
Impacts of apple tree planting on deep soil water content and groundwater recharge for the Loess Tableland of China
CHENG Liping
通讯作者:
收稿日期:2020-09-21接受日期:2020-12-18
基金资助: |
Received:2020-09-21Accepted:2020-12-18

摘要
关键词:
Abstract
Keywords:
PDF (3106KB)元数据多维度评价相关文章导出EndNote|Ris|Bibtex收藏本文
本文引用格式
程立平, 王亚萍, 齐光. 黄土塬区苹果种植对深剖面土壤水分和地下水补给的影响[J]. 地理研究, 2021, 40(9): 2684-2694 doi:10.11821/dlyj020200908
CHENG Liping, WANG Yaping, QI Guang.
1 引言
借助得天独厚的地理环境条件,渭北黄土高塬沟壑区成为中国乃至世界上苹果最优产区之一。自从20世纪80年代以来,在政策引导和经济利益双重驱动下,渭北黄土旱塬区苹果种植面积大幅增加,成为商品化苹果生产基地。然而由于黄土高原地处蒸发力大于降水量的半湿润和半干旱气候区,苹果生育所需水量超过年降水量,需水缺额要通过吸收深层土壤储水补充[1],从而使深层土壤储水重新参与了植物-大气的水循环之中,导致深层土壤发生干燥化以至形成干层[2,3,4,5],甚至影响地下水的补给过程[6,7,8],引起****们的关注。但是由于采样深度和样地数量的限制,苹果林地深剖面土壤水分随林龄变化过程以及不同林龄苹果林地对地下水补给的影响依然不清,因此本文通过在不同年份测定数十块苹果林地0~20 m深剖面土壤湿度以及土壤水氯离子浓度,研究不同林龄苹果林地厚深黄土剖面土壤水分变化以及其对地下水补给的定量影响,以期进一步明确大面积农田转换为果园对区域水循环的影响,为黄土塬区农果面积优化以及地下水资源的可持续利用提供一定的理论参考。2 研究区概况与研究方法
2.1 研究区概况
研究区地处黄土高原南部,陕西省长武县境内,中国科学院长武农业生态试验站所在的黄土塬面上,是典型的黄土塬,平均海拔1220 m。该区属暖温带半湿润易旱大陆性季风气候,年均降水量580 mm,降水年内分布不均、年际变化较大,7—9月降水量占年降水量55%以上。长武塬区黄土堆积深厚,土壤为黑垆土,母质为中壤质马兰黄土,地下水埋深40~80 m。深厚的黄土剖面由黄土-古土壤序列组合而成,由于强烈的侵蚀作用,在黄土塬边缘沟坡地带,存在有自然出露的古土壤层,利用环刀法测定黄土和古土壤层土壤容重分别为1.31 g/cm3和1.50 g/cm3,田间持水量分别为21.21%和24.68%(表1)。Tab. 1
表1
表1黄土剖面田间持水量和容重
Tab. 1
土层名称 | 田间持水量(g/g,%) | 容重(g/cm3) | |||||||
---|---|---|---|---|---|---|---|---|---|
最大值 | 最小值 | 平均值 | 标准差 | 最大值 | 最小值 | 平均值 | 标准差 | ||
古土壤层 | 27.09 | 22.25 | 24.68 | 1.63 | 1.58 | 1.41 | 1.50 | 0.04 | |
黄土层 | 22.01 | 20.43 | 21.21 | 0.46 | 1.37 | 1.27 | 1.31 | 0.03 |
新窗口打开|下载CSV
2.2 土壤样品采集与测定
不同年份于长武站所在的王东村塬面上选择不同林龄苹果林地采集土壤样品。2009年10月选择18龄果园,2012年7月选择13龄、15龄、20龄-1和20龄-2四块样地,2018年8月选择0龄(对照农田)、5龄、10龄、20龄和27龄五块样地,其中2009年选择的18龄和2018年选择的27龄苹果林地为同一样地。利用土钻法采集土壤样品,取样深度20 m,每间隔20 cm采集一个土壤样品,所有土样利用烘干法测定土壤质量含水量,土壤体积含水量通过质量含水量乘以土壤容重获得。利用离子色谱仪测定2018年农田、10龄和27龄苹果林地下土壤水氯离子含量,0~6 m间隔20 cm测定一次,6~20 m间隔50 cm测定一次。具体方法为:土壤风干研磨过0.5 mm筛、烘干,取烘干样3 g加入3.6 mmol/L Na2CO3 和4.5 mmol/L NaHCO3溶液30 mL后:① 摇床震荡2小时;② 超声震荡1小时;③ 静置15分钟后离心分离;④ 取上清液过0.45μm针头过滤器;⑤ 离子色谱仪测定过滤液。
2.3 土壤储水计算
土壤储水量计算公式为:式中:W为土壤储水量(mm);
2.4 土壤储水量消耗速率计算
土壤储水量消耗速率计算公式为:式中:Cr为土壤储水量消耗速率(mm/a);T为苹果林地林龄(a);WT1、WT2分别为林龄为T1和T2苹果林地土壤储水量(mm)。
2.5 地下水补给计算
在干旱半干旱地区,氯离子平衡法[9]是一种广泛应用的地下水补给量计算方法,其在黄土高原地区也有很好的适用性[6,7,10-14]。地下水多年平均潜在补给量计算公式为:式中:R为地下水多年平均潜在补给量(mm/a);P为多年平均降水量(mm);Cp为降水(包括干沉降)氯离子平均浓度(mg/L);Cs为植物根系层以下土壤水氯离子平均浓度(mg/L)。长期监测氯离子沉降数据对于利用氯离子平衡法估算地下水补给量至关重要,由于研究区没有氯离子沉降的长期监测数据,本研究利用了东亚酸沉降监测网络西安鸡窝子站(研究区东南约100 km处)的监测数据(https://www.eanet.asia/)。2000—2018年鸡窝子站降水中氯离子体积加权平均浓度为1 mg/L,而氯离子干沉降约占总沉降的10%~33%[12],本文假定干沉降比例为20%,所以Cp为1.2 mg/L。
地下水下渗补给速度为:
式中:V为地下水下渗补给速度(mm/a);R为地下水多年平均潜在补给量(mm/a);
相较于农作物,苹果树具有极深的根系,因此农田转换为苹果林地后苹果根区与根区以下土壤氯离子平衡的建立是一个极其漫长的过程,而在这种平衡建立之前氯离子平衡法并不适用于估算地下水补给量[15]。为了能够计算苹果林地下地下水补给量,Zhang等[7]基于水量平衡和氯离子平衡建立了新的估算公式:
式中:Rd为苹果林地下地下水平均潜在补给量(mm/a);Rs是农田下地下水平均潜在补给量(mm/a);ΔS是苹果林地相对于农田土壤水分亏缺量(mm);T是苹果林地林龄(a)。
2.6 数据处理与制图
采用Excel 2010软件进行数据处理,利用Origin 2018软件制图。3 结果分析
3.1 不同地块相同年限苹果林地深剖面土壤水分差异
深厚的黄土剖面由黄土-古土壤序列组合而成,与黄土层相比,古土壤层黏重、持水性能较高,但是同层黄土层(或古土壤层)则质地均匀[16],因此黄土剖面物理性质的水平变化远小于其垂直变化[14]。受降水入渗和蒸散发影响,黄土剖面上层土壤处于水分消耗、补充的交替过程,含水率波动明显,形成干湿交替层[17]。不同时间不同地块的20龄苹果林地土壤湿度在4 m以上土层差异明显,而在4 m以下深层则几乎没有差异(图1),因此0~4 m土层可定义为干湿交替层的厚度范围,与已有研究结果一致[3]。干湿交替层以下深层剖面土壤水分含量主要受土地利用方式影响[3],三块20龄苹果林地4~20 m剖面平均水分含量分别为14.96%、15.11%和14.70%,方差分析表明含水率无显著差异(p=0.46)。可见,对于相同年限苹果林地而言,尽管不同时间不同地块样地4 m以下土层土壤含水率有所差异,但是并无显著性差异。基于此,本文主要分析不同林龄苹果林地4 m以下深层土壤水分的变化。图1
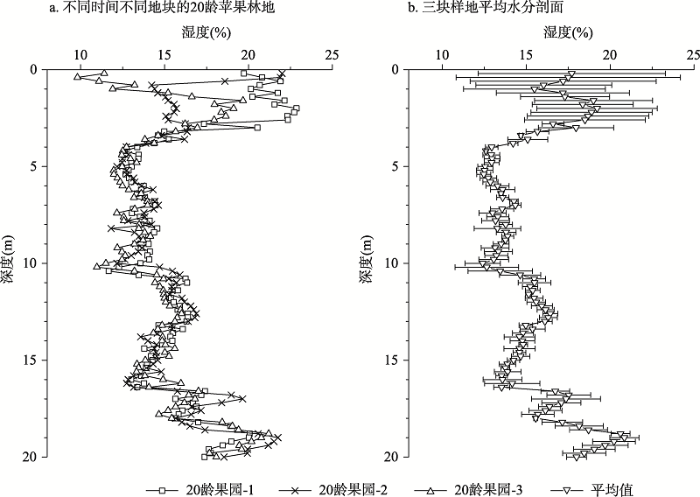
图120龄苹果林地土壤水分剖面
注:图b中误差线为标准差(n=3)。
Fig. 1Soil water profiles beneath 20-yr apple orchards
3.2 苹果林地深剖面土壤水分消耗过程
由于采样时间不同,不同林龄苹果林地干湿交替层内(0~4 m)土壤水分曲线随林龄增加没有表现出规律性变化,但是4 m以下深层土壤水分曲线表现出随林龄增加由浅及深向左偏移(图2)。这是因为在半湿润半干旱的黄土地区,苹果林地蒸散需水量超过年降水量,需要通过吸收深层土壤储水来补充蒸散需水缺额[3]。由于幼龄苹果林地耗水较小,5龄苹果林地4~7 m土层范围土壤水分较农田有所恢复,但是10、13、15、18和20龄苹果林地土壤水分利用深度分别已达7、11、13、16和20 m,27龄苹果林地则已经利用了20 m深度以下土壤水分(图2),从而使深层土壤水分含量由浅及深逐年降低。5、10、13、15、18、20和27龄苹果林地4~20 m剖面平均土壤水分含量分别为20.84%、20.31%、19.23%、17.88%、15.54%、14.93%和13.38%,土壤水分曲线随林龄增加向左偏移。图2
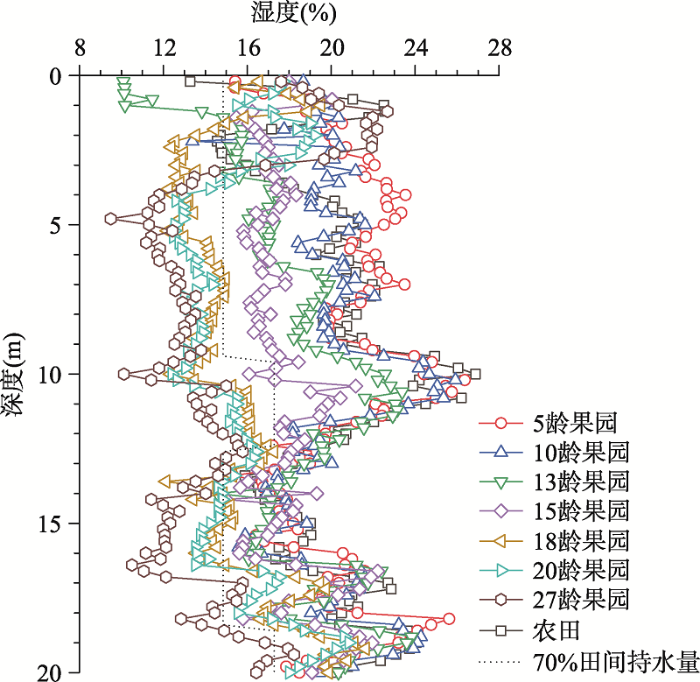
图2不同林龄苹果林地土壤水分剖面
Fig. 2Soil water profiles beneath apple orchards with different stand ages
在黄土高原地区,由于潜在蒸发大于降水量,加之地下水深埋,浅层土壤经常处于干燥化状态,加上高耗水人工林草的参与,使土壤干燥化向深层土层发展,最终将在土体某一深度范围内形成厚度不等的低湿层-土壤干层[1,5]。土壤干层内土壤含水量介于萎蔫湿度和田间稳定湿度之间,研究区土壤稳定湿度为田间持水量的70%[5]。从图2看出,苹果林地在15至18龄之间形成土壤干层,18龄苹果林地土壤干层已下延至12m深度。随着果树林龄增加,土壤干层继续向下延伸,干燥化程度提高,27龄苹果林地土壤干层下限深度已达19 m。
农田转换为苹果林地后土壤储水量逐年减少,水分亏缺量(相对于农田)逐年增加,27龄苹果林地深层(4~20 m)土壤储水量为2921 mm,亏缺量为1623 mm(图3)。但是苹果林地深层土壤储水量随林龄增加并非直线减少,而是呈现倒“S”型曲线下降,其下降过程可用Logistics模型较好的拟合(R2=0.99)。原因在于幼龄期苹果树耗水量较小甚至小于农作物,因此农田转换为果园的前10 a为深层土壤储水量的缓慢减少期;10~15 a为深层土壤储水量加速消耗期,15~18 a深层土壤储水量消耗速率达到最大(183 mm/a),18~27 a为消耗速率减速期,27 a后苹果林地下已然形成深厚的土壤干层[1],加之果树趋于老龄化,耗水量降低,深层土壤储水量进入稳定期(图3、表2)。
图3
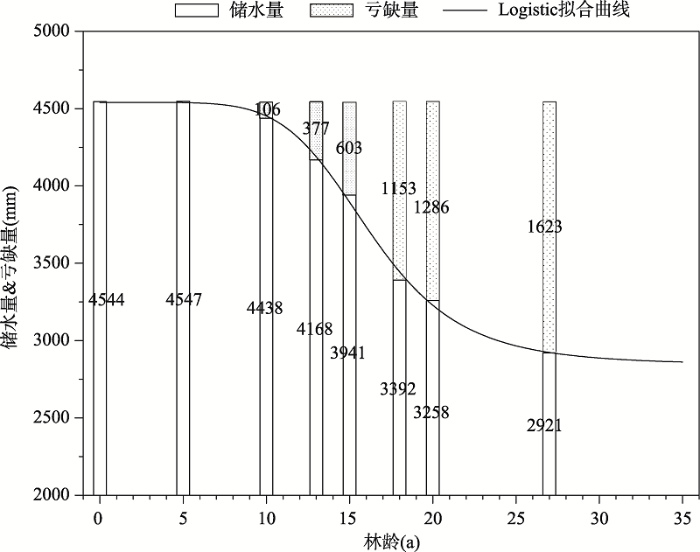
图3不同林龄苹果林地深层(4~20 m)土壤储水量和亏缺量(相对于农田)
Fig. 3Soil water storage and soil water deficit (relative to farmland) in deep soil layers (4-20 m) beneath apple orchards with different stand ages
Tab. 2
表2
表2苹果林地深层(4~20 m)土壤储水量消耗速率
Tab. 2
林龄段(a) | 0~5 | 5~10 | 10~13 | 13~15 | 15~18 | 18~20 | 20~27 |
---|---|---|---|---|---|---|---|
Cr(mm/a) | 0 | 22 | 90 | 113 | 183 | 67 | 48 |
新窗口打开|下载CSV
3.3 黄土剖面土壤水氯离子浓度变化
在半干旱半湿润地区,受土壤水分蒸散发的影响,浅层土层普遍存在氯离子累积现象[18,19]。图4a给出了不同地块土壤水氯离子浓度剖面变化,可以看出土壤水氯离子在浅层随深度波动变化明显,而在深层则相对稳定,但是不同地块波动变化的深度范围有所差异。农田0~4 m氯离子介于(23.4~1171.4)mg/L之间,4 m以下则介于(15.0~36.7)mg/L之间;10龄苹果林地0~4 m氯离子介于(24.2~485.4)mg/L之间,4 m以下则介于(15.4~50.4)mg/L之间;27龄苹果林地0~10 m氯离子介于(29.4~408.0)mg/L之间,10 m以下则介于(19.3~37.3)mg/L之间。农田转换为苹果林地后(浅根作物转换为深根林木)所导致的蒸散量增加,不仅使深层土壤储水量发生减少,而且使深层氯离子产生富集,农田、10龄和27龄苹果林地3~10 m氯离子平均含量分别为30.8 mg/L、36.6 mg/L和114.3 mg/L。可见,由于幼龄苹果林地耗水量较小且对深层土壤水分影响有限(图3),因此对深层土壤氯离子含量富集作用并不明显,但是随着林龄的增加,苹果林地对深层土壤氯离子的富集作用越来越突出(图4a)。土壤剖面中氯离子含量的累积不仅与蒸散发有关,同时受施用化肥的影响。文献表明,研究区含氯化肥的施用记录可以追溯到20世纪80年代[14],尤其在苹果林地的广泛施用,但是不同地块施用化肥时间不同,导致影响深度不同。图4a可见,农田、10龄苹果林地土壤水氯离子含量最大值出现在2.2 m深度处,而27龄苹果林地则出现在4.8 m深度处,表明27龄苹果林地含氯化肥施用时间应早于农田和10龄苹果林地。图4
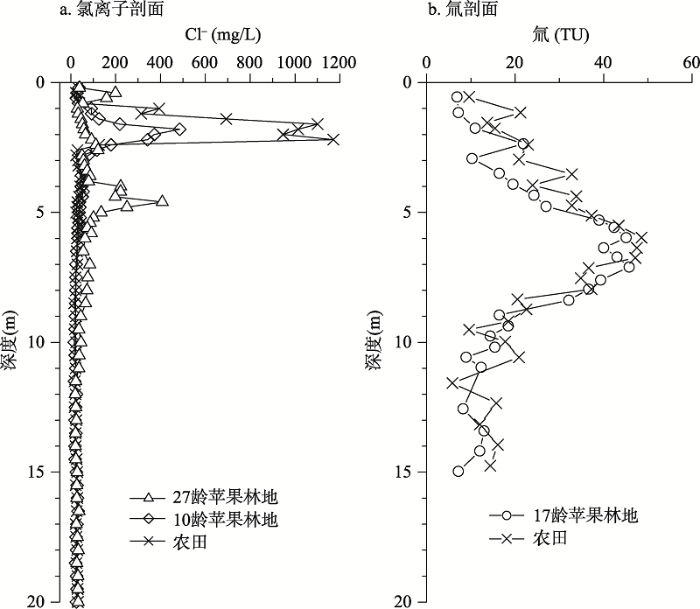
图4黄土深剖面氯离子和氚分布
注:图b数据来源于同一区域研究文献[20]。
Fig. 4Distributions of chloride and tritium for deep loess profiles
3.4 地下水补给方式与补给量
由于独特的水文地质条件,降水是黄土塬区地下水唯一补给来源,但是在很长一段时间内关于降水是以何种方式(活塞流/优先流)通过深厚黄土包气带补给地下水存在一定的争议[8,21-23]。随着技术手段的进步,黄土包气带中存在的完整氚峰剖面[14,20,24,25]以及氯离子、硝态氮等剖面分布规律[6,7,12,14]均表明活塞流是黄土塬区土壤水分深层渗漏以及地下水补给的主导方式(图4b)。尤其是在农田、果园等土地利用方式下,活塞流甚至是地下水补给的唯一方式。为了排除含氯化肥的施用对氯离子平衡方法估算地下水补给量的影响,本文选取7 m以下土层土壤水氯离子平均值来计算农田条件下降水对地下水的潜在补给量。农田下7~20 m土层土壤水氯离子平均浓度为23.1 mg/L,利用公式(3)计算得出地下水多年平均补给量为30.2 mm/a,占多年平均降水量的5.2%。农田7~20 m土层平均体积含水率为28.7%,则土壤水多年平均下渗速度为105 mm/a。
研究表明26龄苹果根系深达18 m [2],虽然农田转换为苹果林地27 a后10 m以上土层土壤氯离子已明显富集,但是其根际深度和根际深度以下土壤氯离子尚未建立平衡,因此氯离子平衡法并不适用于估算苹果林地地下水的补给量[15],所以本文利用公式(5)来计算(表3)。由于幼龄苹果林地水分消耗小,0~5龄苹果林地并未对地下水补给造成影响,5龄以后苹果林地将逐渐减少地下水补给量,直至阻断地下水的补给。其原因在于随着林龄增加苹果耗水增加,导致深层土壤水分亏缺逐年升高,土壤干层形成,进而阻断了降水对地下水的补给。
Tab. 3
表3
表3不同林龄下苹果林地地下水补给量
Tab. 3
林龄(a) | 0(农田) | 5 | 10 | 13 | 15 | 18 | 20 | 27 |
---|---|---|---|---|---|---|---|---|
△S/T | 0.0 | 0.0 | 10.6 | 29.0 | 40.2 | 64.0 | 64.3 | 60.1 |
Rd | 30.2 | 30.2 | 19.6 | 1.2 | 0.0 | 0.0 | 0.0 | 0.0 |
新窗口打开|下载CSV
4 讨论与结论
4.1 讨论
在半湿润半干旱的黄土高原地区,由于潜在蒸发大于降水量,苹果林地蒸散量超过年降水量,需水缺额通过吸收深层土层储水来补充[1],使本该向下运移补给地下水参与水文大循环的深层土壤水重新参与了土壤-植物-大气的水分小循环。黄土塬区降水只能补给到黄土剖面上部的干湿交替层[3],而干湿交替层以下的深层土壤水因得不到降水补给由浅及深逐年减少。本研究表明,苹果林地深层土壤储水随林龄增加呈倒“S”曲线趋势下降,可用Logistics模型较好模拟(R2=0.99),与已有相关研究结果不同;相关研究指出,随苹果林龄增加,苹果林地下土壤储水呈直线趋势下降[2, 6]。造成这种差异的原因可能有以下几点:① 已有研究选择样地较少,如Huang等[6]选择了15、24和30龄三个林龄苹果林地,Wang等[2]选择了5、9、17、22和26龄五个林龄苹果样地,本研究选择了10块不同林龄苹果林地。② 黄土剖面取样深度不同,Huang等取样深度为15 m,Wang等取样深度为18 m,本研究取样深度为20 m。③ 本研究将0龄苹果林地(农田)作为起点进行拟合,而已有研究则是将所选择最小林龄苹果林地作为起点进行拟合。随林龄增加,苹果利用土壤水分深度逐年增加,10、13、15、18和20龄苹果已经分别利用了7、11、13、16和20 m深度土壤水分,这与苹果根系向深层生长规律基本一致[2,26]。由于采样深度限制,本文没有明确27龄苹果土壤水分利用的具体深度,但是Li等观测发现22龄苹果树水分利用深度已达24.8 m[26],因此可以推测27龄苹果林地土壤水分利用深度至少在24.8 m深度以下。黄土塬区降水是地下水的唯一补给来源以及活塞流是地下水补给的主导方式已得到广泛的认可[6,14,24,27]。本研究利用氯离子平衡法计算得出,长武黄土塬区农田条件下地下水多年平均潜在补给量为30.2 mm/a,占年均降水量的5.2%,与同一研究区利用氚峰示踪法[20]以及氯离子平衡法[14]估算结果基本一致。黄土区小麦根系分布深度一般在3 m之内,降水只有通过农作物根系层形成深层渗漏,才有可能继续向下运移并对地下水形成补给。Liu等利用P-Ⅲ型统计曲线对长武旱作高产农田降水最大入渗深度进行分析,表明降水入渗至3 m深度的重现期为10 a[28]。说明虽然农田条件下地下水多年平均潜在补给量为30.2 mm,但是并不意味降水每年都能通过作物根系层形成深层渗漏,而只有在丰水年份降水才能形成深层渗漏。例如在特大丰水年2003年,长武农田降水入渗深度达5 m[28],利用本研究中农田3~5 m土层平均湿度19.2%计算,仅2003年农田条件下深层渗漏量高达500 mm,相当于16.6年(500/30.2)的潜在补给量之和。正是因为如此,黄土剖面存在的1963-氚峰极有可能只是被1963年以来的数个丰水年的降水以活塞流的形式下推至目前的位置,这也是农田和17龄苹果林地(1998年种植)氚峰所在位置并无明显差异的原因(图4b)。
虽然农田转换为苹果林地最初几年并未对地下水补给造成影响,但是随着苹果林龄的增加,苹果林地下形成的深厚土壤干层终将阻断降水对地下水的补给,使地下水潜在补给量降低为0,Huang等[6]和Li等[20]的研究也得到了类似的结果。黄土塬区是苹果优生区,政府政策引导和较高的经济收益提高了农民的种植积极性,苹果种植面积自20世纪80年代开始大幅增加。调查发现长武塬区潜水水位自90年代初期开始快速下降(年均下降0.3 m),与苹果大面积种植在时间上具有很好的吻合性和相关性[29],同样也表明了苹果种植对地下水的补给具有重要影响。随着苹果种植面积的继续扩大,农耕地面积将进一步减少[29],在地下水补给中占据主导地位的均匀活塞流将逐步减弱[6,7],导致黄土塬区地下水位将持续下降,进而影响地下水资源的可持续利用。
4.2 结论
(1)苹果林地土壤水分利用深度随林龄增加逐年向下延伸,27龄苹果林地可利用20 m深度以下土壤水分,导致深剖面土壤水分含量随林龄增加由浅及深逐年降低。5、10、13、15、18、20和27龄苹果林地4~20 m剖面平均水分含量分别为20.84%、20.31%、19.23%、17.88%、15.54%、14.93%和13.38%。苹果林地下4~20 m土层土壤储水量随林龄增加呈倒“S”曲线趋势下降,具体表现为0~10龄为缓慢减少期,10~15龄为加速减少期,15~18龄减少速率达到最大的183 mm/a,18~27龄为减速减少期,之后深层土壤储水量进入稳定期。(2)丰水年份形成的活塞流是深层渗漏以及地下水补给的主导方式,氯离子平衡法计算得出农田条件下地下水多年平均潜在补给量为30.2 mm,占多年平均降水量的5.2%。农田转换为苹果林地后形成的深厚土壤干层将减弱黄土塬区地下水补给过程中活塞流的主导作用。
黄土塬区苹果大面积种植对厚深黄土剖面土壤水资源和地下水补给造成了深刻影响,需通过政策引导协调农果面积比例,通过生产技术改造保持适度生产力并提升苹果品质,保证地下水资源持续补给,达到可持续利用的目的。
致谢:
真诚感谢二位匿名评审专家在论文评审中所付出的时间和精力,评审专家对论文结构、结果与分析方面,特别是对于不同地块相同林龄苹果林地深剖面水分比较和严谨性方面的修改意见,使本文获益匪浅。参考文献 原文顺序
文献年度倒序
文中引用次数倒序
被引期刊影响因子
[本文引用: 4]
[本文引用: 4]
URL [本文引用: 5]
[本文引用: 5]
[本文引用: 5]
[本文引用: 1]
[本文引用: 3]
[本文引用: 3]
[本文引用: 8]

Interpretation of groundwater recharge mechanisms is problematic because of the muted instantaneous response of subsurface water to rainfall and limited recharge rates, particularly in semi-arid environments with deep loess deposits. Here we identify the possible groundwater recharge mechanisms in 200-m thick loess deposits with unsaturated zone thickness of over 40 m. We collected soil samples up to 15 m deep under four land use types (one grassland and three apple orchards with stand ages 15, 24 and 30 years old), and used three-year precipitation and groundwater samples to determine the contents of stable water isotopes, chloride, and tritium. Our overarching goal is to determine the relative importance of piston and preferential flow in groundwater recharge using multiple tracers and quantify the effects of land use change on groundwater recharge. We find that while both piston and preferential flows are important in groundwater recharge, the unsaturated and saturated zones have yet to come to hydraulic equilibrium. This suggests different groundwater recharge mechanisms: tracers in the unsaturated zone suggest piston flow, while the detectable tritium in the saturated zone implies preferential flow. Recharge rates in the unsaturated zones range between 23 and 82 mm year(-1), accounting for 4%-14% of mean annual precipitation, and increasing with depth presumably because of land use and/or climatic conditions. Total recharge rate in the saturated zone is 112.6 +/- 44.1 mm year(-1), accounting for 19 +/- 9% of mean annual precipitation. Overall, our study finds that piston flow contributes more to total recharge (53%-69%) than does preferential flow. Nevertheless, piston flow may become less important because of land use change (farmland to apple orchard conversion). Our findings have implications for the need to strike a delicate balance between the economic gains from afforestation and the possible risks to groundwater supply sustainability.
URL [本文引用: 5]
[本文引用: 2]
[本文引用: 1]
URL [本文引用: 1]
URL
URL [本文引用: 2]
URL
URL [本文引用: 7]
[本文引用: 2]
[本文引用: 1]
[本文引用: 1]
[本文引用: 1]
[本文引用: 1]
URL [本文引用: 1]
URL [本文引用: 1]
URL [本文引用: 4]
[本文引用: 1]
[本文引用: 1]
URL [本文引用: 1]
URL [本文引用: 2]
URL [本文引用: 1]
[本文引用: 2]

Soil water and carbon stocks have always been research hotspots. However, the interaction between soil water and carbon in deep soil (>1 m below surface) remains poorly understood. The present study used the chronosequence approach to investigate water extraction and carbon input by roots to a depth of 25.2 m in 8-, 11-, 15-, 18-, and 22-year-old afforested apple (Mains pumila Mill.) orchard stands in a sub-humid region of the Chinese Loess Plateau. Three long-term cultivated farmlands were used as a benchmark of soil water and carbon status before land use change. Measurements showed that the apple trees accessed deep soil water reserves by growing deep roots, with the resulting desiccated soil possibly stimulating apple trees to extend their roots into deeper, moister soil. Accordingly, soil water content in the root zone decreased progressively with increasing stand age. For example, the roots of apple trees in the 22-year-old stand extended to 23.2 m below the soil surface and extracted 1530 +/- 43 mm deep soil water. Consequently, carbon input from root biomass correlated well with the water storage loss in deep soil (R-2 = 0.88). Deep roots accounted for 49 +/- 22% of the total root biomass and contributed 0.44 +/- 0.15 Mg C ha(-1 )yr(-1) to the deep soil. However, the roots of apple trees did not significantly change the soil organic carbon content in the root zone possibly because there was limited root biomass per unit soil depth and because soil water content in the root zone gradually decreased. These findings demonstrate the importance of deep soil in regulating water and carbon cycles, advancing our understanding of interactions among water, roots, and carbon in this zone. (C) 2019 Elsevier B.V.
[本文引用: 1]

Apportioning recharge to piston flow and preferential flow is critical for groundwater resource management and hydrologic connectivity evaluation, but the occurrence of the two flows in thick unsaturated zones is still poorly understood. The main objective of this study was to quantify the relative contributions of these two flows in the deep unsaturated zone of the Loess Plateau, China (CLP). The Bayesian mixing model (MixSIAR) was integrated with the line conditioned excess (lc-excess) of stable isotopes (delta H-2 and delta O-18) in precipitation, soil water (8-25 m), and groundwater to determinate the contributions. Piston flow contributed 62 +/- 8% to the total recharge while preferential flow did only 38 +/- 8%, indicating piston flow was the dominant groundwater recharge mechanism. On comparison with results from similar study sites in the CLP, the primary groundwater recharge mechanism differed among sites and may depend on spatial scales or geomorphology. The information is critical for understanding hydrological process and evaluating groundwater quantity and quality in the highlands of the CLP and other regions with similar hydrogeological conditions.
URL [本文引用: 2]
[本文引用: 2]
[本文引用: 2]