李艺1,彭宏熙2,陈环宇3,柳景青1
(1.浙江大学 建筑工程学院,杭州 310058; 2.中水珠江规划勘测设计有限公司 市政与环境设计所,广州 510610; 3.浙江大学 滨海产业技术研究院,天津 300301)
摘要:
为研究供水管道材料对管道生物膜致病菌种的影响,以已稳定运行8年的中国东部某城市实际供水管道中试平台管道为实验对象,从平行设置的球墨铸铁管(DCIP)、高密度聚乙烯管(HDPE)、镀锌钢管(GSP)、不锈钢复合管(SSCP)和钢管(SP)管道中收集13组生物膜样品,开展宏基因组测序分析,对比研究不同管道材料的致病菌种类分布情况。结果表明:DCIP生物膜中致病菌相对含量最为丰富,GSP致病菌相对含量最少;此外,世界卫生组织颁布的12种全球优先致病菌(priority pathogens)在本次供水管道中发现9种,在不同管道的相对丰度为0.079%~1.084%,表明供水管道生物膜对人类和环境的严重危害潜力;依据致病菌在5种管材中相对丰度的分布情况,发现与5种管材相关联的优势致病菌。研究结果为基于致病风险控制的供水管道管材选择提供了理论依据。
关键词: 供水管道 生物膜 致病菌分布 管材影响 宏基因组测序
DOI:10.11918/202007136
分类号:TU991
文献标识码:A
基金项目:国家自然科学基金(52070166)
Effects of pipe materials on pathogens in biofilms by metagenomic sequencing
LI Yi1,PENG Hongxi2,CHEN Huanyu3,LIU Jingqing1
(1.College of Civil Engineering and Architecture, Zhejiang University, Hangzhou 310058, China; 2.Institute of Municipal and Environmental Design, China Water Resources Pearl River Planning Surveying & Designing Co., Ltd., Guangzhou 510610, China; 3.Binhai Industrial Technology Research Institute, Zhejiang University, Tianjin 300301, China)
Abstract:
To investigate the influence of pipe materials on species of pathogens in water distribution system, a drinking water distribution system (DWDS) in a city in eastern China which has been operating steadily for eight years was taken as the experimental object. Metagenomic sequencing analysis was conducted to study the effects of pipeline materials on pathogens in 13 sets of biofilms collected from ductile iron pipe (DCIP), polyethylene pipe (HDPE), galvanized steel pipe (GSP), stainless steel composite pipe (SSCP), and steel pipe (SP). Results show that the relative abundance of pathogens in DCIP biofilm was the most, while that in GSP biofilm was the least. In addition, nine of 12 pathogens on the global priority pathogens list published by WHO were detected from the water distribution pipeline, with relative abundance ranged from 0.079% to 1.084%, indicating the severe hazard potential of biofilms in water supply pipelines to humans and the environment. Based on the relative abundance distributions of the pathogens in five pipe materials, the priority pathogens associated with the five pipe materials were found. The research results provide an effective and theoretical basis for the selection of water distribution pipe materials.
Key words: drinking water distribution system (DWDS) biofilm pathogens distribution pipe materials metagenomic sequence
李艺, 彭宏熙, 陈环宇, 柳景青. 宏基因组测序法研究管材对生物膜致病菌的影响[J]. 哈尔滨工业大学学报, 2021, 53(11): 21-28. DOI: 10.11918/202007136.

LI Yi, PENG Hongxi, CHEN Huanyu, LIU Jingqing. Effects of pipe materials on pathogens in biofilms by metagenomic sequencing[J]. Journal of Harbin Institute of Technology, 2021, 53(11): 21-28. DOI: 10.11918/202007136.

基金项目 国家自然科学基金(52070166) 作者简介 李艺(1996—),女,硕士研究生;
柳景青(1972—),男,教授,博士生导师 通信作者 柳景青,liujingqing@zju.edu.cn 文章历史 收稿日期: 2020-07-28
Abstract Full text Figures/Tables PDF
宏基因组测序法研究管材对生物膜致病菌的影响
李艺1, 彭宏熙2, 陈环宇3, 柳景青1


1. 浙江大学 建筑工程学院,杭州 310058;
2. 中水珠江规划勘测设计有限公司 市政与环境设计所, 广州 510610;
3. 浙江大学 滨海产业技术研究院,天津 300301
收稿日期: 2020-07-28
基金项目: 国家自然科学基金(52070166)
作者简介: 李艺(1996—),女,硕士研究生; 柳景青(1972—),男,教授,博士生导师
通信作者: 柳景青,liujingqing@zju.edu.cn
摘要: 为研究供水管道材料对管道生物膜致病菌种的影响,以已稳定运行8年的中国东部某城市实际供水管道中试平台管道为实验对象,从平行设置的球墨铸铁管(DCIP)、高密度聚乙烯管(HDPE)、镀锌钢管(GSP)、不锈钢复合管(SSCP)和钢管(SP)管道中收集13组生物膜样品,开展宏基因组测序分析,对比研究不同管道材料的致病菌种类分布情况。结果表明:DCIP生物膜中致病菌相对含量最为丰富,GSP致病菌相对含量最少;此外,世界卫生组织颁布的12种全球优先致病菌(priority pathogens)在本次供水管道中发现9种,在不同管道的相对丰度为0.079%~1.084%,表明供水管道生物膜对人类和环境的严重危害潜力;依据致病菌在5种管材中相对丰度的分布情况,发现与5种管材相关联的优势致病菌。研究结果为基于致病风险控制的供水管道管材选择提供了理论依据。
关键词: 供水管道 生物膜 致病菌分布 管材影响 宏基因组测序
Effects of pipe materials on pathogens in biofilms by metagenomic sequencing
LI Yi1, PENG Hongxi2, CHEN Huanyu3, LIU Jingqing1


1. College of Civil Engineering and Architecture, Zhejiang University, Hangzhou 310058, China;
2. Institute of Municipal and Environmental Design, China Water Resources Pearl River Planning Surveying & Designing Co., Ltd., Guangzhou 510610, China;
3. Binhai Industrial Technology Research Institute, Zhejiang University, Tianjin 300301, China
Abstract: To investigate the influence of pipe materials on species of pathogens in water distribution system, a drinking water distribution system (DWDS) in a city in eastern China which has been operating steadily for eight years was taken as the experimental object. Metagenomic sequencing analysis was conducted to study the effects of pipeline materials on pathogens in 13 sets of biofilms collected from ductile iron pipe (DCIP), polyethylene pipe (HDPE), galvanized steel pipe (GSP), stainless steel composite pipe (SSCP), and steel pipe (SP). Results show that the relative abundance of pathogens in DCIP biofilm was the most, while that in GSP biofilm was the least. In addition, nine of 12 pathogens on the global priority pathogens list published by WHO were detected from the water distribution pipeline, with relative abundance ranged from 0.079% to 1.084%, indicating the severe hazard potential of biofilms in water supply pipelines to humans and the environment. Based on the relative abundance distributions of the pathogens in five pipe materials, the priority pathogens associated with the five pipe materials were found. The research results provide an effective and theoretical basis for the selection of water distribution pipe materials.
Keywords: drinking water distribution system (DWDS) biofilm pathogens distribution pipe materials metagenomic sequence
虽然水厂的出厂水是以《生活饮用水卫生标准》(GB 5749—2006)作为标准,但是饮用水从水厂到用户之间还需要经过长距离的供水管网系统,供水管道生物膜会引起大量致病菌和致病菌的滋生[1-2],生物膜的脱落会恶化水质,最终影响用户端的用水水质。最近的流行病学报告指出,供水管道系统是疾病暴发的重要来源[3]。因此,当供水管道生物膜中存在致病菌时,人体接触饮用水会导致健康风险。
致病菌在供水管道生物膜中广泛存在。Wang等[4]在模拟供水系统(simulated distribution system, SDS)生物膜中发现了军团菌属(Legionella spp.)、分枝杆菌属(Mycobacterium spp.)、假单胞菌属(Pseudomonas spp.)、棘阿米巴属(Acanthamoeba spp.),还发现了芽孢杆菌属(Bacillus spp.)。Azevedo等[5]在实际管道生物膜中分离出了幽门螺旋杆菌(Helicobacter pylori)。供水管道生物膜的生长与许多因素相关,如管材[6]、有机物浓度[7]、消毒剂[8]、水力停留时间[9],其中,管道材料被认为是影响生物膜中致病菌生长的重要因素。Wang等[10-11]在SDS中发现管道材料是控制饮用水微生物群落的主要因素,也影响特定致病菌的分布,相较PVC管道,水泥管或铁管更利于水中分枝杆菌生长,同时,水泥管比铁管和PVC管道更利于水中铜绿假单胞菌(Pseudomonas aeruginosa)生长。Proctor等[11]发现铜管相对于交联聚乙烯(PEX)抑制嗜肺军团菌和军团菌的生长;Ren等[12]利用培养的方法发现,灰口铸铁管比DCIP和SSCP有更低相对丰度,含有潜在致病菌属的相对丰度最低。因为很难从实际供水管道中获得生物膜样品,以往研究通常使用反应器和模拟管道[13],模拟条件无法准确代表在复杂环境中运行的实际供水管道细菌群落的特征[14]。
16S rRNA基因扩增子测序已经广泛运用于检测饮用水管网[15]、污水[16]、活性污泥、河水[17]、海洋[18]等环境中的潜在致病菌属。在饮用水领域中,有大量研究利用16S rRNA方法研究管材[4, 19-20]、消毒剂、水龄[4, 10]、空间位置[15, 21]等对供水管道生物膜微生物群落结构和潜在致病菌属群落的影响。然而,由于16S rRNA基因测序相对较差的分辨率,无法精确到细菌的种水平,而大多潜在致病菌属包含许多非致病的菌种,16S rRNA基因测序可能会高估致病菌的相对丰度,也会忽略一些稀有致病菌[22];与16S rRNA扩增子测序相比,鸟枪宏基因组测序可以全面概述各种环境中的微生物群落[23-24]和功能基因[25-27],并已被利用在饮用水系统中检测致病菌[26]。
在华东某城市的供水管道中试平台收集球墨铸铁管(DCIP)、高密度聚乙烯管(HDPE)、镀锌钢管(GSP)、不锈钢复合管(SSCP)和钢管(SP)管道中的生物膜,利用宏基因组测序,以实际供水管道微生物膜为研究对象,比较不同管道材料中的致病菌群落差异。本研究为饮用水供水管道生物膜中的细菌致病菌分布提供了新的见解,为供水管道管材与生物膜致病菌分布的关联性解析提供依据。
1 实验 1.1 供水管道生物膜的中试平台设计以中国东部某城市SX的供水管网系统为研究对象。该城市供水水源为水库水,属于Ⅱ类水,经过混凝—沉淀—砂滤—氯化(氯气)工艺处理后进入供水管网。中试平台建立在管网末端,距离水厂约22 km,该中试平台于2009年6月建成并投入使用。如图 1所示,该平台内布置5组并联管线,每组管线由长0.5 m的管段串联而成,管道内径分为DN80和DN150两种。DCIP、GSP、SSCP和SP管径DN80,HDPE管径DN150。中试平台与实际管网水相连,进水由实际管网接入,出水排入下游实际管网。
Fig. 1
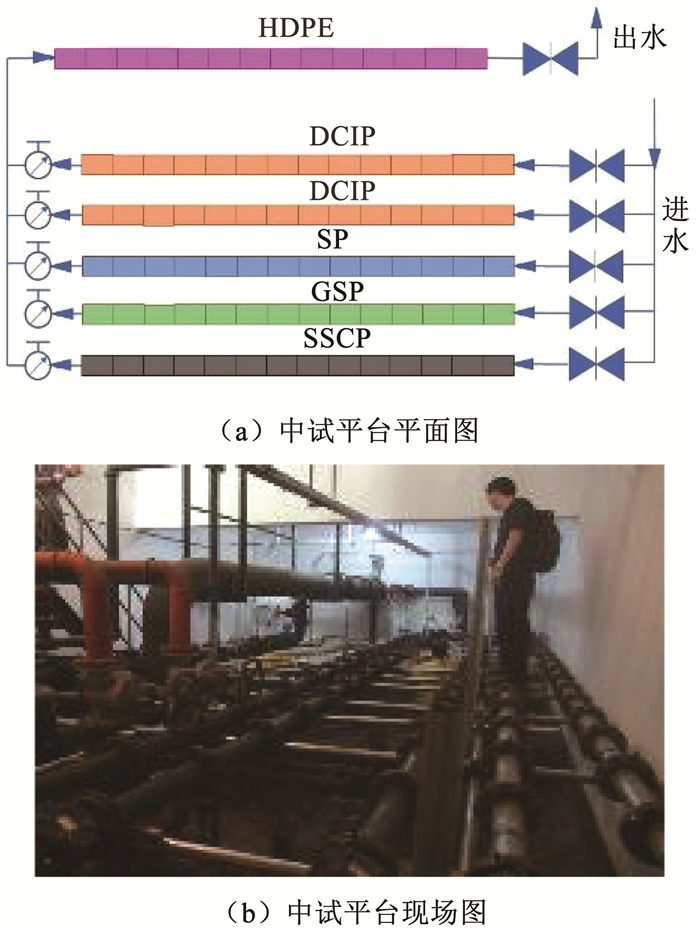
1.2 采样管道的信息2016年7月与2017年7月在该中试平台进行生物膜采样,共采集13个生物膜样本。采样管道基本信息见表 1。
表 1
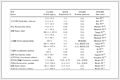
DCIP.1 DCIP 80 1 2016年7月
DCIP.2 2 2016年7月
DCIP.3 3 2016年7月
HDPE.1 HDPE 150 1 2017年7月
HDPE.3 3 2016年7月
GSP.1 GSP 80 1 2016年7月
GSP.2 2 2016年7月
GSP.3 3 2016年7月
SSCP.1 SSCP 80 1 2016年7月
SSCP.2 2 2016年7月
SSCP.3 3 2016年7月
SP.1 SP 80 1 2017年7月
SP.3 3 2016年7月
表 1 采样管道基本信息 Tab. 1 Basic information of sample pipes
1.3 生物膜现场采集与预处理方法在采集生物膜样品前,需完成平台进水水样采集。水样采集完成后,切断该平台的供水,为了防止生物膜脱落,缓慢排掉装置中的水,之后卸下管段并移至干净场所,用已灭菌的刷子、三脚架、托盘和磷酸盐缓冲盐水(PBS)对管段生物膜进行采集[28]。将刷下来的生物膜样品放在利用冰袋维持低温的环境中,并在2 h内送到实验室,保存在4 ℃冰箱中。
为保证实验结果的准确性,生物膜样品需要在采集后的24 h内进行预处理,预处理流程按照Peng等[29]制定的方法进行。
滤膜上的微生物用FastDNA SPIN kit for Soil(MP Biomedicals,美国)提取样品的基因组DNA,具体操作步骤按照说明书进行。提取后的DNA被定容于50 μL无核酸酶溶液(DNase/Pyrogen-free solution)中,最后保存于-80 ℃冰箱。
1.4 理化指标检测方法水体中的pH、溶解氧(dissolved oxygen, DO)、温度、余氯、浑浊度、总有机碳(total organic carbon, TOC)、总磷、总氮、氨氮等指标的检测方法均按照文献[30]的步骤进行。
1.5 宏基因组测序将生物膜样品送至华大基因(中国)与浙江大学农生环测试中心进行高通量测序,使用Illumina HiSeq X Ten平台进行双端150 bp的宏基因组测序。本文分析的宏基因组测序原数据已保存到美国国家生物技术信息中心(NCBI)序列读物档案库(SRA)中,登录号为PRJNA 659358。
1.6 数据分析方法将测序公司返回的FASTQ格式的原始测序数据用SolexaQA2[31]进行质量检测,只保留Phred质量分数大于等于20的序列[21]。每个样本的clean data大约有10 GB。然后将序列上传MG-RAST平台(版本4.0.3)[32],与Silva-SSU、SEED Subsystem数据库进行比对,比对条件为:E截止值为10-5,最小识别率为60%,最小比对长度为50 bp,比对结果即为样品中微生物群落组成和功能基因分布。
先前已有研究对抗生素抗性基因进行归一化处理[33-34],本文的抗生素抗性基因的类型丰度以10-6为单位,即百万个读数中的一个读数[35-36]。
将所有测定数据导入Excel进行初步整理,所有统计分析均使用统计软件IBM SPSS Statistics 24和R Studio进行。基于Bray-Curtis距离对生物膜细菌群落进行聚类分析,并使用主坐标分析(PCoA)图进行可视化。使用Bray-Curtis距离矩阵(具有999个排列)进行相似性分析(ANOSIM),研究不同管材样本之间差异的显着性。统计检验在P<0.05被认为显著。
2 结果与分析 2.1 中试平台进水的理化指标表 2显示了中试平台进水水质的理化性质,菌落总数超过《生活饮用水卫生标准》(GB 5749—2006)要求的100 CFU/mL,其余指标均符合国标。
表 2
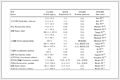
余氯 mg·L-1 0.24±0.05
DO mg·L-1 6.69±0.5
浊度 NTU 0.67±0.3
pH 5.98±0.1
总磷 mg·L-1 0.003±0.001
总氮 mg·L-1 3.1±0.1
HPC CFU·mL-1 140±20
氨氮 mg·L-1 0.016±0.003
TOC mg·L-1 1.37±0.2
Fe mg·L-1 0
Mn mg·L-1 0.11±0.05
Zn mg·L-1 0.06±0.01
Mg mg·L-1 1.7±0.2
电导率 μS·cm-1 166.5±10
表 2 中试平台进水水质 Tab. 2 Water quality parameters in pilot platform
2.2 不同管材细菌群落结构分布如图 2所示,在DCIP的生物膜中,其主要细菌属,按相对丰度的降序排列,是假单胞菌属(4.40%~22.33%)与黄杆菌属(Flavobacterium spp.)(2.09%~18.35%),这与之前美国伊利诺伊州进行的DWDS研究一致[37]。在HDPE生物膜中,其主要细菌属是不动杆菌属(Acinetobacter spp.) (0.05%~34.66%)与黄杆菌属(3.17%~10.63%),这与先前针对PVC和PEX管道进行的研究一致[38]。在GSP生物膜中,其主要细菌属是土生单胞菌属(Terrimonas spp.)(1.45%~21.87%)与马赛菌属(Massilia spp.)(4.92%~21.04%)。在SSCP生物膜中,其主要细菌属是鞘氨醇单胞菌属(Sphingomonas spp.)(2.84%~24.43%)与短波单胞菌属(Brevundimonas spp.)(4.71%~10.13%),先前也曾在DWDS生物膜中发现过这两种细菌[39]。在SP生物膜中,其主要细菌属是噬几丁质菌属(Chitinophaga spp.)(0.35%~27.52%)与不动杆菌属(5.12%~7.10%)。
Fig. 2
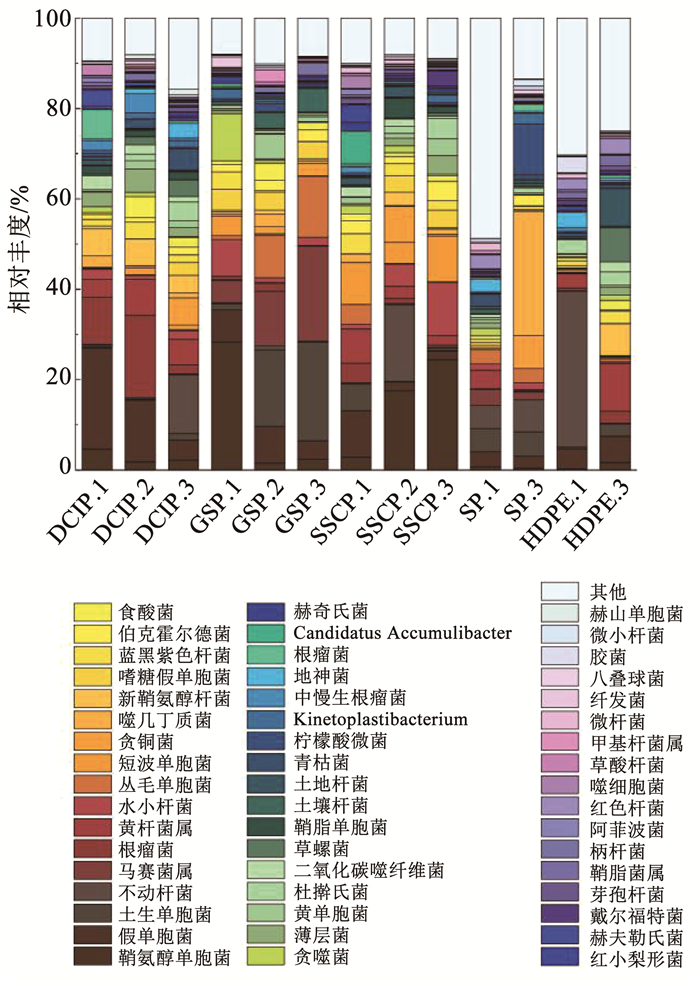
如图 3所示,PCoA图显示不同管材生物膜细菌群落之间分离清晰,揭示了不同管材生物膜细菌群落区别明显。相似性分析(ANOSIM)确认差异显著(R=0.517,P<0.01),表明管道材料在微生物群落中起着重要的作用。
Fig. 3
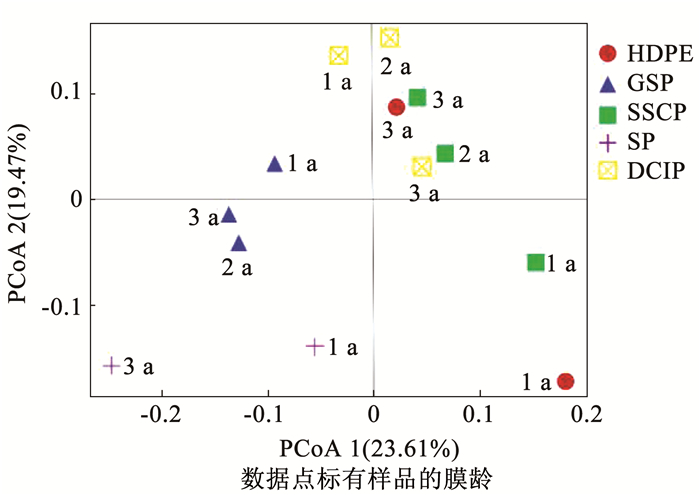
2.3 使用鸟枪宏基因组测序鉴定致病菌结果为了确定致病菌,将13个样本的宏基因组数据分类学结果与包含538种致病菌的致病菌数据库[40]进行了比较,在所有13个样本中共鉴定出96个属149种的致病菌。如图 4所示,5种管材共享15种致病菌,这些致病菌总量占据每种管材相对丰度77%~93%。DCIP生物膜中致病菌种类最为丰富,发现86种致病菌,GSP致病菌种类最少,发现55种致病菌。DCIP特有致病菌为22种,数量最为丰富,相对丰度为3.7%;GSP生物膜特有致病菌为8种,数量最少,相对丰度为2.6%。
Fig. 4
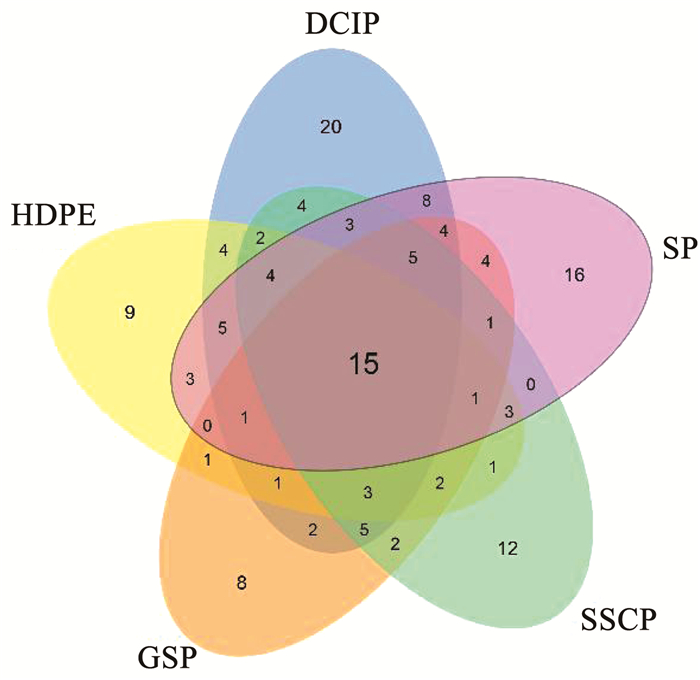
如图 5所示,DCIP生物膜中的致病菌平均相对丰度最高,达6.4%,GSP生物膜中的致病菌平均相对丰度最低,为1.61%,两种管材之间生物膜致病菌群落具有显著差异(P<0.05)。致病菌种的平均相对丰度在5种管材分布情况为DCIP>SP>HDPE>SSCP>GSP,以往的研究也证实了这一点。Srijan等[41]发现SSCP是金属管中控制致病菌的最佳管材,与DCIP相比,SSCP表面光滑且耐腐蚀,不利于微生物的附着。对于GSP的研究尚少,Silhan等[42]发现相比塑料管与铜管,大肠杆菌在GSP上的存活时间最短,GSP更不利于致病菌生存。DCIP具有较高的表面粗糙度,有利于微生物在内壁滋生[43],此外,铁是细菌繁殖必不可少的元素,当从铁管中释放后,会促进生物膜的生长[4],还可以消耗残留的消毒剂[44],从而提高致病菌的存活率。重要的是致病菌获取铁与引发细菌性疾病相关,铁的缺失会降低细菌的毒力[45]。本研究同样证明了这一点(图 6),基于SEED subsystem分类的一级功能组成显示,DCIP生物膜中"铁的摄取与代谢"平均相对丰度在5种管材中最高,明显高于GSP生物膜(P<0.05)。5种管材中,含致病菌最为丰富的属为不动杆菌属,其次是假单胞菌属、从毛单胞菌属(Comamonas spp.)、短波单胞菌属、罗尔斯通氏菌属。
Fig. 5
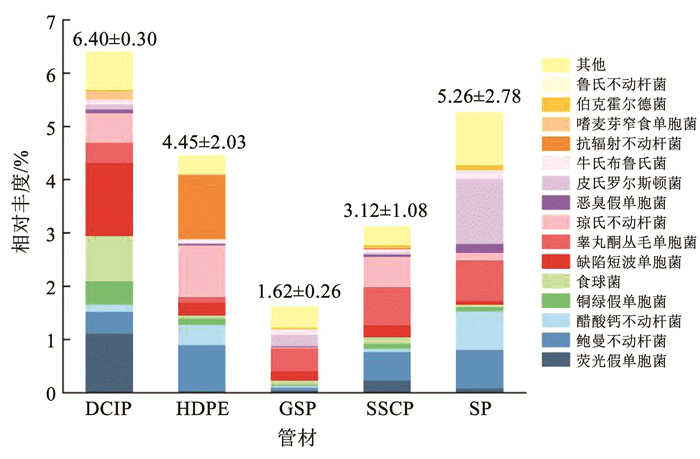
Fig. 6
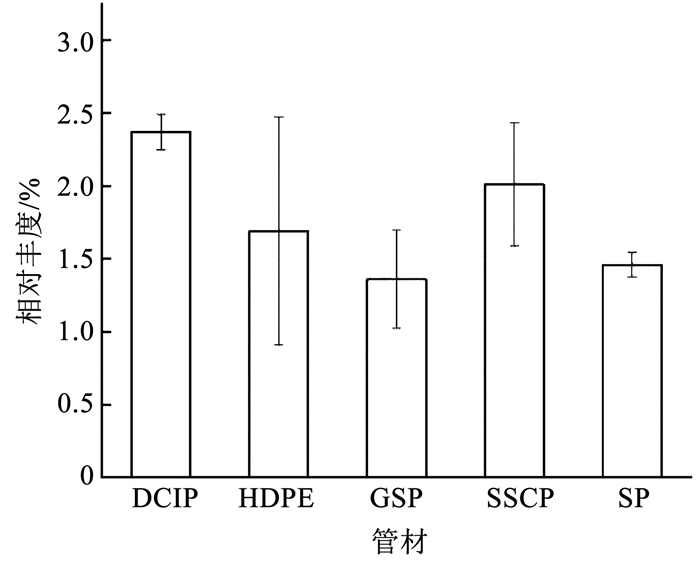
2.4 不同管材相关致病菌的识别在所有鉴定出的致病菌种中,荧光假单胞菌(Pseudomonas fluorescens)、鲍曼不动杆菌(Acinetobacter baumannii)、醋酸钙不动杆菌(Acinetobacter calcoaceticus) 和铜绿假单胞菌是4种含量最丰富的致病菌,也是目前常被研究的典型致病菌。相比其他4种管材,如图 7所示,鲍曼不动杆菌和醋酸钙不动杆菌更偏向在HDPE和SP上分布,这与不动杆菌属分布情况相似。细菌荧光假单胞菌与铜绿假单胞菌更易在DCIP上生长,与假单胞菌属分布情况相似,这证实了课题组之前的研究结果——黄杆菌属、短杆菌属(Brevibacterium spp.)、假单胞菌属等菌属对铜绿假单胞菌的生长具有促进作用。有基于模拟供水管道的研究发现铜绿假单胞菌在铁管生物膜中的含量少于PE管和水泥管[46],这与本文所得结论相悖,表明模拟供水管道与真实管道中的致病菌分布有差异。此发现对供水管网致病菌研究起到指导意义。
Fig. 7
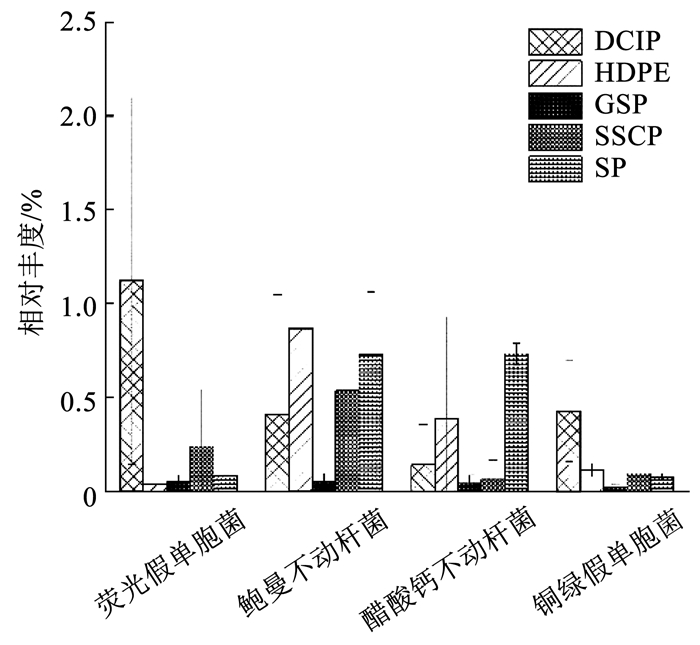
在这项研究中,为了识别与5种管道材料相关的致病菌,规定两项严格的标准:1)在某一种管材生物膜中观察到该种致病菌的相对丰度占所有5种管材相对丰度之和的60%以上;2)该种致病菌在5种管材生物膜上的总相对丰度在0.5%以上[40]。将满足上述两个条件的致病菌分别称称为DCIP、HDPE、GSP、SSCP和SP相关致病菌,得到以下5种分布特征(如表 3):1)DCIP相关致病菌,如荧光假单胞菌、铜绿假单胞菌、狗咬二氧化碳嗜纤维菌(Capnocytophaga cynodegmi)、缺陷短波单胞菌(Brevundimonas diminuta)、嗜麦芽窄食单胞菌(Stenotrophomonas maltophilia)、类产碱假单胞菌(Pseudomonas pseudoalcaligenes)、无乳链球菌(Streptococcus agalactia)和豚鼠气单胞菌(Aeromonas caviae)。2)HDPE相关致病菌,如抗辐射不动杆菌(Acinetobacter radioresistens)。3)GSP相关致病菌,如食酸戴尔福特菌(Delftia acidovorans)。4)SSCP相关致病菌,如约翰逊不动杆菌(Acinetobacter johnsonii)。5)SP相关致病菌,如洋葱伯克霍尔德菌(Burkholderia cepacia)、浅绿气球菌(Aerococcus viridans)、成团泛菌(Pantoea agglomerans)和皮氏罗尔斯顿菌(Ralstonia pickettii)。
表 3
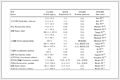
DCIP HDPE GSP SSCP SP
首要 鲍曼不动杆菌 碳青霉烯 0.408±0.639 0.864±1.205 0.051±0.041 0.537±0.893 0.725±0.336
铜绿假单胞菌 碳青霉烯 0.427±0.269 0.113±0.034 0.018±0.019 0.093±0.106 0.073±0.019
肠杆菌科 碳青霉烯、头孢菌素 0.008±0.011 0.021±0.030 0.006±0.003 0.002±0.003 0.194±0.248
高 沙门氏菌属 氟喹诺酮 0 0.017±0.024 0 0 0.059±0.084
金黄色葡萄球菌 甲氧西林、万古霉素 0.003±0.004 0 0.003±0.005 0 0.027±0.029
弯曲菌属 氟喹诺酮 0 0.004±0.006 0 0 0
粪肠球菌 万古霉素 0.002±0.004 0 0 0 0
幽门螺旋菌 克拉霉素 0 0 0 0.001±0.002 0
中 志贺氏菌属 氟喹诺酮 0.002±0.004 0.013±0.018 0 0 0.006±0.008
总和 0.850±0.475 1.032±1.30 0.079±0.056 0.633±0.85 1.084±0.022
表 3 不同管材生物膜中9种全球优先致病菌的平均相对丰度 Tab. 3 Average relative abundance of nine priority pathogens in biofilms from different pipe materials
2.5 不同管材生物膜中抗生素耐药全球优先致病菌2017年,世界卫生组织(WHO)首次发布了全球关于抗生素耐药细菌的优先致病菌(priority pathogens)名单,以帮助研究和开发抗生素耐药性的新疗法[47]。该名单一共包含了12类致病菌,分成3个优先级,即首要、高级和中级。本研究在5种供水管道生物膜中共检测到9类全球优先致病菌(本文中出现的所有全球优先致病菌不一定携带耐药基因)。如表 3所示,在5种管材中,HDPE和SP生物膜中的优先致病菌含量最为丰富,分别为1.032%和1.084%,GSP的最低,为0.079%。根据对人类健康的危害,耐碳青霉烯的鲍曼不动杆菌在WHO的优先致病菌名单占据首位,铜绿假单胞菌占据第二位,这两种细菌也在本研究被大量发现,可见供水管道微生物膜的潜在危害性。级别为首要的3类致病菌占总优先致病菌总数的91%~99%,这3种菌均对碳青霉烯类药物产生抵抗性,表 4显示了与碳青霉烯抗性相关的β-内酰胺酶基因的丰度。
表 4
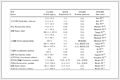
相对丰度/10-6 2 310±250 1 820±1 730 2 530±340 2 150±130 1 620±390
表 4 不同管材生物膜中β-内酰胺酶基因的丰度 Tab. 4 Abundance of β-lactamase in biofilms from different pipe materials
值得明确的是,本研究中获得的基因组信息囊括了活细菌和死细菌细胞提取的所有DNA,因此,这可能会高估有致病能力细菌的相对丰度。本研究虽然在供水系统中检测出致病菌,由于没有临床毒理实验的佐证,致病菌的致病效果还有待进一步研究。
3 结论1) DCIP生物膜中致病菌种类与含量相对最为丰富,其次为钢管,GSP生物膜中致病菌种类与含量最少,致病能力较强。
2) HDPE和SP生物膜的全球优先致病菌含量最为丰富,GSP的最低,为0.079%。
3) 确定5种管材的相关致病菌:DCIP相关致病菌,如荧光假单胞菌、铜绿假单胞菌、狗咬二氧化碳嗜纤维菌等;HDPE相关致病菌,如抗辐射不动杆菌;GSP相关致病菌,如食酸戴尔福特菌;SSCP相关致病菌, 如约翰逊不动杆菌;SP相关致病菌,如洋葱伯克霍尔德菌、浅绿气球菌等。
参考文献
[1] WINGENDER J, FLEMMING H C. Biofilms in drinking water and their role as reservoir for pathogens[J]. International Journal of Hygiene and Environmental Health, 2011, 214(6): 417. DOI:10.1016/j.ijheh.2011.05.009
[2] DOUTERELO I, CALERO-PRECIADO C, SORIA-CARRASCO V, et al. Whole metagenome sequencing of chlorinated drinking water distribution systems[J]. Environmental Science: Water Research and Technology, 2018, 4(12): 2080. DOI:10.1039/C8EW00395E
[3] BENEDICT K M, RESES H, VIGAR M, et al. Surveillance for waterborne disease outbreaks associated with drinking water-United States, 2013-2014[J]. Morbidity and Mortality Weekly Report, 2017, 64(31): 842.
[4] WANG H, MASTERS S, EDWARDS M A, et al. Effect of disinfectant, water age, and pipe materials on bacterial and eukaryotic community structure in drinking water biofilm[J]. Environmental Science and Technology, 2014, 48(3): 1426. DOI:10.1021/es402636u
[5] AZEVEDO N F, ALMEIDA C, CERQUEIRA L, et al. Coccoid form of Helicobacter pylori as a morphological manifestation of cell adaptation to the environment[J]. Applied and Environmental Microbiology, 2007, 73(10): 3423. DOI:10.1128/AEM.00047-07
[6] ALLION A, LASSIAZ S, PEGUET L, et al. A long term study on biofilm development in drinking water distribution system: comparison of stainless steel grades with commonly used materials[J]. Revue de Metallurgie, 2011, 108(4): 259. DOI:10.1051/metal/2011063
[7] FALKINHAM J O, NORTON C D, LECHEVALLIER M W. Factors influencing numbers of Mycobacterium avium, Mycobacterium intracellulare, and other Mycobacteria in drinking water distribution systems[J]. Applied and Environmental Microbiology, 2001, 67(3): 1225. DOI:10.1128/AEM.67.3.1225-1231.2001
[8] MOORE M R, PRYOR M, FIELDS B, et al. Introduction of monochloramine into a municipal water system: impact on colonization of buildings by Legionella spp[J]. Applied and Environmental Microbiology, 2006, 72(1): 378. DOI:10.1128/AEM.72.1.378-383.2006
[9] SIDARI F P, STOUT J E, SCOTT DUDA D G, et al. Maintaining Legionella control in building water systems[J]. Journal (American Water Works Association), 2014, 106(10): 24. DOI:10.5942/jawwa.2014.106.0147
[10] WANG H, MASTERS S, HONG Y, et al. Effect of disinfectant, water age, and pipe material on occurrence and persistence of legionella, mycobacteria, pseudomonas aeruginosa, and two amoebas[J]. Environmental Science and Technology, 2012, 46(21): 11566. DOI:10.1021/es303212a
[11] PROCTOR C R, DAI D, EDWARDS M A, et al. Interactive effects of temperature, organic carbon, and pipe material on microbiota composition and Legionella pneumophila in hot water plumbing systems[J]. Microbiome, 2017, 5(1): 130. DOI:10.1186/s40168-017-0348-5
[12] REN H, WANG W, LIU Y, et al. Pyrosequencing analysis of bacterial communities in biofilms from different pipe materials in a city drinking water distribution system of East China[J]. Applied Microbiology and Biotechnology, 2015, 99(24): 10713. DOI:10.1007/s00253-015-6885-6
[13] REVETTA R P, GOMEZ-ALVAREZ V, GERKE T L, et al. Establishment and early succession of bacterial communities in monochloramine-treated drinking water biofilms[J]. FEMS Microbiology Ecology, 2013, 86(3): 404. DOI:10.1111/1574-6941.12170
[14] DOUTERELO I, HUSBAND S, BOXALL J B. The bacteriological composition of biomass recovered by flushing an operational drinking water distribution system[J]. Water Research, 2014, 54: 100. DOI:10.1016/j.watres.2014.01.049
[15] EL-CHAKHTOURA J, PREST E, SAIKALY P, et al. Dynamics of bacterial communities before and after distribution in a full-scale drinking water network[J]. Water Research, 2015, 74: 180. DOI:10.1016/j.watres.2015.02.015
[16] FANG T, CUI Q, HUANG Y, et al. Distribution comparison and risk assessment of free-floating and particle-attached bacterial pathogens in urban recreational water: implications for water quality management[J]. Science of the Total Environment, 2018, 613: 428. DOI:10.1016/j.scitotenv.2017.09.008
[17] KORAJKIC A, PARFREY L W, MCMINN B R, et al. Changes in bacterial and eukaryotic communities during sewage decomposition in Mississippi river water[J]. Water Research, 2015, 69: 30. DOI:10.1016/j.watres.2014.11.003
[18] HONG Y, YIN B, ZHENG T. Diversity and abundance of anammox bacterial community in the deep-ocean surface sediment from equatorial Pacific[J]. Applied Microbiology and Biotechnology, 2011, 89(4): 1233. DOI:10.1007/s00253-010-2925-4
[19] WANG H, EDWARDS M, FALKINHAM J O, et al. Molecular survey of the occurrence of legionella spp., mycobacterium spp., pseudomonas aeruginosa, and amoeba hosts in two chloraminated drinking water distribution systems[J]. Applied and Environmental Microbiology, 2012, 78(17): 6285. DOI:10.1128/AEM.01492-12
[20] LIN W, YU Z, CHEN X, et al. Molecular characterization of natural biofilms from household taps with different materials: PVC, stainless steel, and cast iron in drinking water distribution system[J]. Applied Microbiology and Biotechnology, 2013, 97(18): 8393. DOI:10.1007/s00253-012-4557-3
[21] ZHANG Y, OH S, LIU W T. Impact of drinking water treatment and distribution on the microbiome continuum: an ecological disturbance's perspective[J]. Environmental Microbiology, 2017, 19(8): 3163. DOI:10.1111/1462-2920.13800
[22] SEGATA N, WALDRON L, BALLARINI A, et al. Metagenomic microbial community profiling using unique clade-specific marker genes[J]. Nature Methods, 2012, 9(8): 811. DOI:10.1038/nmeth.2066
[23] LIAO X, CHEN C, WANG Z, et al. Pyrosequencing analysis of bacterial communities in drinking water biofilters receiving influents of different types[J]. Process Biochemistry, 2013, 48(4): 703.
[24] MA L, LI B, ZHANG T. New insights into antibiotic resistome in drinking water and management perspectives: a metagenomic based study of small-sized microbes[J]. Water Research, 2019, 152: 191. DOI:10.1016/j.watres.2018.12.069
[25] DOUTERELO I, DUTILH B E, ARKHIPOVA K, et al. Microbial diversity, ecological networks and functional traits associated to materials used in drinking water distribution systems[J]. Water Research, 2020, 173: 115586. DOI:10.1016/j.watres.2020.115586
[26] HUANG K, ZHANG X X, SHI P, et al. A comprehensive insight into bacterial virulence in drinking water using 454 pyrosequencing and Illumina high-throughput sequencing[J]. Ecotoxicology and Environmental Safety, 2014, 109: 15. DOI:10.1016/j.ecoenv.2014.07.029
[27] CHEN H, CHEN R, JING L, et al. A metagenomic analysis framework for characterization of antibiotic resistomes in river environment: application to an urban river in Beijing[J]. Environmental Pollution, 2019, 245: 398. DOI:10.1016/j.envpol.2018.11.024
[28] 任红星. 饮用水给水系统中微生物群落时空分布及其动态变化规律研究[D]. 杭州: 浙江大学, 2016
REN Hongxing. The spatial-temporal distribution and dynamic change of microbial community in drinking water supply system[D]. Hangzhou: Zhejiang University, 2016
[29] PENG H, SHAO Y, ZHANG Y, et al. Optimization of ultrasonic parameters for effective detachment of biofilm cells in an actual drinking water distribution system[J]. Journal of Zhejiang University: Science A, 2020, 21(3): 167. DOI:10.1631/jzus.A1900416
[30] 王若玮. 淋浴软管滞留水微生物再生长影响因素及其溯源研究[D]. 长沙: 湖南大学, 2019
WANG Ruowei. Study on the influencing factors of microbial regrowth in the retention water of shower hose and its source[D]. Changsha: Hunan University, 2019
[31] COX M P, PETERSON D A, BIGGS P J. SolexaQA: At-a-glance quality assessment of Illumina second-generation sequencing data[J]. BMC Bioinformatics, 2010, 11(1): 485. DOI:10.1186/1471-2105-11-485
[32] MEYER F, PAARMANN D, D'SOUZA M, et al. The metagenomics RAST server: a public resource for the automatic phylogenetic and functional analysis of metagenomes[J]. BMC Bioinformatics, 2008, 9(1): 386. DOI:10.1186/1471-2105-9-386
[33] MA L, LI B, JIANG X T, et al. Catalogue of antibiotic resistome and host-tracking in drinking water deciphered by a large scale survey[J]. Microbiome, 2017, 5(1): 154. DOI:10.1186/s40168-017-0369-0
[34] ZHANG H, CHANG F, SHI P, et al. Antibiotic resistome alteration by different disinfection strategies in a full-scale drinking water treatment plant deciphered by metagenomic assembly[J]. Environmental Science and Technology, 2019, 53: 2141. DOI:10.1021/acs.est.8b05907
[35] WU Y, CUI E, ZUO Y, et al. Fate of antibiotic and metal resistance genes during two-phase anaerobic digestion of residue sludge revealed by metagenomic approach[J]. Environmental Science and Pollution Research, 2018, 25: 13956.
[36] LI A D, LI L G, ZHANG T. Exploring antibiotic resistance genes and metal resistance genes in plasmid metagenomes from wastewater treatment plants[J]. Frontiers in Microbiology, 2015, 6: 1025. DOI:10.3389/fmicb.2015.01025
[37] LIU G, TAO Y, ZHANG Y, et al. Hotspots for selected metal elements and microbes accumulation and the corresponding water quality deterioration potential in an unchlorinated drinking water distribution system[J]. Water Research, 2017, 124: 435. DOI:10.1016/j.watres.2017.08.002
[38] COWLE M W, WEBSTER G, BABATUNDE A O, et al. Impact of flow hydrodynamics and pipe material properties on biofilm development within drinking water systems[J]. Environmental Technology (United Kingdom), 2019. DOI:10.1080/09593330.2019.1619844
[39] KEIN?NEN-TOIVOLA M M, REVETTA R P, SANTO-DOMINGO J W. Identification of active bacterial communities in a model drinking water biofilm system using 16S rRNA-based clone libraries[J]. FEMS Microbiology Letters, 2006, 257(2): 182. DOI:10.1111/j.1574-6968.2006.00167.x
[40] ZHANG S, HE Z, MENG F. Floc-size effects of the pathogenic bacteria in a membrane bioreactor plant[J]. Environment International, 2019, 127: 645. DOI:10.1016/j.envint.2019.04.002
[41] AGGARWAL S, GOMEZ-SMITH C K, JEON Y, et al. Effects of chloramine and coupon material on biofilm abundance and community composition in bench-scale simulated water distribution systems and comparison with full-scale water mains[J]. Environmental Science and Technology, 2018, 52(22): 13077. DOI:10.1021/acs.est.8b02607
[42] SILHAN J, CORFITZEN C B, ALBRECHTSEN H J. Effect of temperature and pipe material on biofilm formation and survival of Escherichia coli in used dinking water pipes: a laboratory-based study[J]. Water Science and Technology, 2006, 54(3): 49. DOI:10.2166/wst.2006.447
[43] BERRY D, XI C, RASKIN L. Microbial ecology of drinking water distribution systems[J]. Current Opinion in Biotechnology, 2006, 17(3): 297. DOI:10.1016/j.copbio.2006.05.007
[44] ZHU Y, WANG H, LI X, et al. Characterization of biofilm and corrosion of cast iron pipes in drinking water distribution system with UV/Cl2 disinfection[J]. Water Research, 2014, 60: 174. DOI:10.1016/j.watres.2014.04.035
[45] CORNELIS P, ANDREWS S C. Iron uptake and homeostasis in microorganisms[M]. [S. l. ]: Caister Academic Press, 2010
[46] WANG H, MASTERS S, FALKINHAM J O, et al. Distribution system water quality affects responses of opportunistic pathogen gene markers in household water heaters[J]. Environmental Science and Technology, 2015, 49(14): 8416. DOI:10.1021/acs.est.5b01538
[47] WHO. Global priority list of antibiotic-resistant batceria to guide research, discovery, and development of new antibiotics[R]. World Health Organization, 2017: 27