To realize solar-to-electrical energy conversion, several kinds of solar technologies have been developed since the invention of silicon solar cells. Recently, antimony selenosulfide Sb2(S,Se)3 (including Sb2S3 and Sb2Se3) has been considered one of the promising emerging materials for solar cell applications due to simple chemical composition, abundant elemental storage and excellent stability[1]. In terms of practical applications, a solar technology should simultaneously possess high-efficiency, low-cost and long-term stability. The stability for these materials and related devices has already been examined[2-4]. In particular, Sb2Se3 solar cells have passed through the stringent stability test[4]. Furthermore, the compound is non-toxic and doesn’t contain rare elements, which sets the ground for future low-cost production. The optical bandgap for antimony selenosulfide lies in 1.1–1.7 eV[1], falling into the optimal region for sunlight absorption. According to the Shockley-Queisser limit, ~32% PCE can be expected for Sb2(S,Se)3 solar cells.
In practical, one of the basic requirements for making efficient solar cells is to prepare high-quality absorber films. Recently, Tang et al. reported a hydrothermal deposition method (Figs. 1(a) and 1(b)), which produced flat and compact Sb2(S,Se)3 films with large grains[5]. The increase of selenium content in the compound can suppress the deep-level defects of anti-site SbS, while not yielding new defect SbSe. This characteristic suggests an effective strategy for suppressing the deep-level defects. At suitable Se/(S+Se) atomic ratio, Sb2(S,Se)3 solar cells can break the 10% bottleneck efficiency[5, 6], which is recorded in the record-efficiency cells table collected by AMOLF (Fig. 1(c), SbSSe stands for Sb2(S,Se)3 solar cells).

class="figure_img" id="Figure1"/>
Download
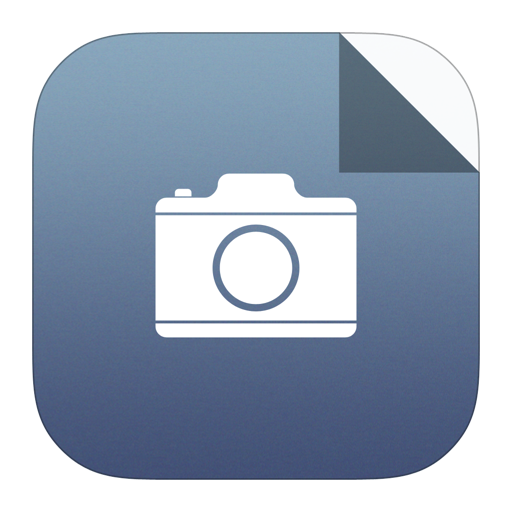
Larger image
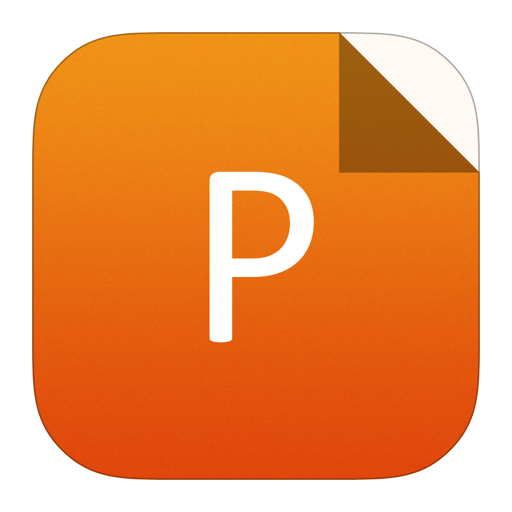
PowerPoint slide
Figure1.
(Color online) (a) Crystal structure of Sb2S(e)3. (b) Schematic for the hydrothermal deposition of Sb2(S,Se)3 in an autoclave, Copyright 2020, Springer Nature[5]. (c) Shockley-Queisser limit (black line) achieved by record-efficiency cells, grey lines showing 75% and 50% of the limit, Copyright 2020, https://www.lmpv.nl/sq/. (d) Two-step formation process of self-trapped excitons (STEs) in Sb2S3: hole is self-trapped first and then electron is captured by trapped hole to form STE, Copyright 2019, Springer Nature[10]. (e) Schematic diagram for the carrier transport near the dislocations along (001) orientation of Sb2Se3, Copyright 2021, John Wiley and Sons[11]. (f) EDX line scan for sample Sb2Se3/CdS (Sb2Se3 from CSS approach), Copyright 2020, American Chemical Society[12].
Because of the unique quasi-one-dimensional (Q1D) structure, the suitably oriented crystal is critical for efficient charge transport, which is quite different from the three-dimensional (3D) absorbers. In this case, vapor deposition methods, like rapid thermal evaporation (RTE), vapor transport deposition (VTD), closed-space sublimation (CSS), show strong ability to tune the crystal orientation[4, 7-9]. The high-temperature and low-pressure reactive system enables the fast grain growth, thus kinetically facilitating tilted (Sb4S(e)6)n ribbons on the substrate. However, it was observed that although the favorable orientation was obtained in high percentage, the efficiency did not show great improvement. This causes new concerns regarding the efficiency improvement in this class of solar cells[10-12]. Further investigations on the materials and device characteristics generate new understanding on fundamental issues associated mainly with the microstructures of antimony selenosulfide.
It is generally accepted that the Voc loss in antimony selenosulfide solar cells is one of the critical factors impeding the efficiency improvement. This deficit is usually ascribed to the defects which lead to increased recombination and dark current[13]. Nonetheless, Yang et al. recently demonstrated that the intrinsically self-trapping in Sb2S3 film is primarily responsible for this substantial energy loss[10]. The spectroscopic study of excited-state carrier properties suggests that the lattice deformation in this film gives rise to self-trapped excitons and thus transient defect state in bandgap (Fig. 1(d)). With this energy loss, the maximum Voc is limited to ~0.8 V for Sb2S3 solar cells, which matches the obtained top Voc of 0.8 V in Ref. [14]. This finding pointed out that the suppression of self-trapping effect is able to essentially increase the device efficiency. Plausibly, further investigating similar phenomenon in the alloy-type Sb2(S,Se)3 and pure selenide Sb2Se3 would be much interesting and significant for understanding the basic properties of this class Q1D materials and efficiency improvement.
The Q1D structure was also found to cause microstructure dislocations. Recently, Li et al. demonstrated that the stress generated during fast grain growth in achieving preferred [hk1]-oriented Sb2Se3 film brings forth the dislocations (Fig. 1(e)). This structure characteristic results in low carrier mobility and carrier density even in films with favorable orientation, thus limiting the efficiency improvement[11]. According to the detailed measurement, the majority carrier mobility in Sb2S3 and Sb2Se3 exhibits orders of magnitude lower than that in Cu2ZnSnSe4 (CZTSe). Therefore, developing advanced process to delicately controlling over the crystal growth becomes essential for obtaining high-quality antimony chalcogenide absorber films.
To date, highly efficient Sb2(S,Se)3 solar cells are primarily obtained by using CdS as the electron-transporting material (ETM). Most recently, a few theoretical studies were conducted to analyze the interfacial properties and in turn provide resolved strategies[12, 15]. Williams et al. indicated that the unstable Sb2Se3/CdS interface inevitably led to Sb and Se diffusing into CdS layer, thus deteriorating the Sb2Se3 layer and forming interfacial voids (Fig. 1(f))[12]. As a consequence, substantial interfacial defects were generated. This phenomenon becomes particularly serious in films from high-temperature deposition process. Even in Sb2(S,Se)3 film made with low-temperature hydrothermal deposition, a simulation study showed that the interface recombination between CdS and Sb2(S,Se)3 still gave rise to large Voc loss. Optimistically, seeking ideal ETM/Sb2(S,Se)3 junction with favourable lattice coupling and band alignment is expected to improve efficiency[15].
In summary, the deposition method, the film quality, the grain size, and the defect density are critical factors determining the performance for Sb2(S,Se)3 solar cells. How to reduce the defects, to optimize ETM/Sb2(S,Se)3 interface, to increase charge mobility, to reduce Voc loss, thus enhancing the device efficiency, is the mission in developing this new solar technology.
Acknowledgements
This work was financially supported by the National Natural Science Foundation of China (22005293, U19A2092), the China Postdoctoral Science Foundation (BH2060000144), and the National Key Research and Development Program of China (2019YFA0405600). L. Ding thanks the National Key Research and Development Program of China (2017YFA0206600) and the National Natural Science Foundation of China (51773045, 21772030, 51922032, 21961160720).