1.
Introduction
The rapid expansion of wearable electronic devices has greatly promoted the development of flexible and portable energy conversion and storage devices. Amongst them, a new type of fiber-shaped energy storage device has received extensive attention and rapid development, which provides a reliable strategy to leap over the geometrical restrictions of traditional planar energy storage devices[1–3]. Compared with the conventional planar devices, fiber-shaped devices deserve better flexibility which are adapted to a variety of product designs, especially woven with the ordinary fabric. The leap forward in progress of energy storages devices is always accompanied by the revolution of electrode technology. Similar to the planar energy devices, fiber electrodes play pivotal roles effecting the performance of fiber-shaped energy storages devices. It is no exaggeration that carbon nanomaterials are currently the most popular material preferred for electrodes since its intrinsic outstanding conductivity and diversity in architecture. Among which, carbon nanotube fibers and graphene fibers are considered to be the most promising fiber-shaped electrodes for wearable electronics due to their excellent electrical conductivity, flexibility and mechanical strength. Recent research in the carbon nanomaterials field points to diverse possible applications, such as electronic devices and interconnects, field emission devices, electrochemical devices, nanoscale sensors, electromechanical actuators, separation membranes, filled polymer composites, drug delivery systems and so on. In this review, we mainly explore the state-of-the-art applications of carbon nanotubes and graphene on flexible energy storage devices for widely used wearable electronic products, including the fiber-shaped supercapacitors (FSC) and fiber-shaped lithium-ion battery (FLIB). To this end, recent advances in the fabrication, structure, mechanism, and properties of fiber-shaped energy storage devices are summarized here, with the focus on designing the electrode material with high performance. We further discuss the status of this field and point out the value-added applications that exist today versus the revolutionary applications that will ensue in the distant future.
2.
The applications of carbon-based fiber electrodes in FSC
Carbon nanotube (CNT) fiber has a unique flexible fiber structure with high specific surface areas and excellent electrochemical properties, which make it an ideal electrode material to replace the traditional metal wire electrode. In 2003, Dalton et al. used a wet spinning method to prepare CNT fiber, which can be employed as fiber electrode to fabricate FSC. Although the capacitance was only 5 F/g, it created a precedent that the carbon-based fiber can be used to prepare the flexible fiber-shaped storage devices[4]. The low electrochemistry properties were due to the presence of surfactants and macromolecules added in the preparation of CNT fiber. Recently, high performance CNT fibers had been prepared by dry spinning technology from the spinnable CNT array[5, 6]. The dry spinning CNT fibers were highly ordered and had excellent structure, strength and conductivity, therefore it indicated potential applications in the development of fiber-shaped energy devices[7]. Peng and his co-workers firstly used the dry spinning aligned CNT fiber to fabricate all-solid-state FSC by placing two identical fiber electrodes in parallel or winding, which showed excellent flexibility and cyclic stability[8]. Besides the fiber electrodes, the performance of FSC can be improved by developing new device structure. Chen et al. assembled the FSC into a core-shell structure based on carbon nanotube fibers and films as two electrodes, which provided an effective pathway for ions or electrons, thus delivering stable charge-discharge cycles at a relative high rate with the capacity of 59 F/g (Fig. 1(a)), which was higher than that of the winding structure capacitance with 4.5 F/g[9].
As a new member of carbon nanomaterials, graphene has been widely employed as electrode materials for energy storage devices, which also can be used to construct FSC. Great efforts had been devoted to realize the preparation of high performance graphene fiber electrodes by wet spinning technology. Firstly, graphene oxide (GO) suspension was injected into a specific coagulation bath, and the GO sheets were aggregated into continuous GO fibers. The traction in the collection process can further improve the orientation of the graphene sheet in GO fiber. After being chemically reduced by hydriodic acid, graphene fibers with high conductivity and strength can be obtained[10, 11]. Similarly, graphene fiber can be prepared through injecting the GO suspension into a capillary and hydrothermally treating it at 230 °C for 2 h[12]. The as-prepared graphene fibers have good flexibility and high electrical conductivity, which were applicable as electrodes for FSC. Meng et al. prepared a full-graphene fiber electrode with core-shell structure with graphene fibers served as the core and 3D porous graphene skeleton as the shell based on hydrothermal and electrochemical reductions (Fig. 1(b)). This hierarchical graphene hybrid fiber structure combined the high conductivity of graphene fibers and the high exposed surfaces of 3D graphene networks, thus offering great advantages as fiber electrodes for high performance FSC[13]. An all-in-one FSC based on graphene fiber has been prepared by a controllable laser reduction method. Both sides of the GO fiber were reduced into graphene to act as the two electrodes of the supercapacitor, while the non-reduced GO in the middle part was used as the diaphragm (Fig. 1(c)). After adding the electrolyte, the all-in-one FSC can be integrated onto a single graphene fiber[14].
Gao and co-workers had pioneered the direction on the preparation of graphene fiber by the wet-spinning method, and expanded the application of graphene fibers by designing novel structures or incorporating hybrid contents. At first, they demonstrated that GO sheets can form chiral liquid crystals in the GO aqueous dispersions (Fig. 2(a)), which can be wet-spun to continuous graphene oxide fiber. After being chemically reduced, neat graphene fiber without any supporting polymer can be fabricated[10, 11, 15]. The graphene sheets can be aligned in the axial direction to provide strong interactions between sheets, which determined the mechanical strength of graphene fiber (Fig. 2(b)). The combination of high conductivity, flexibility, high strength, and unique porous structure made the graphene fiber highly attractive in many applications, especially in the fiber-shaped electronic devices. The capacity of FSC prepared singly by using CNT fiber or graphene fiber as electrodes was still limited. The energy storage mechanism of carbon based super capacitors is mainly the double layer of energy storing, that means, the energy stored in the double layer electric field between the electrode and electrolyte. Therefore, increasing the specific surface area of the electrode can effectively improve the capacity of the device. Subsequently, they fabricated a conductive, lightweight and porous graphene aerogel fiber by combining the wet-spinning technology and ice-templating strategy (Fig. 2(c)). This porous graphene fiber had a unique core-shell structure and the high porosity enabled this aerogel fiber to be greatly useful in fiber-shaped energy storage devices[16]. Additionally, it is easy to incorporate other materials in the graphene fiber through the wet-spinning method, which can greatly improve the capacitance of the FSC. Gao and co-workers further chose metal oxide, bismuth oxide nanotubes as the hybrid component to prepare the high performance graphene fiber. This fiber can be directly employed as an electrode to fabricate FSC[17]. Developing such a co-spinning method would be a simple strategy for designing composite graphene fiber with high electrochemical performance for practical applications.

class="figure_img" id="Figure2"/>
Download
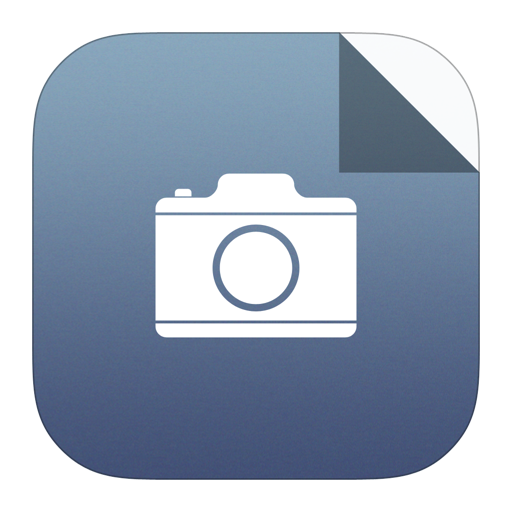
Larger image
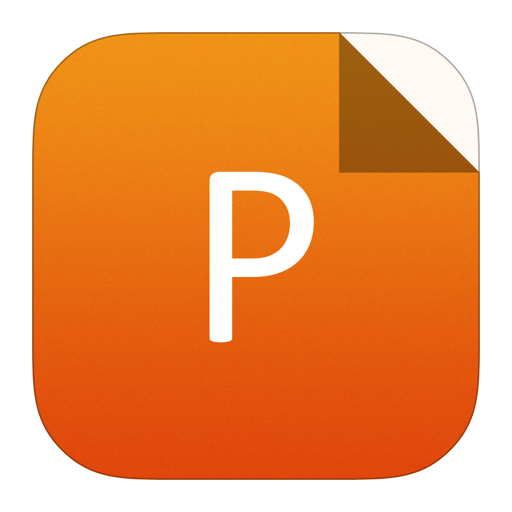
PowerPoint slide
Figure2.
(Color online) (a) Proposed model for one pitch of GO CLCs. (b) Four-meter-long GO fiber wound on a Teflon drum (diameter, 2 cm). (c) SEM images of fracture morphology of the porous graphene fiber. (d) I–V curves of GMF and GCF in a three-electrode system (vs. Ag/AgCl).
In addition, the improvement of the conductivity of the fiber electrode can reduce the internal resistance of energy storage devices, which is benefited to enhance the power density of the device. CNT fibers prepared by chemical vapor deposition have high electrical conductivity, while graphene prepared by chemical reduction-oxidation has a high specific surface area. Therefore, CNT/graphene hybrid fibers can combine the high conductivity of CNT and high specific surface area of graphene.
Recently, researchers developed a variety of methods, such as double-direction crimping[18], chemical vapor deposition secondary growth[19] and solution co-spinning[20, 21] to fabricate hybrid fibers based on various carbon nanomaterials. The synergistic effect had effectively improved the electrochemical properties of the fiber electrode for FSC. For example, Ren et al. prepared CNT/mesoporous carbon hybrid fiber electrodes by the double-direction crimping method, and the capacitance of the FSC was increased about 20 times by controlling the addition of mesoporous carbon[22]. Additionally, pseudocapacitive materials such as metal oxides and conducting polymers can also be introduced into the fiber electrodes to increase the capacitance and energy density of FSC. Transition-metal oxide can be deposited on the surface of CNT fibers or graphene fibers by electrochemical deposition, which can greatly enhance the pesudocapacitance of FSC[23–25]. Researchers obtained a variety of conductive polymer composite fiber electrodes by polymerizing conductive polymers (poly-(3, 4-ethylenedioxythiophene) (PEDOT)[26], polypyrrole (PPy)[27] and polyaniline (PANI)[28, 29] onto the surface of the CNT fibers or graphene fibers through the electrochemical polymerization method. Wang et al. designed a one-step hydrothermal strategy to prepare graphene/molybdenum disulfide (MoS2) hybrid fibers with an intercalated nanostructure (Fig. 1(d)). The hybrid fiber electrodes combined the high electrical conductivity from graphene sheets and high pesudocapacitance from MoS2 sheets, resulting in a high specific capacitance of 368 F/cm3[30].

class="figure_img" id="Figure1"/>
Download
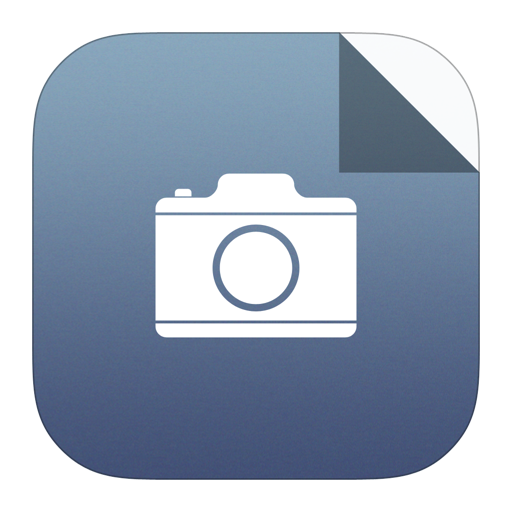
Larger image
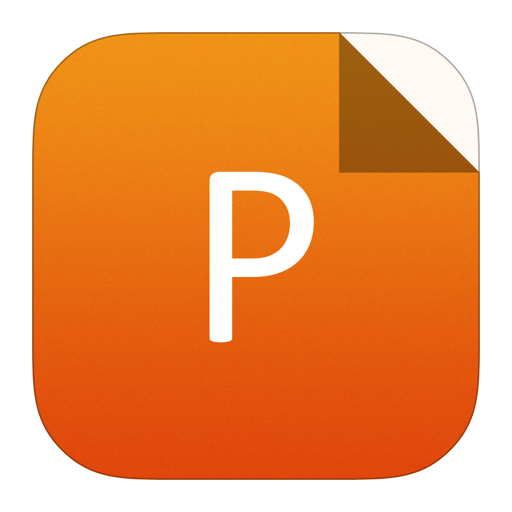
PowerPoint slide
Figure1.
(Color online) (a) Schematic illustration to both the cross-sectional structure and mechanism for the high electrochemical properties of the coaxial FSC. (b) Schematic illustration of the FSC (down) fabricated from two twined GF@3D-G fibers (up) with polyelectrolyte. (c) Schematic illustration of the laser reduction of GO fiber for the preparation of RGO-GO-RGO fiber (up) and the all-in-one FSC (down). (d) Schematic illustration to the intercalated nanostructure of the graphene/MoS2 hybrid fiber electrode for FSC.
The working voltage of the FSC can not only improve the energy density but extend a wider range of applications. At present, phosphoric acid/polyvinyl alcohol electrolyte was used to prepare fiber-shaped symmetrical supercapacitors, while the working voltage was low (0.8–1.0 V). Due to the excellent electrochemical property of pseudo-capacitance electrode material, the combination of pseudo-capacitor and double-layer capacitor can produce hybrid supercapacitors (also known as asymmetric supercapacitors), which give a higher operating voltage and energy density. Zhenget al. prepared a fiber-shaped hybrid supercapacitor, which explored the graphene fibers loaded with MnO2 as the anode material and graphene/CNT hybrid fibers as the cathode material. The operating voltage of this hybrid FSC reached to 1.6 V (Fig. 2(d))[31]. On the basis of this, Yu et al. also used MnO2 as the fiber cathode material and nitrogen-doped graphene/CNT hybrid fiber as the cathode material to increase the working voltage to 1.8 V[32].
3.
The fabrication and application of multi-functional FSC
With the development of carbon-based fiber electrodes for preparing flexible FSC, researchers gradually focused on designing functional and intelligent fiber-shaped energy storage devices to extend the practical applications. Typically, stretchable FSC has been developed to overcome the low elongation at the break of CNT or graphene fibers. A normal FSC was wrapped with a pre-stretched silicone rubber (PDMS) fiber. After the pre-stretched fiber was removed, a stretchable FSC was obtained[33]. However, this device was composed of different components, which was easy to separate in the repeated deformation and reduced the flexibility of the FSC. Yang et al. prepared a highly stretchable FSC with PDMS fiber as the substrate, phosphoric acid/polyvinyl alcohol as the solid electrolyte and oriented CNT film as the electrode (Fig. 3(a)). This stretchable FSC remained 95% after 100 cycles of stretching (75% elongation), showing good elasticity and cyclic stability[34]. Through optimizing the material and structure, a super-stretchable FSC with higher capacity (~80 F/g), greater stretch ratio (400%), and better tensile cycle stability (300%, 5000 cycles) can be prepared[35]. Different from elastic fibers as a substrate, a spring-like CNT fiber electrode was prepared with twisting technology by Zhang et al., whose unique spiral structure gives the fiber electrode a higher elongation at the break (300%). This pure CNT-based stretchable supercapacitor remains about 94% of the initial capacity after 300 cycles of stretching[36].

class="figure_img" id="Figure3"/>
Download
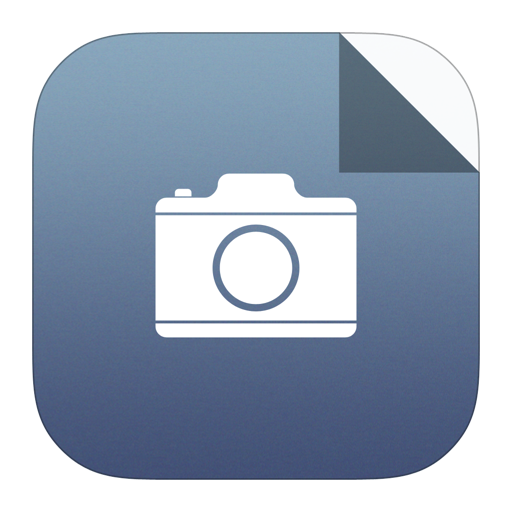
Larger image
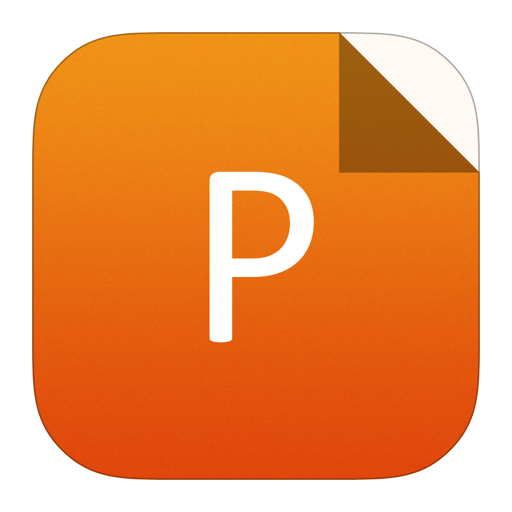
PowerPoint slide
Figure3.
(Color online) (a) Schematic illustration to the fabrication of a highly stretchable FSC with a coaxial structure. (b) Schematic illustration to the self-healable FSC. (c) An electrochromic FSC with a different color in response to different potential. (d) A shape-memory FSC under a different state.
Although the stretchable FSC has good flexibility and extensibility, the fiber electrode still has the problem of fracture in practical application. Sun et al. developed a novel fiber electrode with self-healing function by wrapping conducting CNT films around polymer fibers, which can be used to fabricate a self-healable FSC (Fig. 3(b)). After the fiber electrode has broken, the cross-section of the fiber electrode re-contact and the polymer fiber matrix will re-heal into a fiber in a short time. The specific capacitance of the self-healable FSC was restored to 92% after self-healing. The reversible breaking and healing process could be repeated several times with stable performances[37]. The multi-functional and intelligent FSC can expand the application of the wearable energy storage devices. Chen et al. described an electrochromic electrode by electro-depositing polyaniline (PANI) on CNT fiber, which can be used to fabricated electrochromic FSC. During the charge-discharge process, the electrochromic electrode can change color between blue, green, and light yellow in response to the different potentials (Fig. 3(c)). These electrochromic FSC are promising for display applications in addition to powering various portable and wearable electronic devices[38]. Deng et al. designed a novel fiber electrode by winding aligned CNT sheets on a shape-memory polyurethane substrate, which can be employed to fabricate shape-memory FSC. The deformed shapes of FSC under bending and stretching can be fixed as expected and recovered to the original shape when required (Fig. 3(d)). Moreover, the electrochemical performances of the FSC can be well-maintained during deformation, at the deformed state and after the recovery[39]. Sun et al. fabricated a FSC by mimicking the in-series units to produce high working voltages inspired by electrocytes in the electric eel. The working voltage of the device was determined by the numbers of electrochemical capacitor units, which can be readily increased by increasing the number of in-series devices. This structure can prevent the used of conventional conducting wires and a high output voltage up to 1000 V can be realized in this work, which was the highest voltage among the wearable energy storage devices[40].
4.
The development of fiber-shaped lithium ion battery (FLIB)
A great deal of effort has been devoted to developing new materials, optimizing structures, and improving the electrochemical properties of fiber-shaped energy storage device. Among which, Peng’s group at Fudan University has developed a new family of FLIB based on aligned CNT fibers. Firstly, they deposited MnO2 on the aligned CNT fibers to function as the anode, and then conjugated with the cathode of lithium wire to fabricate the FLIB without metal current collector and binder (Fig. 4(a)). Since the aligned CNT/MnO2 composite fiber electrode has excellent mechanical and electrical properties, the FLIB showed good electrochemical performance[8]. A carbon nanotube/silicon composite fiber electrode was fabricated based on electron beam evaporation technology, which can effectively combine the high specific capacity of the silicon and high electrical conductivity of the CNT. This composite fiber electrode can be used to fabricate FLIB (Fig. 4(b)), which exhibited desired flexibility and good electrochemical performance[41]. However, the metal lithium wire greatly limits the practical applications of the FLIB. Therefore, the cathode of the composite fiber and the anode of the CNT/lithium manganese oxide (LMO) hybrid fiber were wound on the cotton fiber to prepare a flexible FLIB without metal lithium[42]. Subsequently, they used commercial cathode lithium titanate (LTO) as the active material instead of silicon to prepare the fiber-shaped LTO-LMO battery, which resulted in the capacity of 138 mAh/g (Fig. 4(c))[43]. On this basis, two aligned CNT composite fiber electrodes with anode and cathode materials were parallel wrapped onto the PDMS fiber to fabricate the super-stretchy FLIB with a strain of up to 600% (Fig. 4(d))[44].

class="figure_img" id="Figure4"/>
Download
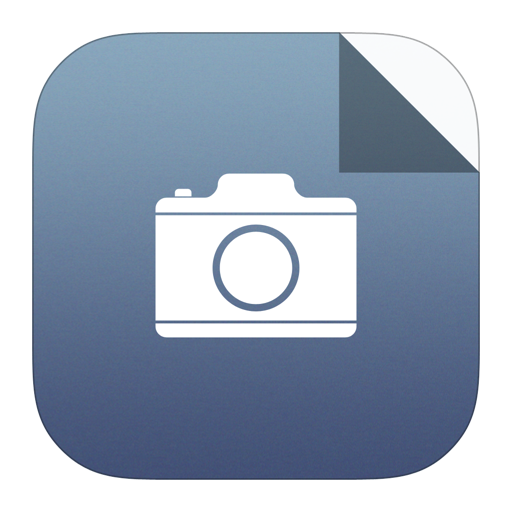
Larger image
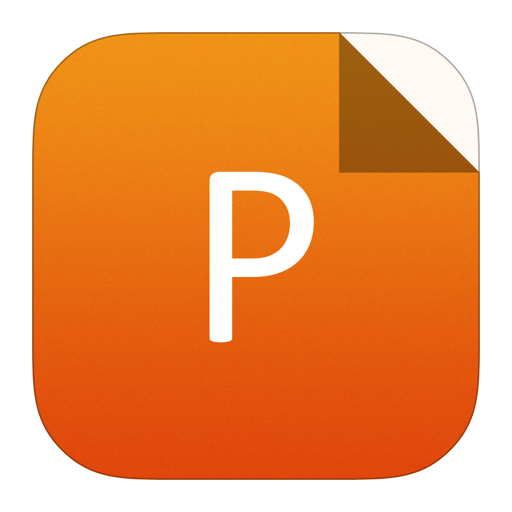
PowerPoint slide
Figure4.
(Color online) (a) Schematic illustration to the FLIB fabricated by twisting an aligned CNT/MnO2 composite fiber and Li wire. (b) Schematic illustration to the preparation of the aligned CNT/Si composite fiber electrode used for FLIB. (c) Schematic illustration of the full FLIB prepared by CNT/LMO fiber and CNT/LTO fiber. (d) Schematic illustration of the fabrication of the super-stretchy FLIB.
Besides the traditional supercapacitors and LIB, several new kinds of fiber-shaped energy storage devices such as lithium-sulfur batteries, zinc-air batteries, lithium-air batteries and aluminum-air batteries have been prepared to further enhance the performances and applications of fiber-shaped energy storage devices. Fang et al. developed a fiber-shaped lithium-sulfur battery by using carbon-based hybrid fiber electrodes. Mesoporous carbon (MC) particles were chosen as the host materials to reserve sulfur, and the aligned CNT fibers were employed as a fiber skeleton to embrace the sulfur-encapsulated MC particles through the twisting process. The combination of multidimensional carbon nanomaterials can prevent the loss of sulfur during the charge-discharge process (Fig. 5(a)). So, the high content of sulfur enclosed in the fiber electrode can greatly enhance the performance of the fiber-shaped devices[45]. Recently, metal-air batteries have attracted a great deal of attention for long-term power supply in wearable devices because of the relatively high capacity. Due to the non-reactive zinc (Zn) metal and aqueous solvent, the Zn-air battery has been considered as a safe, highly effective and eco-friendly energy system. An all-solid-state cable-type flexible Zn-air battery with polymer electrolyte has been fabricated by using nonprecious metal catalyst to replace platinum (Fig. 5(b)). The electrocatalyst can effectively enhance the electrochemical performance of the metal-air battery. Additionally, the cable-type Zn-air battery can be successfully operated with external strain, which exhibited a promising application in flexible energy devices[46]. However, this cable-type Zn-air battery was not stretchable and wearable because of the large diameters (~8 mm). Xu et al. developed a fiber-shaped Zn-air battery by using CNT films as the air electrode and designing a spring structure. Aligned CNT films can be directly used as an air electrode in this fiber-shaped battery, and the battery showed stable electrochemical properties under bending and stretching conditions[47].

class="figure_img" id="Figure5"/>
Download
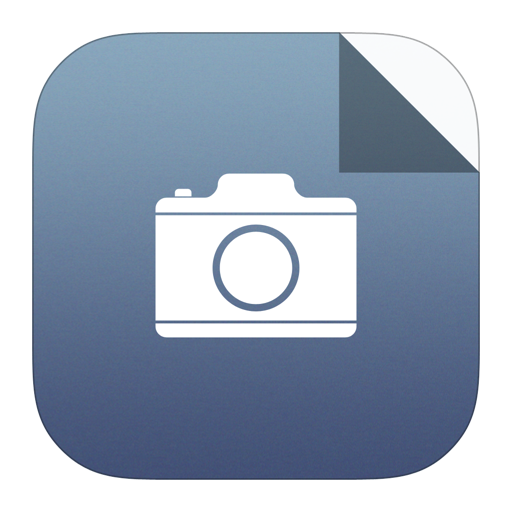
Larger image
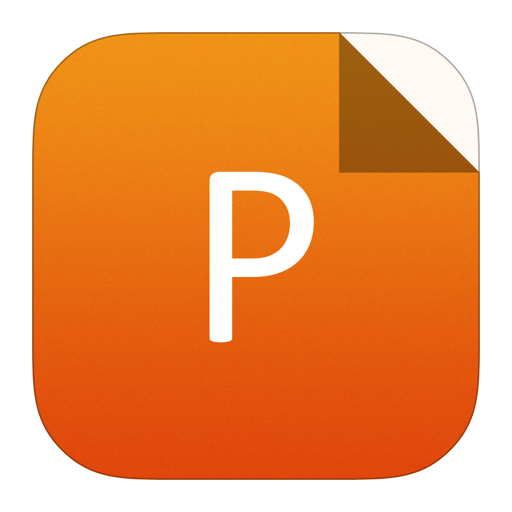
PowerPoint slide
Figure5.
(Color online) (a) Schematic illustration to fiber-shaped Li-S battery. (b) Schematic illustration to the cable-type Zn-air battery. (c) SEM images of the aligned CNT sheets with different angles. (d) Schematic illustration of the fabrication of the fiber-shaped Li-air battery.
Owing to the small thickness, good conductivity, light weight and flexibility, aligned CNT films can greatly enhance the flexible, stretchable and wearable performance of metal-air batteries (Fig. 5(c)). Subsequently, an all-solid-state fiber- shaped aluminum-air (Al-air) battery has been prepared by using cross-stacked aligned CNT sheets as the air electrode onto a spring-like Al wire[48]. The lithium-air (Li-air) battery has been proposed as a promising energy storage candidate with a high theoretical specific energy density of ~3500 W·h/K, which was 5–10 times higher than the commercial LIB. Zhang et al. employed aligned CNT films as an air electrode to fabricate a fiber-shaped Li-air battery by using gel polymer electrolyte, which can effectively work for 100 cycles in air and exhibit a cutoff capacity of about 500 mAh/g (Fig. 5(d))[49]. The above fiber-shaped metal-air batteries also showed high flexibility and safety, which were critical for modern wearable electronics.
5.
The application of flexible energy storage devices
The fiber-shaped energy storage devices based on carbon nanomaterials are attracting increasing attentions because of their unique advantages, such as flexibility, shape diversity, twisting ability and so on. These properties have enabled promising applications in portable and wearable electronic devices, for instance, soft electronic products, roll-up displays, and wearable textiles. Considerable efforts have been made to improve the performance of energy storage systems. Aiming at practical application, a stretchable wire-shaped lithium-ion battery was designed from two aligned multi-walled carbon nanotube/lithium oxide composite yarns. By winding the yarn cathode and anode around an elastic substrate, a stretchable battery with spring structure was obtained, which displays considerable capacity retention after stretching for 200 cycles at a strain of 100%[43]. The novel self-healable wire-shaped supercapacitor was fabricated, whose high specific capacitance of 140.0 F/g was restored to 92% after self-healing. The wire-shaped energy devices are particularly promising for wearable and portable electronics[37]. Inspired by the hierarchical neural network structure, large area supercapacitor textiles have been designed with a hierarchical conducting structure. The textile devices showed high capacitance, power and energy of 69.3 F, 80.7 mW, and 5.4 mW·h, respectively[50].
6.
Conclusion and outlook
Recent advances in fiber-shaped energy storage devices are described from the view point of the fiber electrode, with their electrochemical performances. The opportunities, challenges and the major bottlenecks, including large-scale manufacturing for carbon based material, will be identified as we define the applications space for wearable electronics, and much effort should be made. Researchers have devoted great efforts to explore new electrodes and active materials to enhance the performances fiber-shaped energy storage devices. However, these academic achievements have not yet been translated into industrial application. The pending issue resides in the industrialization and mainly includes two aspects.
Firstly, we should develop composite electrode materials and a fabrication method to prepare high-performance fiber electrodes. As flexible electrodes, the electrical conductivity must be further enhanced, reflecting the key achievements for high power and energy densities of fibrous devices. Composite electrode materials and the fabrication method should be developed to prepare high-performance fiber electrodes. Furthermore, scientists are finding ways to enhance the performance of the electrode by creating novel structures, such as the hybrid electrodes with conductive polymers or pseudo-materials and asymmetric structure. The cyclic number is also regarded as a critical index under repeated charge and dis-charge cycles. Benefitting from the delicate design of electrodes and an effective strategy, the latest lithium–ion battery and supercapacitor can run more than 2000 and 100 000 cycles, respectively. Strategies like modifying the electrolyte are also helpful to keep the performance insusceptible for repeated recharges.
Another challenge at the industrialization of fiber-shaped energy storage devices is the large-scale continuous preparation of fiber electrodes or devices for a commercially viable candidate for application. Scientists have found ways to address this problem by upgrading the electrode materials or fabrication strategies, by combining with designing dedicated equipment. Dalton and co-workers have prepared a 100 meter-long carbon nanotube fiber electrode by the wet spinning process, while the conductivity of the fiber electrode was limited. Subsequently, researchers developed wet spinning methods for continuous preparation of fiber electrodes with core-shell structure (Fig. 6(a))[21]. Besides, a nitrogen-doped graphene/CNT hybrid fiber electrode has been prepared by continuous hydrothermal strategy without post-treatment. These hybrid fibers can be directly used to assemble FSC (Fig. 6(b)). The disadvantage was that the hydrothermal process required a longer reaction time, so the production efficiency of the fiber electrode was low[51]. Wang et al. developed a synchronous deposition strategy to continuously fabricate FSC based on aligned CNT composite fibers (Fig. 6(c)). This continuous fabrication strategy was extendable to a variety of pseudocapacitive active materials such as RGO, MnO2, PANI and PPy, so it was available to continuously fabricate high-performance FSC with different pseudocapacitive composite fibers. This work also presented a general and effective strategy in the development of fiber-shaped energy storage devices aiming at a large-scale production[52]. More efforts should be paid to realize the industrial preparation and application of the fiber-shaped energy devices. Based on the graphene oxide solutions, Qu and co-workers developed a directly wet-spinning technology to fabricate the continuous supercapacitor fibers by using a customized microfluidic system. Through the microfluidic spinneret, they can directly assemble the different parts including two fiber electrodes and the gel polymer electrolyte into a whole fiber, which can directly fabricate the supercapacitor fibers without coating and twisting processes. This FSC has a sandwiched structure, where the rGO fiber electrodes were separated by the gel polymer electrolyte[53]. This method provided a sample way to realize the large-scale continuous production of fiber-shaped electronic devices. Nowadays, the fiber-shaped devices have been mainly woven into the textiles by hand, which prevented the large-scale production of wearable electronics.

class="figure_img" id="Figure6"/>
Download
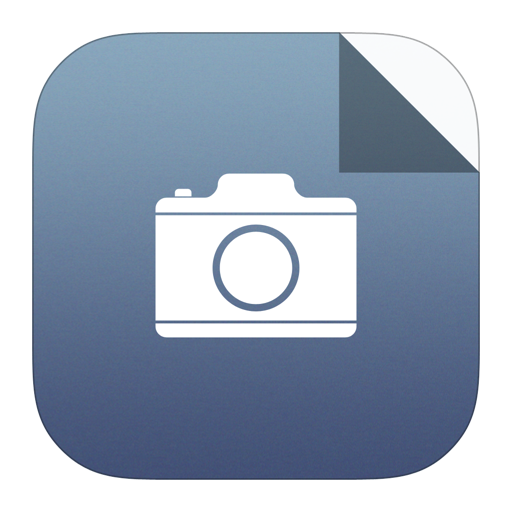
Larger image
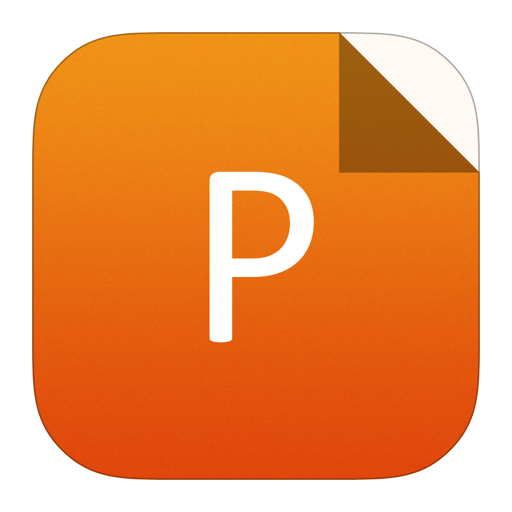
PowerPoint slide
Figure6.
(Color online) (a) Schematic illustration showing the coaxial spinning process of GO@CMC fiber. (b) Schematic illustration of the fabrication process of CNT/graphene hybrid fiber by hydrothermal method. (c) Schematic illustration of the experimental setup for the continuous fabrication of FSC.
To fully realize the commercial application of fiber-shaped energy storage devices, we also should develop efficient weaving techniques to produce flexible and wearable energy storage textiles. So, more efforts should be paid to overcome the above problems aiming to realize the industrial preparation and application of the fiber-shaped energy devices. To summarize, although much effort is needed to optimize the fiber-shaped energy storage devices in terms of carbon material based electrodes from structure to property, they represent a new family of energy-storage systems, which may change our life greatly in the near future.