

河海大学水文水资源与水利工程科学国家重点实验室 水文水资源学院,南京 210098
Validation of watershed soil effective depth based on water balance and its effect on simulation of land surface water-carbon flux
HUANGRichao

通讯作者:
收稿日期:2016-01-19
修回日期:2016-03-24
网络出版日期:2016-05-25
版权声明:2016《地理学报》编辑部本文是开放获取期刊文献,在以下情况下可以自由使用:学术研究、学术交流、科研教学等,但不允许用于商业目的.
基金资助:
作者简介:
-->
展开
摘要
关键词:
Abstract
Keywords:
-->0
PDF (1827KB)元数据多维度评价相关文章收藏文章
本文引用格式导出EndNoteRisBibtex收藏本文-->
在全球变化背景下,水文学正从以单一水循环过程为主要研究对象,向以水分、能量与物质耦合循环的生态水文为主要研究内容的综合性、交叉性学科发展[1],研究尺度从流域尺度向区域和全球尺度拓展。目前,已发展了一系列区域和全球尺度的陆面模型,用以模拟和预测陆面蒸散发量、径流以及植被吸收和释放碳通量等。在全球动态植被模型中,LPJ模型(Lund-Potsdam-Jena Dynamic Global Vegetation Model)被广泛应用于气候变化背景下植被变化和水碳通量变化研究[2-8]。该模型由Sitch等[8]基于过程的平衡态陆地生物圈模型(BIOME模型系列[9])框架,耦合了具有机理性的陆地植被动态、碳和水模型发展而来,之后其功能不断得到扩展,如用于生态系统水、碳和营养物质模拟的动态植被—生物地球化学耦合模型LPJ-TEM[10],用于模拟全球碳循环的植被动态与大气模型耦合模型[11],以及用于湿地水文及CH4通量研究的LPJ-WHYME[12]等。
在LPJ模型以及其他陆面模型中,水平衡是区域和全球水分循环及其与能量、物质循环耦合的基础和纽带,水平衡方程通常表述为下列形式:
式中:P为降水量;ET为实际蒸散发量;R为径流量;W为土壤蓄水量,小于等于土壤最大持水量或蓄水容量WM。上式各项一般采用单位面积水量,即水深(mm)。当P超过ET和土壤缺水量之和,即P > ET+(WM-W),产生自由水,即形成(地表、地下)径流量R。因此,WM是模型产流量计算的重要参数。
WM定义为地表以下某一深度内植被能够吸收的最大水分[13-14]:
式中:θf、θr分别为田间持水率和凋萎含水率;he为土壤有效深度或厚度。WM取决于土壤特性和植被等因素;θf、θr取决于土壤质地、有机质·含量等特性;he在植被覆盖区取决于植被根系发育深度,在裸土地区取决于土壤特性[14]。联合国粮农组织和联合国教科文组织(FAO/Unesco)[15]早在1971-1980年根据106种土壤类型的颗率组成、土壤厚度分布推求WM分布。Dunne[16]通过综合分析全球土壤质地、土壤有机质含量、植被根系深度和土壤厚度,估算出全球0.50×0.50网格WM分布,并得出全球(除格陵兰岛、南极洲和裸地外)WM平均值为8.6 cm,30%、60%、89%以上区域分别低于5 cm、10 cm、15 cm。中国著名的新安江模型与陕北模型创始人赵人俊教授研究认为[17],WM在不同气候区存在差异,在湿润地区较小,在干旱区较大,如长江流域WM在12 cm左右,在燕山地区与东北东部,在16 cm左右。
土壤有效厚度he及蓄水容量WM变化对水、碳通量影响显著,如Milly[18]研究表明,当全球平均WM从4 cm增加到60 cm,全球平均蒸散发量增加36%,径流减少35%;Feddema[19]认为土壤中水分亏缺与WM减少成正比,且湿润期WM对径流影响以及干旱期WM对蒸散发影响尤为显著。然而,LPJ模型以及其它大尺度陆面模型中,大都采用某一特定土壤深度(he)进行全球或区域陆面水、热平衡以及通量计算,如在LPJ中he设置为150 cm[5]、SSiB中设置为160 cm[20]。LPJ模型应用于全球不同地区水碳动态变化模拟结果表明,不同地区采用同一he值会出现模型计算的水量平衡误差问题[5]。因此,需要根据不同区域特性对he进行修正,并探索其对WM和陆面水碳等通量的影响。
目前,通过实测资料确定he的方法主要有两种,一是根据土壤剖面实测含水率变化数据进行估算,如Ladson等[14]收集澳大利亚180个土壤剖面实测含水率,采用土壤剖面中含水率变化的最大深度确定he,并结合最湿、最干时土壤含水率确定WM;另一种是综合考虑植被根系深度分布、土壤厚度以及空间比例估算he[16],该方法研究表明,仅考虑单一下垫面因素会导致估算的he存在较大误差,如Ladson等[14]根据植被根系发育深度,森林植被区he值可深至1200 cm,草地和作物区he值可深至200 cm;但Dunne[16]认为在网格尺度内如果考虑裸土和根系之间比例,he值显著降低。这两种方法需要详细的植被(覆盖度、根系分布)、土壤(含水率)等观测资料,在缺乏可靠的观测资料地区he估值误差还很大[14]。由式(1)可知,水量平衡可综合考虑不同气候及下垫面特征下土壤有效厚度he(或蓄水容量WM)对蒸散发、径流等影响,因此,本文根据流域实测降水量、径流量以及潜在蒸散发量资料,并结合流域植被、土壤特性以及实际蒸散发(如植被蒸腾、土壤蒸发)计算方法,计算出不同he和WM下径流深,再根据实测径流深与模型计算径流深之间误差最小,反求he和WM,以修正原LPJ模型中假设的固定常数。
为此,本文选取位于中国不同气候区的3个典型流域,以多年水量平衡为目标,对3个典型流域LPJ模型土壤有效厚度进行修正。在此基础上,对比分析土壤有效厚度修正前后土壤蓄水容量及LPJ模型模拟的流域陆面通量(径流R,实际蒸散发ET和净初级生产力NPP)差异,为提高该模型在不同气候区应用可靠性提供参考依据。
1 方法
1.1 LPJ全球动态植被模型及水—碳计算方法
LPJ模型以碳平衡和水量平衡为基础,逐网格演算陆地植被动态、土壤和大气之间的水—碳交换动态过程[5]。该模型中每个网格内水平衡模型计算框架如图1所示,其中,潜在蒸发量计算采用Priestley-Taylor公式[5],实际蒸散发量ET[5]为植被蒸腾Et、土壤蒸发Es和植被截留Ei三者的总和,且土壤蒸发假设只发生在表层20 cm土层内。产流计算采用简单的水箱模型,土壤层分为两层(上层厚度d1为50 cm,下层厚度d2为100 cm),其上下层水量平衡公式如下:式中:
总产流量R包括地表径流量R1、地下径流量R2以及下层渗漏量P2,计算如下:
式中:fwhc1、fwhc2分别为上下层土壤最大持水量(田间持水率减去凋萎含水率);d1、d2分别为上下层土壤有效厚度(cm);R为总径流量(mm d-1);w1、w2分别为上下层土壤含水量与各层最大持水量的比值;由于网格之间不考虑侧向水流交换及汇流路线,在月水量演算中本文假定计算时段内的网格水量都汇到流域出口断面。
LPJ模型中的碳循环模块模拟CO2在植被、土壤和大气之间的交换过程[4]。其中,净初级生产力NPP是植被净的碳获取量,为总初级生产中获得的碳与植物呼吸所释放的碳之间平衡量[2],表达式如下:
式中:GPP为总初级生产力(g C m-2);Rm为所有植被功能类型维持所需要的呼吸作用(g C m-2)。在GPP计算中,水分胁迫通过冠层导度参数与光合作用发生联系。
1.2 评价指标
由式(3)~(7)可知,模型中水量平衡计算主要取决于上下层土壤蓄水容量(即式(5)(6)中fwhc1×d1、fwhc2×d2),其中,fwhc1、fwhc2可根据土壤特性给定,因此,模型待率定参数为d1、d2。本文以实测与计算月径流深相对误差ER为目标函数,率定上下层土壤有效深度(d1、d2),并统计Nash-Sutcliffe效率系数ENS对模型模拟的月径流过程进行评价。ER、ENS计算公式如下:式中:
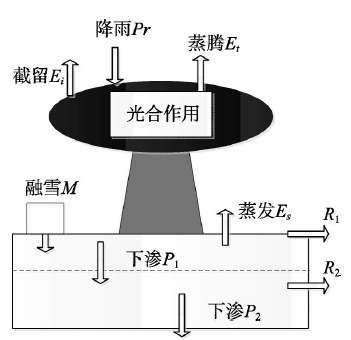
图1各网格内水量平衡计算框架
-->Fig. 1The frame of the water balance computed for each grid
-->
2 研究区概况与数据
本文选取位于中国不同气候区的3个典型流域:泾河流域(张家山站以上)、淮河流域(蚌埠站以上)、东江流域(博罗站以上)(图2),基本特性如表1所示。由表1可以看出:① 不同气候区流域年均降水量差异较大。位于南方湿润地区的东江流域年均年降水量是半湿润和湿润的淮河流域2倍多,是半湿润、半干旱的泾河流域4倍多;② 各流域的径流差异比降水差异更为显著,东江流域年径流是淮河流域的3.7倍,是泾河流域的23.7倍;③ 反映气候干旱程度的干旱指数从泾河流域的1.86,减少到淮河流域的1.17、东江流域的0.59。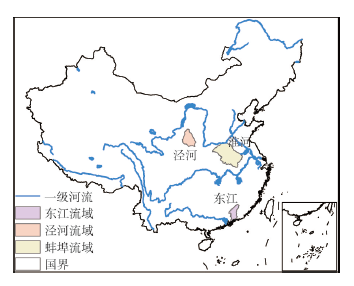
图2研究区位置图
-->Fig. 2The location of the study regions
-->
从下垫面特征来看,泾河流域森林等高覆盖植被主要集中在流域南部边缘山区,位于北部上游盐池、定边等县的黄土高原沟壑区植被稀少,土质疏松。该流域土壤以黄绵土和黑垆土为主,黄土高原区土壤最大厚度达180~200 m,由于暴雨集中,水土流失严重[21]。淮河流域天然植被以落叶阔叶林与针叶松林的混交林为主,土壤主要为潮土、沙姜黑土和水稻土。东江流域内植被属南亚热带季雨常绿阔叶林和南亚热带草被以及人工营造的针叶林,常年青绿,大部分山地、丘陵已基本绿化[22],流域土壤以赤红壤、红壤和水稻土为主[23]。
本文采用1901-2000年0.5°
Tab. 1
表1
表1流域特征表
Tab. 1List of basin characteristics
水系 | 测站 | 流域面积 (km2) | 年均降水量 (mm) | 年均径流量 (mm) | 年径流系数 | 干旱系数 | 气候 |
---|---|---|---|---|---|---|---|
泾河 | 张家山 | 43216 | 454 | 40 | 0.09 | 1.86 | 半湿润、半干旱 |
淮河 | 蚌埠 | 121330 | 863 | 256 | 0.30 | 1.17 | 半湿润、湿润 |
东江 | 博罗 | 25325 | 1827 | 947 | 0.52 | 0.59 | 湿润 |
新窗口打开
3 结果分析
3.1 基于水量平衡的土壤有效厚度反演
基于水量平衡反演土壤有效厚度的具体方法为:设定式(4)(5)中d1变化范围为20~130 cm、变化间隔为10 cm,d2变化范围为10~300 cm、变化间隔为10 cm;选择d1、d2不同组合情形,模拟1956-2000年期间不同d1、d2组合下径流深,统计月径流深模拟值与实测值之间误差ER值;选取ER值最小时对应的d1、d2值为模型推求的上下层土壤有效厚度值he。以泾河流域为例(图3,表2)可以看出,d1在60 cm、d2在80 cm组合下(图3中实线对应值)ER最小为0.1%、ENS值接近最大为47.3%。与模型中原采用的d1 = 50 cm、d2 = 100 cm厚度组合(图3中虚线对应值)相比(ER = 10.0%,ENS = 45.9%),he修正后水量平衡误差显著减少。其他两个流域也采用相同的方法,最终3个流域的he修正值及模型模拟误差和效率系数等结果如表2所示。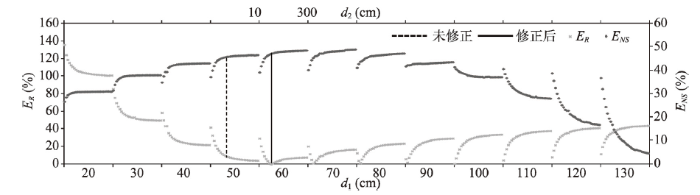
图3泾河流域相对误差ER和效率系数ENS随上下层土壤有效厚度变化(横轴上每两个刻度之间均为同一d1值,d2从左往右依次增大(10~300 cm))
-->Fig. 3Efficiency coefficient and relative error against different effective depths of soil in the Jinghe River watershed (Between every two calibration values on the horizontal axis is the same value of d1, while d2 increases from left to right (10-300 cm))
-->
Tab. 2
表2
表2有效土壤厚度、蓄水容量率定结果及模拟与实测径流误差统计
Tab. 2The calibration result of effective thickness, soil water storage capacity and statistical error of simulated and measured runoff
流域 | 泾河 | 淮河 | 东江 | ||||||
---|---|---|---|---|---|---|---|---|---|
修正前 | 修正后 | 修正前 | 修正后 | 修正前 | 修正后 | ||||
d1/d2 (cm) | 50/100 | 60/80 | 50/100 | 60/30 | 50/100 | 40/30 | |||
WM (cm) | 流域均值 | 22.1 | 20.6 | 22.1 | 13.2 | 20.4 | 9.5 | ||
变化范围 | 19.1~22.5 | 17.8~21.0 | 16.5~22.5 | 9.9~13.5 | 18.0~22.5 | 8.4~10.5 | |||
ER (%) | 10.0 | 0.1 | 3.0 | 0.1 | 4.8 | 0.6 | |||
ENS (%) | 45.9 | 47.3 | 53.8 | 54.6 | 60.9 | 58.0 |
新窗口打开
3个流域修正后的土壤有效厚度he(表中修正后d1和d2之和)在70~140 cm之间(表2),比原模型中he采用150 cm值小(表中修正前d1和d2之和)。对比he修正前后水平衡对比,3个流域模拟的水量平衡误差ER大幅减低,其中泾河流域ER改变最大,由10%减少到0.1%;东江流域ER由4.8%减少到0.6%;淮河流域ER改变最小,由3%减少到0.1%。模拟过程的效率系数ENS值在泾河、淮河流域略有提高,东江流域略有降低,效率系数ENS值变化不大主要是由于模型未考虑汇流过程以及水库调蓄等作用等。
由每个网格上土壤最大持水率与土壤有效厚度的乘积计算逐网格蓄水容量WM,再根据网格面积加权平均获得流域平均WM(表2)。he修正前3个流域平均WM都在20.0 cm以上,流域之间差异不大;he修正后WM平均值从湿润的东江流域的9.5 cm,增加到湿润、半湿润淮河流域的13.2 cm,进一步增加到半湿润、半干旱泾河流域的20.6 cm,这与现有的经验值相符合[17]。
3.2 陆面径流R、实际蒸散发ET、净初级生产力NPP通量模拟结果
土壤有效厚度he修正前后3个流域模拟的1956-2000年年径流深、蒸发量以及NPP结果对比如表3所示,可以看出,修正前后东江流域的R变化量最大,为50 mm;泾河流域R变化量最小,为-4 mm;淮河流域处于中间,为9 mm。对于年径流过程变化(变差系数Cv)来看,修正后土壤有效厚度he减小,径流过程变化程度却增大,其中,修正后的泾河流域年际变化最为显著,Cv增加了4%;东江流域年际变化最小,Cv增加了1.5%。Tab. 3
表3
表31956-2000年土壤有效厚度修正前后流域水、碳通量对比结果
Tab. 3The comparison of watershed flux of soil effective depth before and after correction during 1956-2000
泾河 | 淮河 | ||||||||||||||||
---|---|---|---|---|---|---|---|---|---|---|---|---|---|---|---|---|---|
通量 | 实测 (mm) | 未修正 | 修正后 | 实测 (mm) | 未修正 | 修正后 | |||||||||||
模拟 (mm) | ER (%) | Cv (%) | 模拟 (mm) | ER (%) | Cv (%) | 模拟 (mm) | ER (%) | Cv (%) | 模拟 (mm) | ER (%) | Cv (%) | ||||||
R | 40 | 44 | 10.0 | 69.0 | 40 | 0.1 | 73.0 | 256 | 247 | 3.0 | 38.3 | 256 | 0.1 | 39.5 | |||
ET | 414 | 406 | 0.98 | 11.5 | 410 | 0.97 | 11.6 | 608 | 611 | 0.5 | 6.8 | 603 | 0.82 | 7.3 | |||
NPP | 502 | 17.4 | 513 | 17.4 | 538 | 12.0 | 555 | 12.1 | |||||||||
东江 | |||||||||||||||||
通量 | 实测 (mm) | 未修正 | 修正后 | ||||||||||||||
模拟 (mm) | ER (%) | Cv (%) | 模拟 (mm) | ER (%) | Cv (%) | ||||||||||||
R | 947 | 891 | 5.9 | 29.5 | 941 | 0.1 | 31.0 | ||||||||||
ET | 880 | 931 | 5.56 | 4.0 | 882 | 0.23 | 4.7 | ||||||||||
NPP | 622 | 8.7 | 621 | 13.7 |
新窗口打开
从he修正前后3个流域模拟的多年平均实际蒸散发ET与水量平衡估算值(Pr-R)对比来看(表3),修正后ET相对误差ER都小于1%。修正前后3个流域的ET差异与R类似,东江流域ET差异最大,由修正前的931 mm减少到修正后的882 mm,相对误差由5.56%减少到0.23%;泾河流域ET改变最小,变化值为4 mm;淮河流域ET改变处于两个流域中间。从3个流域ET年过程Cv看(表3),修正后3个流域ET年际变化程度略有增大。
从修正前后LPJ模拟的NPP结果对比来看,淮河流域改变量最大,为17 gCm-2a-1;东江流域改变量最小,只有-1 gCm-2a-1;泾河流域改变量为11 gCm-2a-1。修正后东江流域NPP的Cv增加,其他两个流域Cv变化不大。
3.3 R、ET、NPP空间分布及其修正前后的变化
基于修正后的土壤有效厚度he,统计3个流域模拟的1956-2000年多年平均年径流深R及空间分布(图4),可以看出,3个流域年均径流深R都呈现由南往北递减的趋势。对比修正前后各网格R变化,泾河流域各网格修正后R值较未修正值普遍减少,南部减少较北部偏大,减小幅度在1.5~6.3 mm之间,面加权平均值减少4 mm。淮河流域修正后R值较修正前面加权平均值增加9 mm,北部部分网格R值较修正前减少,最大减小量18.0 mm;南部普遍增加,最大增加量60.1 mm。东江流域修正后R值较修正前面加权平均值增加50 mm,增加值空间变化范围为26.1~67.0 mm。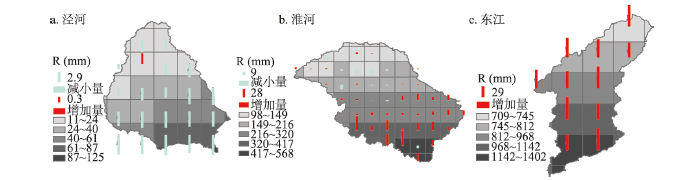
图4流域多年平均均径流深R空间分布及修正前后的变化
-->Fig. 4The spatial distribution of annual mean runoff and the difference before and after correction
-->
修正后3个流域多年平均实际蒸散发ET空间分布以及修正前后差异(图5)可以得出,泾河流域和淮河流域ET空间上呈现由东南向西北递减的趋势;东江流域中部最大,南北小,其中ET最大值位于新丰江大型水库区,这与吕乐婷等利用SWAT模型模拟的ET空间分布基本一致[26]。从修正前后各网格ET增减的分布差异来看,ET变化与R增减的空间分布相反,即泾河流域大部分网格修正后ET值增加,南部增量大,最大网格增量为6.1 mm;淮河流域北部某些区域相对于修正前增加,南部普遍减少,变化范围为-60~16.9 mm;东江流域全境相对于修正前减少量较大,减小幅度为25.6~66.6 mm。
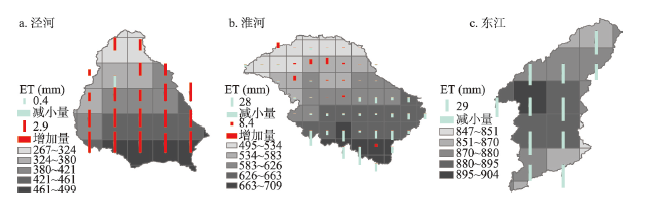
图5流域多年平均实际蒸发ET空间分布及修正前后的变化
-->Fig. 5The spatial distribution of annual mean actual evapotranspiration and the difference before and after correction
-->
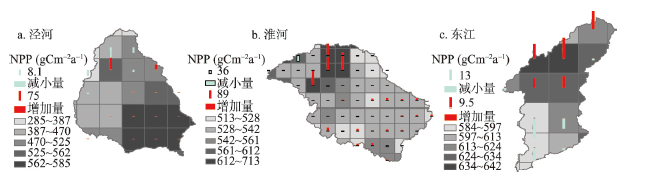
图6流域多年平均均净初级生产力NPP空间分布及修正前后的变化
-->Fig. 6The spatial distribution of annual mean net primary production and the difference before and after correction
-->
修正后的3个流域多年平均NPP空间分布差异较大(图6)。泾河流域NPP空间变化范围在285~585 gCm-2a-1之间,东南区域NPP明显高于西北;淮河流域NPP空间变化范围在513~713 gCm-2a-1之间,北部地区NPP相对其他地区大;东江流域NPP空间变化范围为584~642 gCm-2a-1,中北部NPP比两边高。分析修正前后各网格NPP变化,泾河流域和淮河流域北部NPP变化量较大,增加和减少较为明显,其中,泾河流域NPP增加最大值为150.7 gCm-2a-1,淮河流域NPP增加最大值为178 gCm-2a-1,南部基本不变;东江流域修正后较未修正变化幅度在-28.0~19.0 gCm-2a-1之间,空间上南部以减少为主,北部以增加为主。
4 结论及展望
(1)本文基于水量平衡对LPJ模型中土壤有效厚度进行修正,推求的东江、淮河、泾河流域土壤有效厚度分别为70 cm、90 cm和140 cm,比原模型中采用的150 cm厚度小。土壤有效厚度和蓄水容量从湿润的东江流域到湿润、半湿润淮河流域,以及半湿润、半干旱泾河流域存现增加趋势。采用修正后土壤有效厚度,3个流域模拟的水量平衡误差ER大幅降低,在1%以内。(2)从流域多年平均水碳通量变化来看,东江流域修正前后径流深R和实际蒸散发ET变化量最大,分别为50 mm、-49 mm;泾河流域变化量最小,分别为-4 mm、4 mm。对于修正前后的NPP,淮河流域变化量最大,东江流域最小,分别为17 gCm-2a-1和5 gCm-2a-1。从过程变化(变差系数Cv)来看,修正后的流域R、ET、NPP年际变化增大。
(3)从流域内各网格水碳通量空间变化来看,泾河流域修正后较修正前各网格多年平均R普遍减少,东江流域普遍增加,淮河流域北部网格以减少为主,南部普遍增加。修正前后各网格多年平均ET增减的空间分布与R增减的空间分布相反。对于NPP,泾河流域和淮河流域北部NPP增减变化明显,南部基本不变,东江流域南部以减少为主,北部以增加为主。
由于模型在网格之间并未考虑水量侧向交换及汇流路线,3个流域模拟结果中ENS提高并不明显,因此,在以后的研究中需要进一步研究汇流过程。
The authors have declared that no competing interests exist.
参考文献 原文顺序
文献年度倒序
文中引用次数倒序
被引期刊影响因子
[1] | . 从流域水文过程与植被相互作用 规律、流域生态水文模型、流域生态水文对气候变化的响应三个方面,对国内外相关研究现状进行了总结分析。从中发现,当前流域水文学正在从以单一水循环过程 为主要研究对象发展成以研究水分、能量与物质耦合循环以及水文过程与生态过程的相互作用等为主要内容的综合性、交叉性学科。现关于流域水文过程与植被之间 的关系研究多集中于单向作用的研究,如水文过程对植被的影响研究和植被变化对水文过程的影响研究,而对水文过程与植被之间的反馈机制以及对流域内土壤-植 被-大气复杂系统的整体研究不足,缺乏对流域水文过程与植被相互作用机理的全面认识。有研究表明,气候变化已经影响到流域水文过程和植被生长,气候变化下 的流域生态水文响应是水文学研究的前沿课题。水文学需要强化对流域生态水文机理的研究,只有在充分揭示流域生态水文机理的前提下,才能预测未来气候变化下 的流域生态水文响应。生态最优性原理能够解释植被对外界环境变化的响应,从而被应用于描述植被与水文过程的相互作用。基于生态最优性原理的流域生态水文模 型已逐渐成为生态水文学的研究热点之一。 . 从流域水文过程与植被相互作用 规律、流域生态水文模型、流域生态水文对气候变化的响应三个方面,对国内外相关研究现状进行了总结分析。从中发现,当前流域水文学正在从以单一水循环过程 为主要研究对象发展成以研究水分、能量与物质耦合循环以及水文过程与生态过程的相互作用等为主要内容的综合性、交叉性学科。现关于流域水文过程与植被之间 的关系研究多集中于单向作用的研究,如水文过程对植被的影响研究和植被变化对水文过程的影响研究,而对水文过程与植被之间的反馈机制以及对流域内土壤-植 被-大气复杂系统的整体研究不足,缺乏对流域水文过程与植被相互作用机理的全面认识。有研究表明,气候变化已经影响到流域水文过程和植被生长,气候变化下 的流域生态水文响应是水文学研究的前沿课题。水文学需要强化对流域生态水文机理的研究,只有在充分揭示流域生态水文机理的前提下,才能预测未来气候变化下 的流域生态水文响应。生态最优性原理能够解释植被对外界环境变化的响应,从而被应用于描述植被与水文过程的相互作用。基于生态最优性原理的流域生态水文模 型已逐渐成为生态水文学的研究热点之一。 |
[2] | |
[3] | 基于1981-2010 年青藏高原80 个气象台站观测数据, 通过改进的LPJ 动态植被模型, 模拟并分析了青藏高原实际蒸散及其与降水的平衡关系(<em>P-E</em>) 的时空变化。研究结果表明, 在过去三十年来青藏高原气候呈现以变暖为主要特征的背景下, 降水量整体略有增加, 潜在蒸散呈减少趋势, 特别是2000 年以前减少趋势显著;青藏高原大部分地区实际蒸散呈增加趋势, <em>P-E</em>的变化趋势呈西北增加—东南减少的空间格局。大气水分蒸散发能力降低理论上会导致实际蒸散减少, 而青藏高原大部分地区实际蒸散增加, 主要影响因素是降水增加, 实际蒸散呈增加(减少) 趋势的区域中86% (73%) 的降水增加(减少)。 . 基于1981-2010 年青藏高原80 个气象台站观测数据, 通过改进的LPJ 动态植被模型, 模拟并分析了青藏高原实际蒸散及其与降水的平衡关系(<em>P-E</em>) 的时空变化。研究结果表明, 在过去三十年来青藏高原气候呈现以变暖为主要特征的背景下, 降水量整体略有增加, 潜在蒸散呈减少趋势, 特别是2000 年以前减少趋势显著;青藏高原大部分地区实际蒸散呈增加趋势, <em>P-E</em>的变化趋势呈西北增加—东南减少的空间格局。大气水分蒸散发能力降低理论上会导致实际蒸散减少, 而青藏高原大部分地区实际蒸散增加, 主要影响因素是降水增加, 实际蒸散呈增加(减少) 趋势的区域中86% (73%) 的降水增加(减少)。 |
[4] | <p>Lund-Potsdam-Jena Dynamic Global Vegetation Model (LPJ)模型是由Lund University, Potsdam Climate Research Centre和Max-Planck-Institute for Biogeochemistry, Jena联合开发的植被动态模型,该模型以气候、土壤质地和CO<sub>2</sub>数据作为输入模拟植被与环境的碳水交换过程。为了利用高时间分辨率的输入数据,对模型的代码进行了改写。张掖绿洲盈科站位于干旱区绿洲典型的农田生态系统。应用其观测资料作为LPJ的输入,模拟了张掖绿洲制种玉米的碳水通量,并用涡动观测到的潜热和CO<sub>2</sub>通量验证蒸散发和NEE的模拟结果。结果表明LPJ模型能够较好地模拟制种玉米与环境之间的碳水交换,蒸散发(ET)的模拟值与观测值的R<sup>2</sup>为0.8;NEE的模拟值与观测值的R<sup>2</sup>为0.79。<br /> </p> . <p>Lund-Potsdam-Jena Dynamic Global Vegetation Model (LPJ)模型是由Lund University, Potsdam Climate Research Centre和Max-Planck-Institute for Biogeochemistry, Jena联合开发的植被动态模型,该模型以气候、土壤质地和CO<sub>2</sub>数据作为输入模拟植被与环境的碳水交换过程。为了利用高时间分辨率的输入数据,对模型的代码进行了改写。张掖绿洲盈科站位于干旱区绿洲典型的农田生态系统。应用其观测资料作为LPJ的输入,模拟了张掖绿洲制种玉米的碳水通量,并用涡动观测到的潜热和CO<sub>2</sub>通量验证蒸散发和NEE的模拟结果。结果表明LPJ模型能够较好地模拟制种玉米与环境之间的碳水交换,蒸散发(ET)的模拟值与观测值的R<sup>2</sup>为0.8;NEE的模拟值与观测值的R<sup>2</sup>为0.79。<br /> </p> |
[5] | Earth's vegetation plays a pivotal role in the global water balance. Hence, there is a need to model dynamic interactions and feedbacks between the terrestrial biosphere and the water cycle. Here, the hydrological performance of the Lund–Potsdam–Jena model (LPJ), a prominent dynamic global vegetation model, is evaluated. Models of this type simulate the coupled terrestrial carbon and water cycle, thus they are well suited for investigating biosphere–hydrosphere interactions over large domains. We demonstrate that runoff and evapotranspiration computed by LPJ agree well with respective results from state-of-the-art global hydrological models, while in some regions, runoff is significantly over- or underestimated compared to observations. The direction and magnitude of these biases is largely similar to those from other macro-scale models, rather than specific to LPJ. They are attributable primarily to uncertainties in the climate input data, and to human interventions not considered by the model (e.g. water withdrawal, land cover conversions). Additional model development is required to perform integrated assessments of water exchanges among the biosphere, the hydrosphere, and the anthroposphere. Yet, the LPJ model can now be used to study inter-relations between the world's major vegetation types and the terrestrial water balance. As an example, it is shown that a doubling of atmospheric COcontent alone would result in pronounced changes in evapotranspiration and runoff for many parts of the world. Although significant, these changes would remain unseen by stand-alone hydrological models, thereby emphasizing the importance of simulating the coupled carbon and water cycle. |
[6] | The LPJ dynamic global vegetation and hydrology model with river routing is implemented on a compute cluster in order to reduce the overall computation time. In order to achieve this, a parallel algorithm had to be developed for the river routing part of the LPJ code. It can be shown that the run time of the parallel LPJ model scales well with the number of parallel tasks, even getting a super-linear speedup for 8–128 tasks. The sequential part of the model code can be estimated to be only 0.16%. This offers the opportunity, for example, to apply the model to find optimal climate change mitigation/adaptation paths requiring a multitude of subsequent simulation runs. The algorithm can also be used for networks with a different topology than river routing networks. |
[7] | <p>Overall, the results show that the LPX-DGVM can successfully simulate runoff processes for small catchments in the UK. This study offers promising insights into the use of global-scale models and datasets for local-scale studies of water resources, with the eventual aim of providing local-scale projections of future water distributions. Copyright © 2013 John Wiley & Sons, Ltd.</p> |
[8] | ABSTRACT |
[9] | . . |
[10] | |
[11] | |
[12] | For the first time, a model that simulates methane emissions from northern peatlands is incorporated directly into a dynamic global vegetation model. The model, LPJ-WHyMe (LPJ-Wetland Hydrology and Methane), was previously modified in order to simulate peatland hydrology, permafrost dynamics and peatland vegetation. LPJ-WHyMe simulates methane emissions using a mechanistic approach, although the use of some empirical relationships and parameters is unavoidable. The model simulates methane production, three pathways of methane transport (diffusion, plant-mediated transport and ebullition) and methane oxidation. Two sensitivity tests were conducted, first to identify the most important factors influencing methane emissions and secondly to justify the choice of parameters. A comparison of model results to observations from seven sites revealed in general good agreement but also highlighted some problems. Circumpolar methane emissions for the period 1961鈥1990 were estimated to be between 40.8 and 73.7 Tg CH4 a-1. |
[13] | Abstract [1]69A long-standing challenge for hydrologists has been a lack of observational data on global-scale basin hydrological behavior. With observations from NASA's Gravity Recovery and Climate Experiment (GRACE) mission, hydrologists are now able to study terrestrial water storage for large river basins (>200,000 km 2 ), with monthly time resolution. Here we provide results of a time series model of basin-averaged GRACE terrestrial water storage anomaly and Global Precipitation Climatology Project precipitation for the world's largest basins. We address the short (10 year) length of the GRACE record by adopting a parametric spectral method to calculate frequency-domain transfer functions of storage response to precipitation forcing and then generalize these transfer functions based on large-scale basin characteristics, such as percent forest cover and basin temperature. Among the parameters tested, results show that temperature, soil water-holding capacity, and percent forest cover are important controls on relative storage variability, while basin area and mean terrain slope are less important. The derived empirical relationships were accurate (0.5465≤65 E f 65≤650.84) in modeling global-scale water storage anomaly time series for the study basins using only precipitation, average basin temperature, and two land-surface variables, offering the potential for synthesis of basin storage time series beyond the GRACE observational period. Such an approach could be applied toward gap filling between current and future GRACE missions and for predicting basin storage given predictions of future precipitation. |
[14] | |
[15] | . |
[16] | Plant-extractable water capacity of soil is the amount of water that can be extracted from the soil to fulfill evapotranspiration demands. It is often assumed to be spatially invariant in large-scale computations of the soil-water balance. Empirical evidence, however, suggests that this assumption is incorrect. In this paper, we estimate the global distribution of the plant-extractable water capacity of soil.A representative soil profile, characterized by horizon (layer) particle size data and thickness, was created for each soil unit mapped by FAO (Food and Agriculture Organization of the United Nations)/Unesco. Soil organic matter was estimated empirically from climate data. Plant rooting depths and ground coverages were obtained from a vegetation characteristic data set. At each 0.5 0.5 grid cell where vegetation is present, unit available water capacity (cm water per cm soil) was estimated from the sand, clay, and organic content of each profile horizon, and integrated over horizon thickness. Summation of the integrated values over the lesser of profile depth and root depth produced an estimate of the plant-extractable water capacity of soil.The global average of the estimated plant-extractable water capacities of soil is 86cm (Greenland, Antarctica and bare soil areas excluded). Estimates are less than 5, 10 and 15 cm - over approximately 30, 60, and 89 per cent of the area, respectively. Estimates reflect the combined effects of soil texture, soil organic content, and plant root depth or profile depth. The most influential and uncertain parameter is the depth over which the plant- extractable water capacity of soil is computed, which is usually limited by root depth. Soil texture exerts a lesser, but still substantial, influence. Organic content, except where concentrations are very high, has relatively little effect. |
[17] | |
[18] | The sensitivity of the global water cycle to the water-holding capacity of the plant-root zone of continental soils is estimated by simulations using a mathematical model of the general circulation of the atmosphere, with prescribed ocean surface temperatures and prescribed cloud. With an increase of the globally constant storage capacity, evaporation from the continents rises and runoff falls, because a high storage capacity enhances the ability of the soil to store water from periods of excess for later evaporation during periods of shortage. In addition, atmospheric feedbacks associated with higher precipitation and lower potential evaporation drive further changes in evaporation and runoff. Most changes in evaporation and runoff occur in the tropics and the northern middle-latitude rain belts. Global evaporation from land increases by 7 cm for each doubling of storage capacity. Sensitivity is negligible for capacity above 60 cm. In the tropics and in the extratropics,increased continental evaporation is split between increased continental precipitation and decreased convergence of atmospheric water vapor from ocean to land. In the tropics, this partitioning is strongly affected by induced circulation changes, which are themselves forced by changes in latent heating. In the northern middle and high latitudes, the increased continental evaporation moistens the more» atmosphere. This change in humidity of the atmosphere is greater above the continents than above the oceans, and the resulting reduction in the sea-land humidity gradient causes a decreased onshore transport of water vapor by transient eddies. Results here may have implications for problems in global hydrology and climate dynamics, including effects of water resource development on global precipitation, climatic control of plant rooting characteristics, climatic effects of tropical deforestation, and climate-model errors. 21 refs., 13 figs., 21 tabs. 芦less |
[19] | ABSTRACT This study uses a well-established water balance methodology, the Thornthwaite-Mather approach, to evaluate the effects of soil water holding capacity assumptions on estimates of African evapotranspiration rates, moisture deficit, and moisture surplus conditions. Under constant climate conditions, the model tests the impact of using a constant 150 mm soil water holding capacity compared to using a newly derived soil water holding capacity data set (the Dunne-Willmott data set). The study also uses a worldwide survey of soil degradation between 1950 and 1980 (GLASOD: Global Assessment of Soil Degradation) to evaluate the impacts of human-induced soil degradation on local water balances. The GLASOD data are used to alter local soil water holding capacities based on the Dunne-Willmott data to simulate human soil degradation patterns in Africa. Results indicate that the use of simplified soil water holding capacities can lead to significant errors in estimated evapotranspiration rates and water surplus and deficit conditions in Africa. Regions most affected are those with seasonal wet and dry climates, which are also those locations with the greatest climate variability. Because these are often the climates most studied to detect and model environmental change, it is important that accurate soil moisture estimates be used to simulate climate conditions in these regions. Results also indicate that soil degradation occurring over a 30 yr period (1950 to 1980) has had a significant impact on local water resources. The greatest impacts of these changes are in some of the more productive agricultural areas in the wetter sub-humid climates. Changes include increased runoff during wet seasons and an extended drought period during the dry seasons. Given that these agricultural systems have less flexibility to respond to long-term desiccation as compared to pastoral systems, this could lead to significant changes in local growing seasons and perhaps overall productivity in the future. |
[20] | |
[21] | 植被退化是全球环境变化研究的一个重点问题,植被状况是环境评估的重要指标。利用8km分辨率的NOAA-AVHRR/NDVI时间序列数据,对位于黄土高原的泾河流域1982~2003年植被特征及变化状况进行系统分析,并在此基础上评估降雨与流域植被的相互关系。研究主要利用了变化斜率法、主成分分析法和相关分析法,得到如下结论:过去22a来流域植被NDVI均值波幅和变化都很小,变化较显著的区域集中在流域上游和流域边缘的山区。变化斜率分析得出了类似的结论,气候变化以及人类活动导致的土地利用改变可能是影响在流域不同地区植被状态变化的主要原因。对NDVI时间序列的主成分分析发现PC1和PC2与植被覆盖和气候密切联系,PC3和PC4与流域汛期洪水有关,PC5和PC6体现了人类活动的影响。流域的NDVI与降雨显示了良好的相关性,降雨与NDVI相关性的阈值可能在550mm或更高。 . 植被退化是全球环境变化研究的一个重点问题,植被状况是环境评估的重要指标。利用8km分辨率的NOAA-AVHRR/NDVI时间序列数据,对位于黄土高原的泾河流域1982~2003年植被特征及变化状况进行系统分析,并在此基础上评估降雨与流域植被的相互关系。研究主要利用了变化斜率法、主成分分析法和相关分析法,得到如下结论:过去22a来流域植被NDVI均值波幅和变化都很小,变化较显著的区域集中在流域上游和流域边缘的山区。变化斜率分析得出了类似的结论,气候变化以及人类活动导致的土地利用改变可能是影响在流域不同地区植被状态变化的主要原因。对NDVI时间序列的主成分分析发现PC1和PC2与植被覆盖和气候密切联系,PC3和PC4与流域汛期洪水有关,PC5和PC6体现了人类活动的影响。流域的NDVI与降雨显示了良好的相关性,降雨与NDVI相关性的阈值可能在550mm或更高。 |
[22] | [D]. [D]. |
[23] | |
[24] | |
[25] | |
[26] | ?基于分布式水文模型SWAT以及SUFI-2程序对东江流域进行了51a(1960—2010年)月径流模拟,并在此基础上讨论了东江流域水文过程的空间差异。模拟结果表明,河源站受新丰江水库影响,模拟结果不确定性较大,确定性系数R2和Nash-Sutcliffe效率系数相对其他站点较低,龙川站、岭下站、博罗站R2和Nash-Sutcliffe效率系数皆满足模型适用性要求。流域出口博罗站率定期R2、Nash-Sutcliffe效率系数分别为0.83、0.83,验证期为0.84、0.84,模型模拟精度较高。东江流域径流深主要受降水空间不均影响,形成由北向南先增后减的趋势。流域下垫面差异对产流过程也有一定影响。其中土壤含水量受土壤性质、人类活动等影响,由北向南差异明显;蒸散发量受植被覆盖影响亦较为明显,北部蒸散量占降雨量的比例大于中部及南部。 . ?基于分布式水文模型SWAT以及SUFI-2程序对东江流域进行了51a(1960—2010年)月径流模拟,并在此基础上讨论了东江流域水文过程的空间差异。模拟结果表明,河源站受新丰江水库影响,模拟结果不确定性较大,确定性系数R2和Nash-Sutcliffe效率系数相对其他站点较低,龙川站、岭下站、博罗站R2和Nash-Sutcliffe效率系数皆满足模型适用性要求。流域出口博罗站率定期R2、Nash-Sutcliffe效率系数分别为0.83、0.83,验证期为0.84、0.84,模型模拟精度较高。东江流域径流深主要受降水空间不均影响,形成由北向南先增后减的趋势。流域下垫面差异对产流过程也有一定影响。其中土壤含水量受土壤性质、人类活动等影响,由北向南差异明显;蒸散发量受植被覆盖影响亦较为明显,北部蒸散量占降雨量的比例大于中部及南部。 |