other two trapping limited transport pathways to account for the two thermally activated electrical mobilities of the input data, both from positive point-mass protons, differing only in the charge state of the protons' initial and final trap, giving the direction of the flow of the electrical charge, while the direction of the flow of the mass of the proton is in one direction only, namely the negative electric field direction for the positive point-mass proton. Our 3-step (ABC) protonic transport model has the unique feature of being able to distinguish the initial A (or C) and final C (or A) trap by one proton vibrational energy, 7 and 8, 8 and 9, or even 7 and 9, for the one band three trap unipolar model, the two band one amphoteric trap bipolar model, and also the three band trap-free bipolar model. The mathematical solution, such as that from the Variation Principle, of this many-body or many-particle-species system, favors the three-band model, which is also evident from the two bond pictures given in Figs. 1 and 2, namely, the empty band and the filled band (by the positive point-mass protons) separated by the wider energy gap, 540 meV, are obvious from these two bond pictures. The third and narrow band inside the wider energy gap, from the 8 protons occupied 16 proton sites, and the 8 empty or unoccupied 16 proton sites, repeated from the PUC to the next, over the entire Melted Ice Lattice, would form two bands which would merge in the middle of the wide gap, to give one narrow band. The symmetry is amazing, namely it is located nearly the middle of the 540 meV wide gap with an energy bandwidth nearly one third of theenergy gapwidth, 540/3 = 180 meV and the two inside gaps of 170 meV for the protons and 190 meV for the prohols, exactly that expected by nine protons from the PUC that has trapped one proton to become one proton heavier, hence a lower vibration frequency or energy, of ω9 = 180 meV, while the other, ω8 = 191 meV would be the trapping limited proton and proholess model, while ω7 = 204 meV would be the theory preferred bipolar proton-prohol trap-free model demanded by our melted ice lattice model for the pure liquid water. The configurationentropy agrees, reducing Protonic Effective DOS by at least 16!/8!8! to 2 × 1019 cm–3.
4.
Numerical Results of Our Models
The previous models we explored in our 5th manuscript[1] are given in Fig. 3. The three activation energies were extracted from the three different experimental data in the liquid water temperature range 0–100 °C at one atmospheric pressure. These three energies have the same temperature dependence as that of water density, [dw(T)/dw(TM)]?ndw. Models (a) and (c) assume that the two protonic energy bands of pure liquid water are similar to the two electronic energy bands of elemental semiconductors, so the activation energy from the ion-product equals the energy gap between the two bands, and the pre-exponent factor in the concentration formula equals the theoretical factor T3/2 or nTe = +3/2. From our 4th manuscript, we obtained the best value of ndw = 1.0. Models (b) and (d) assume no proton-prohol pairs but only positive protons in pure liquid water, so the activation energy from the ion-product equals the capture/emission energy of a protonic trap. The activation energies from the two mobility data were extracted based on our 3-STEP Collision Dynamic Kinetics on proton transport in the pure liquid water with the HCP melted ice lattice which primitive unit cell contains four water molecules. The kinetics is shown in Fig. 1 and Fig. 2. Fig. 1 illustrates the generation-recombination pathways in bond diagram for Models (a) and (c), and Fig. 2 the trapping and detrapping pathways in bond diagram for all four models. The mobile protons of Step B are in the protonic conduction energy band, while the initial state (initial primitive unit cell) of Step A and the final state (final primitive unit cell) of Step C are either a protonic bound-trap or a protonic band-state. The activation energy of the 3-STEP collision dynamic kinetics is determined by the discrete energy or frequency of the local protonic phonons absorbed in the Step A emission of protons, ω1, ω9, ω8, or ω7. The phonons which scatter the mobile protons in Step B are wateric phonons with continuous frequency spectrum like Debye acoustic phonon branches, 0 to ω72, and their contribution to the protonic mobility are well described by the theoretical phonon-scattering mobility expression for the elemental semiconductors. In these four models, the ratio of mobile positive charge to mobile negative charge is assumed to be one, so that the mobile positive charge density p+ equals to the square root of the ion-product, however, the charge neutrality may or may not be met.

class="figure_img" id="Figure2"/>
Download
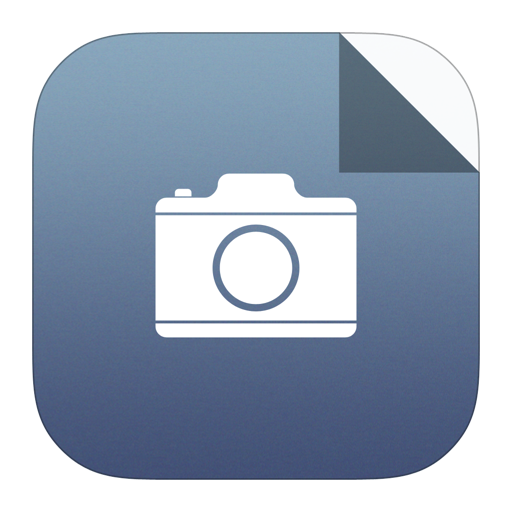
Larger image
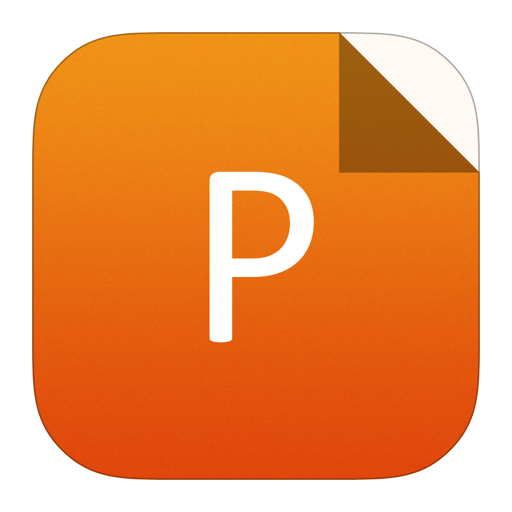
PowerPoint slide
Figure2.
(Color online) Same as Fig. 1 except showing the route details of the phonon-assisted 3-step (A, B, C) trapping-limited diffusion-drift transport processes.
Three new protonic models are proposed in this report, with a new classification. We recognize that protons in the primitive unit cells of the melted ice lattice have two types of movement, the vibrational movement around their lattice positions and the translational movement among the lattice positions and the unoccupied proton sites. The vibrations of protons in the extended water material are described by the protonic vibrational waves quantized by protonic phonons. The jumps of protons in the extended water material are described by one-proton Bloch functions characterized by a quasi-particle (fermionic proton, or positive proton, or proton). The solution of the Schrodinger Equation of the one-proton Bloch functions gives the protonic energy bands. It could be one-band, two-band and three-band solution. Fig. 4 lists one-band, two-band and three-band models. One-band model gives the only mobile charge carrier, positive proton p+, which needs two bound-trap species to address the three experimental activation energies: neutral trap with one activation energy to capture mobile positive proton, negative trap with two activation energies to capture mobile positive protons. Two-band model gives two mobile charge carriers, proton p+ and prohol p?, which has an activation energy to generate the proton-prohol pair, and which needs one bound-trap species to address the other two activation energies: amphoteric trap with three charge states. Three-band model gives four mobile charge carriers, two protons (p8+, p9+) and two prohols (p8? and p7?), which has three activation energies to generate the p8+?p8? pair, the p8+?p7? pair, and the p9+?p8? pair, respectively, and which does not need any bound-trap species to provide the three experimental activation energies.

class="figure_img" id="Figure4"/>
Download
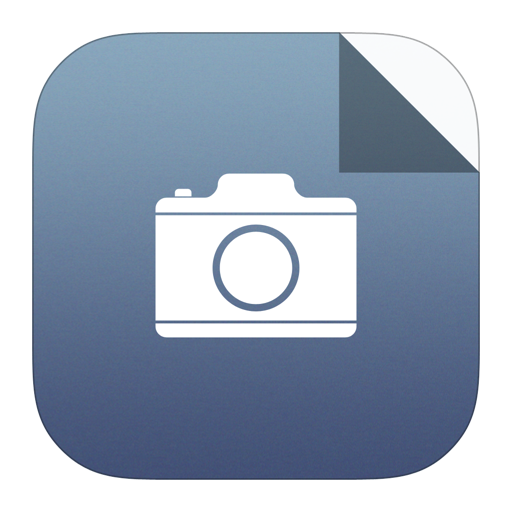
Larger image
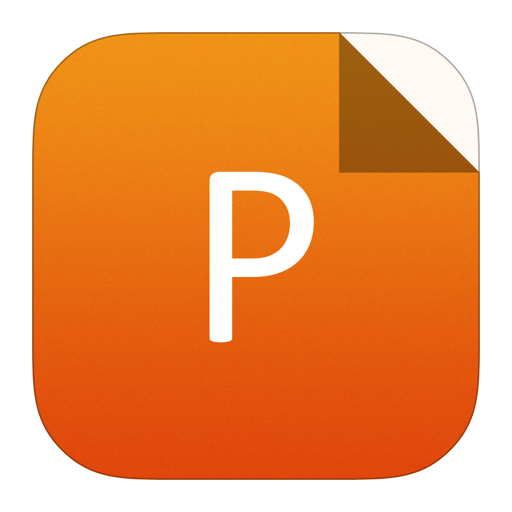
PowerPoint slide
Figure4.
The comparison table of three new protonic models for pure liquid water. (a) one-band unipolar model with two trap species and three trap energy levels, (b) two-band bipolar model with one trap species and three charge states, and (c) three-band bipolar model with no trap species. Different from the models in Fig. 3, these three models are with the charge neutrality from the mobile charges and trap charges, which leads to the inequality of mobile positive charge and mobile negative charge. Also, the phonon energies at the boiling temperature 100 °C are derived from the symmetrical stretching mode frequency of isolated water molecules based on the 1-D inverse bond length force constant assumption.
The tables in Fig. 3 and Fig. 4 have the same row labels. The first row of each table shows the numerical results of the physics-based fit of the ion-product data, the column nTe either the theoretical value of +3/2 or the fitting parameter numerical result, the column ndw either the best experimental guess value of +1.0 or the fitting parameter numerical result of the exponent of the water density factor in the protonic phonon energies, the column ω1@100°C either the best theoretical value of 555.26 meV or the fitting parameter numerical result of the one-proton phonon energy, the column p0 either the best theoretical value of 2 × 1019 cm?3 or the fitting parameter numerical result of the concentration coefficient (see equation on page 8 of W5[1]). The second, fourth and sixth rows show the numerical results of the physics-based fit of the positive ion mobility data, and the third, fifth and seventh rows show those of the negative ion mobility data. The column nTe in the second and third rows gives the fitting parameter numerical result of the exponent of the temperature factor in the Step A mobility expression (see equations on page 9 of W5[1]). The column ω9@100°C in the second row gives the 9-proton phonon energy absorbed during the Step A emission of proton from the protonic trap to the protonic conduction band, either the best theoretical value of 185.09 meV or the fitting parameter numerical result. The column ω8,7@100°C in the third row gives the 8-(7-)proton phonon energy absorbed during the Step A emission of proton from the protonic trap to the protonic conduction band, either the best theoretical value of 196.31 meV (209.87 meV) or the fitting parameter numerical result.

class="figure_img" id="Figure3"/>
Download
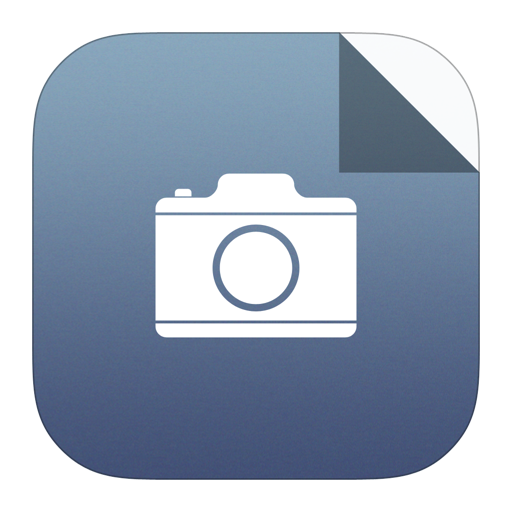
Larger image
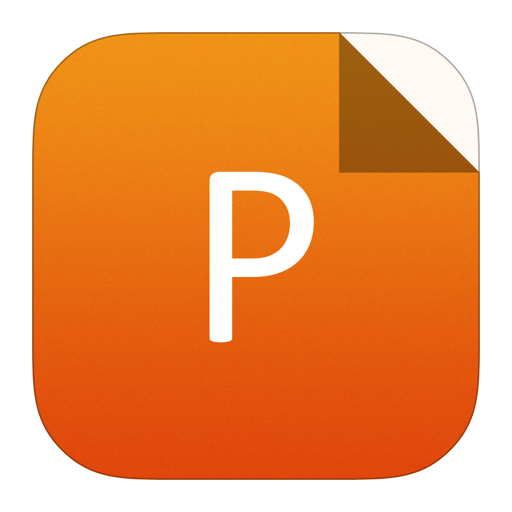
PowerPoint slide
Figure3.
Reproduce Fig. 5 of W5[1] for easy comparison with the following modification. The column labels for the activation energies E± and Eμ+, μ? were replaced by the corresponding protonic phonon energies. The energy coefficients were changed to the protonic phonon energies at the boiling temperature 100 °C. In addition, one column was replaced by the p+/p? column to indicate that the charge neutrality was not applied in W5[1] and the mobile charge ratio is set to be 1.
The %RMS deviations in the last column of the first row (ion product), the sixth row (positive ion mobility) and the seventh row (negative ion mobility) are the figure of merit of each model. The general conclusions are as follows, which lead to anticipated further studies.
(1) All the models are good enough considering the 10% uncertainty of the input data, which was noted by the chemists who obtained the international consensus table and data of the three characteristic parameters, the ion product or pH and the two ion mobilities, in the entire pure liquid water temperature range. But this 10% accuracy is insufficient to distinguish the physics goodness of our models and their extrapolation capability to outside the box such as the nanotechnology regime involving surfaces and interfaces.
(2) The input data are not self-consistent with our models which are using the input data to determine the goodness of our models and to uncover hidden properties. Thus, the raw data need to be analyzed using each of our models. The best models determined by resulting input data can then be used to give the most accurate model which could be extended to give the conditions of phase transition and also the characteristic equations of the multi-parameter phase boundaries.
Acknowledgments
This study has been supported by Xiamen University, Fujian, China. We acknowledge the initial request made to the senior author, Chihtang Sah, by Dean and Professor of Physics, Dr. Chenxu Wu to give a presentation on the State of Microelectronics at the Pentung Sah 111th Anniversary Memorial Symposium during the 2013 National Fall Meeting of the Chinese Physical Society (CPS), hosted by the School of Physics and held for the first time at Xiamen University. Instead, we invited the expert, Dr. Yuan-Chen Sun, a former PhD student of Sah, who was more familiar in this subject as the Senior Vice President of Research and CTO (Chief Technology Officer) just retired from TSMC (Taiwan Semiconductor Manufacturing Company), to give the keynote on the State of Microelectronics. That left us still needing a presentation subject. A commentary in a weekly issue of PNAS (Proceedings of the US National Academy of Sciences) in July, 2013, had a glaring headline on the abnormally high electrical conductivity of pure water[1]. We thought this was well-known and understood in 1959 when a Monograph (No. 139) titled Semiconductor, edited by Hannay, was published by the ACS (American Chemical Society). This led us to decide to discuss the model of water to complement Sun’s Keynote in order to fulfill a two or three presentation program needed for the Pentung Sah 111th Anniversary Memorial Symposium, scheduled for six weeks later, on Friday, September the 13th, of the 2013 Fall Meeting of the CPS, not anticipating the million articles and more than a hundred textbooks on water published in the previous 210 years which needed to be reviewed and studied in order to give an informed presentation to the 2000 physics graduate students and faculty members attending this annual fall meeting of the CPS. We also thank Dean Chenxu Wu for asking us to present our water physics and model findings in a two-hour tutorial lecture at the 10th National Theoretical Physics Summer School of the Natural Science Foundation of China, hosted by the Physics Department of Xiamen University on Friday, August 6, 2016. We also acknowledge the Chinese Academy of Sciences (CAS) in Beijing for the invitation to present our water study findings in two seminars (a 30 minute executive report to the academicians and a 2 hour seminar to active researching staff members) on June 2 and 3, 2016, at its biannual Assembly of Members in Beijing, China. All of the figures in this paper came from those three presentations and some of which were modified from the figures given in our four earlier journal articles. We also thank previous President and Professor, Dr. Chongshi Zhu for hiring us in April 2010 as faculty members of Xiamen University and for supporting our teaching and research interests, and the continuation of this support from the new Xiamen University President Dr. Rong Zhang and Physics Dean Dr. Taotao Fang. This study is also supported by the estate of Linda Su-Nan Chang, the late wife of the third author, Chihtang Sah. Finally, not the least, we acknowledge a spirited and enthusiastic supportive discussion with ECE Professor Cardinal (Cardi) Warde of MIT (Massachusetts Institute of Technology) on both the pure and applied physics aspects of our Water Physics and Model, including a most important application, that of the internet communication via the Third Media, the water in the oceans, which is 80% of the surface of the planet Earth, especially between the islands in his native Caribbean’s.