1.
Introduction
The whispering gallery mode (WGM) resonator has the excellent ability of confining light via total internal reflection at the interface between its high refractive index and low refractive index surrounding. Due to its small size and a high quality factor of the resonator, it is a good candidate for achieving low threshold and narrow linewidth lasers[1–5]. Incorporating quantum dots (QDs) into a WGM microcavity for on-chip light source has been extensively studied in recent years[6, 7]. Note that Si-based photonic devices, such as photodetectors, wave-division multiplexers, light modulators and light switch, have been demonstrated as a result of the significant development of the Si-based manufacturing processing in the past decades. However, it is difficult to fabricate silicon-based laser by directly epitaxial growing III–V optically active material on the silicon due to the fact that they have a large lattice mismatch and different thermal expansion coefficients[8, 9]. Hence, the bonding technique becomes an effective alternative method to realize hybrid III–V/silicon chip light sources[10–17]. III–V/silicon hybrid integrated laser can combine both advantages of III–V emission properties and conventional silicon COMS processes, which will have significant applications in photonic integrated circuits of the silicon optical communication[18, 19].
In this letter, we report on InAs/GaAs QD lasers with GaAs WGM microdisk cavity integrated on the silica/silicon substrate through the bonding technique. At room temperature, lasing emissions are observed with a 5 μm diameter microdisk and the corresponding wavelength is approximately 1200 nm. Numerical calculations are employed to understand the lasing modes. Our reports show an approach to integrate the InAs/GaAs QD active layer on the SiO2/Si chip for low threshold QD WGM microdisk lasers.
2.
Sample fabrication
Two- and three-layer stacked InAs/GaAs QD samples are grown using the molecular-beam epitaxy (MBE) on a semi-insulating GaAs substrate. We first grow a 300 nm GaAs buffer layer and a 100 nm AlAs sacrificial layer. Then the active layers of QDs are grown. For the two-layer stacked QD sample, its structure consists of two periods of 90 nm GaAs and InAs QD layers, and a 90 nm GaAs cap layer. The three-layer stacked QD sample consists of three periods of 50 nm GaAs and InAs QDs layers, and a 140 nm GaAs cap layer. Here, a 100 nm AlAs layer can be etched by using a 19% concentration hydrofluoric acid solution for obtaining the QD active layers. Then the active layer is bonded onto a 1000 nm thickness silica wafer. The GaAs microdisk with InAs/GaAs QDs integrated on the SiO2/Si substrate can be achieved through photolithography and inductively coupled plasma (ICP) etching.
Fig. 1 exhibits the photoluminescence (PL) spectra of two- and three-layer stacked InAs/GaAs QD samples grown on GaAs substrates at the laser excitation power of 24 μW before bonding the QD active layer to the silica. They show a typical PL lineshape of ensemble QDs. Next, InAs/GaAs QD microdisks on SiO2/Si chip are fabricated. The details of the procedure are shown in Figs. 2(a)–2(e), i.e., in sequences, putting a layer of AZ6130 photoresist on the sample, ultraviolet (UV) exposure to define 5 μm diameter microdisk patterns, ICP etching InAs/GaAs layer using Cl2/BCl3 mixed gases and ICP etching silica layer using CHF3/Ar mixed gases. As an example, Fig. 2(f) presents a picture of a 5 μm diameter microdisk obtained by scanning electron micrograph (SEM). It can be seen that the top thin layer is the InAs/GaAs QDs layer.

class="figure_img" id="Figure2"/>
Download
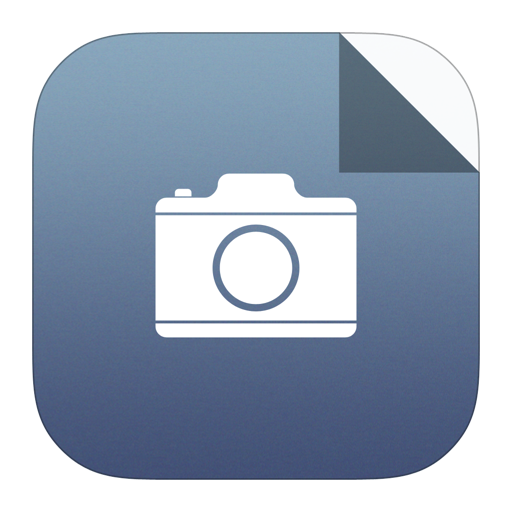
Larger image
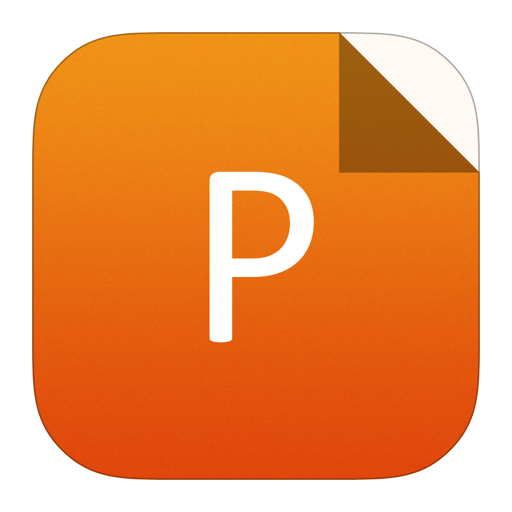
PowerPoint slide
Figure2.
(Color online) Detail processes of the microdisk fabrications. (a) QD sample structure of two-layer stack InAs/GaAs QDs. (b) Bonding the QD active layer on the silica chip and photolithography to define 5 μm diameter microdisk patterns. (c) Developing to acquire the microdisk patterns. (d) ICP etching InAs/GaAs QD active layer. (e) ICP etching silica layer. (f) SEM picture of the fabricated microdisk with a 5 μm diameter.

class="figure_img" id="Figure1"/>
Download
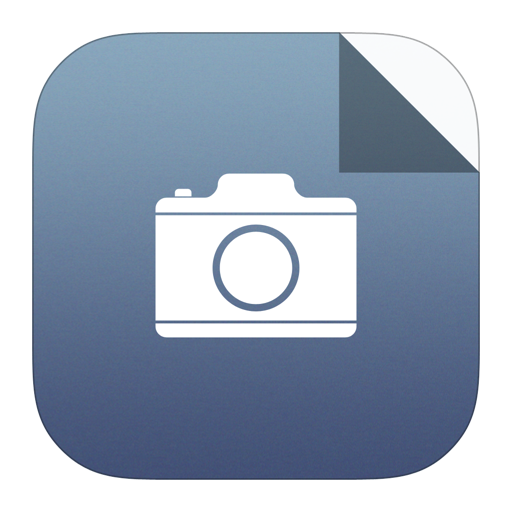
Larger image
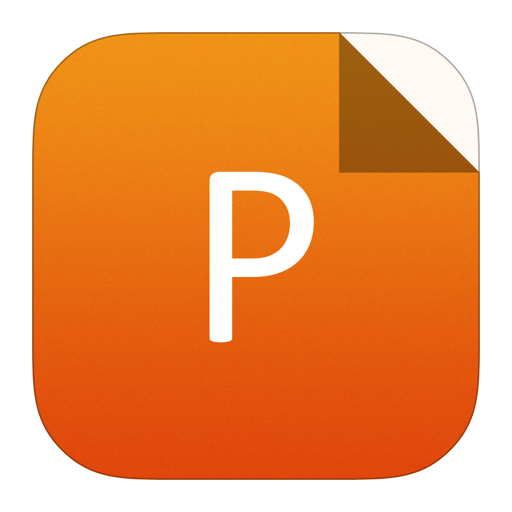
PowerPoint slide
Figure1.
(Color online) Room temperature PL spectra of two- and three-layer stacked InAs/GaAs QD samples grown on GaAs substrates at the laser excitation power of 24 μW.
3.
Results
The microdisk samples are optically pumped by a 640 nm continuous wave (CW) diode laser at room temperature. The excitation laser beam is focused onto QD samples from the top of the microdisk by a microscope objective (NA: 0.65), PL spectra are collected using the same objective, and measured using a 0.3 m focal length monochromator equipped with an InGaAs linear photodiode array detector. Fig. 3(a) exhibits the PL spectra of the two-layer stacked QD sample under different pump powers. It can be seen that, at low pump powers, a few narrow PL emission lines are observed which should correspond to different cavity modes. As the pump power increases, the corresponding emission line intensities become stronger. To clearly demonstrate the PL intensity increase as a function of pump power, light-out versus light-in (L–L) curves of the dominant PL peaks corresponding to the wavelengths of 1154.16 and 1181.72 nm, respectively, are shown in Fig. 3(b). Here, you can see that both emission peak intensities clearly exhibit a superliner increase as the pump power increases, showing typical lasing characteristics. In contrast, however, the background emission intensity, such as at a wavelength of 1163.28 nm, exhibits a liner increase with increasing pump power, as shown in Fig. 3(b). The obtained lasing thresholds are approximately 172.6 and 164.6 μW for the emission peaks of 1154.16 and 1181.72 nm, respectively. In the inset of Fig. 3(b), a double logarithmic scale L–L curve for an emission peak of 1181.72 nm is shown, clearly exhibiting a kind of “S-shaped” property. This result verifies a transition from the spontaneous to stimulated emissions[20–22]. The derived quality factor (Q = λ/Δλ) of the microdisk studied is 2813 according to its full width at half maximum (FWHM) of 0.42 nm at a wavelength of 1181.72 nm when the pump power (~140 μW) is below the lasing threshold power.

class="figure_img" id="Figure3"/>
Download
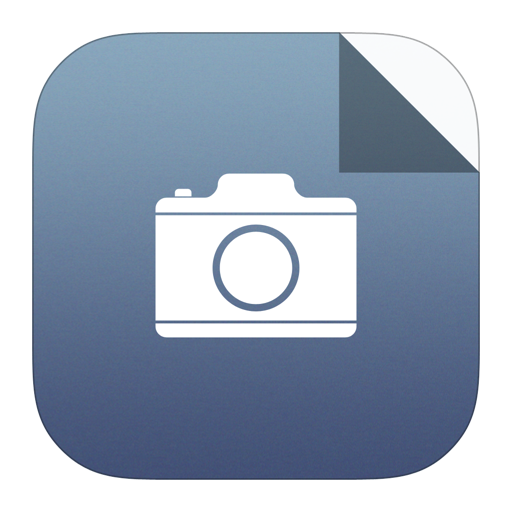
Larger image
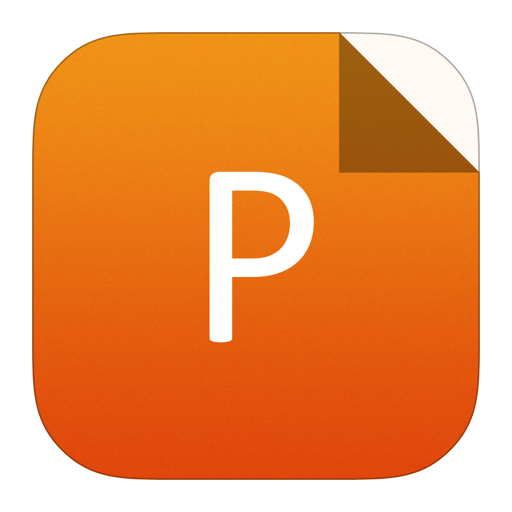
PowerPoint slide
Figure3.
(Color online) Two-layer stacked QD microdisk sample. (a) PL emission spectra under different pump powers. The two lasing mode peaks studied are 1154.16 and 1181.72 nm, respectively. The reference background emission wavelength of 1163.28 nm is chosen. (b) Light-out versus light-in curve of the lasing mode peaks and background emission. The lasing mode peak intensities exhibit a superliner increase, while the background signal exhibits a liner increase. Inset is the light-out versus light-in curve with double logarithmic scale for the lasing mode peak of 1181.72 nm.
Fig. 4(a) exhibits the PL spectra of a three-layer stacked QD sample under different pump powers. Two emission peaks with wavelengths of 1131.69 and 1162.01 nm, respectively, are chosen for analyzing their peak intensity increase as a function of the pump power, which are summarized in Fig. 4(b). The obtained lasing thresholds corresponding to the wavelengths of 1131.69 and 1162.01 nm, respectively, are 40.08 and 27.66 μW. In the inset of Fig. 4(b), for the emission line of 1131.69 nm, it clearly shows a decrease of the FWHM first and then remains almost unchangeable with increasing pump power. The corresponding critical pump power is approximately 37 μW, which is close to the laser threshold obtained above. Fig. 4(c) shows the double logarithmic scale L–L curves, distinct “S-shaped” behaviors are observed for emission lines of 1131.69 and 1162.01 nm, respectively, which further confirms the lasing emission in the QD microdisk sample. Here, the corresponding cavity modes of the wavelengths of 1131.69 and 1162.01 nm may be attributed to the TE29, 1 and TE28, 1 modes of the electromagnetic field distribution in the microdisk, respectively, by employing COMSOL Multiphysics program calculations, where the first and second subscripts are azimuthal and radial order numbers. Fig. 4(d) shows the calculated electric field distribution of the TE29, 1 mode. Its effective mode volume Veff is approximately 7.57 × 10?19 m3 and the effective index neff ≈ 2.48. Then the estimated optical thickness of half wavelength λ/2neff ≈ 228 nm, which is less than a thickness of the GaAs microdisk of 290 nm, implying that it not only allowed fundamental TE modes but also allowed TM modes and higher-order TE modes[23]. Note that only when the microdisk thickness is less than λ/3neff, it would be in favor of the lowest order TE modes lasing[24]. The calculation shows that the free spectral range (FSR) is approximately 32.9 nm based on an expression of ΔλFSR ≈ λ2/2πneffR, which is close to the measured 30.32 nm (see Fig. 4(a)). Other weaker emission lines should be related to the higher-order radial modes. In addition, the obtained Q factor is approximately 1951 for the three-layer QD microdisk, which is far below that of the silica microdisk[25, 26], due to the side wall roughness of the GaAs microdisk. Therefore, in order to obtain a high Q factor, polishing the side wall of the GaAs microdisk is necessary.

class="figure_img" id="Figure4"/>
Download
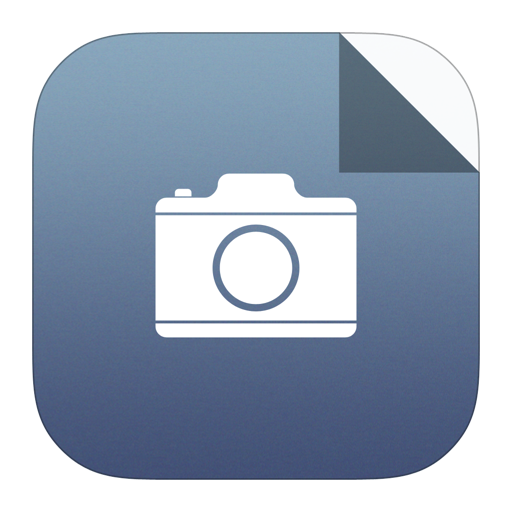
Larger image
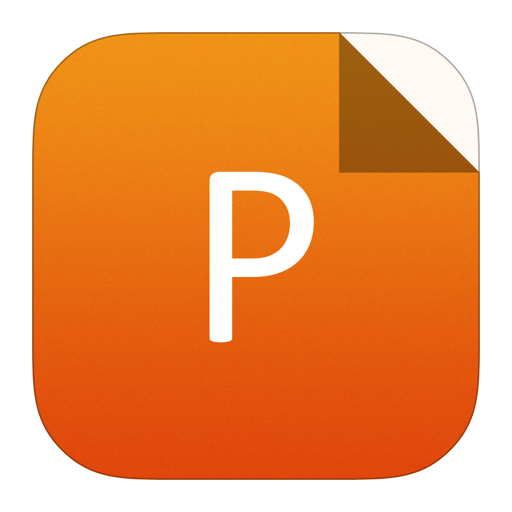
PowerPoint slide
Figure4.
(Color online) Three-layer stacked QD microdisk sample. (a) PL emission spectra under different pump powers. The two lasing mode peaks studied are 1131.69 and 1162.01 nm, respectively, which correspond to TE29, 1 and TE28, 1 cavity modes. (b) Light-out versus light-in curves of the lasing mode peaks of 1131.69 and 1162.01 nm, corresponding to the lasing thresholds of 40.08 and 27.66 μW, respectively. Inset shows the FWHM of the peak of 1131.69 nm as a function of pump power. (c) Light-out versus light-in curve with the double logarithmic scale for both lasing mode peaks. (d) Calculated electric field distribution of TE29, 1 cavity mode.
Compared to the two-layer stacked InAs/GaAs QD sample, it is found that the three-layer stacked InAs/GaAs QD sample has a lower lasing threshold. Note that the laser threshold depends on both the density of the quantum states of emission material and the Q value of the microcavity[27]. Here, two- and three-layer stacked QD samples have similar Q values. This leads to an observed lower laser threshold of the three-layer stacked QD sample owing to the fact that it has a larger density of quantum states.
4.
Conclusion
In conclusion, III–V/silicon heterogeneous integrated WGMs QD microdisk lasers have been demonstrated at room temperature through optical pumping. The microdisk diameter is 5 μm with two-layer or three-layer InAs/GaAs QDs active layers. The lowest laser threshold obtained is approximately 28 μW, which can be further reduced by improving the optical quality of the GaAs microdisk. The experimental results show that the bonding technique is an efficient method to integrate an InAs/GaAs QD active layer on the SiO2/Si chip for fabricating silicon based light sources.