1.
Introduction
Because of the prediction of Landau, people are always uncertain of the existence of two-dimensional (2D) materials before graphene was exfoliated successfully[1]. Also for 2D ferromagnetic (FM) materials, the famous Mermin-Wagner theorem points out: Long-range magnetic order of isotropic spin-S Heisenberg model does not exist at nonzero temperature in dimensions d ≤ 2[2], which discourages many people who want to study 2D ferromagnets. However, people have not completely given up looking for 2D ferromagnets. After unremitting research, it has been found that magnetism can be indued in 2D systems by some external conditions, such as doping[3–5] and defects[6, 7]. Until 2017, 2D FM monolayer CrI3[8] and Cr2Ge2Te6[9] were successfully fabricated in experiments, which has attracted extensive attention. Massive researches on monolayer CrI3 and Cr2Ge2Te6 are beginning to emerge[10–17]. People have regained confidence and enthusiasm for the study of 2D ferromagnet. Recently, new 2D FM materials have been constantly predicted theoretically and synthesized experimentally, such as Fe3GeTe2[18], VSe2[19], MnSe2[20], Fe3P[21], VI3[22], and so on. The appearance of 2D FM materials provides a good platform for people to study physical properties of magnetic materials under 2D limit.
The appearance of van der Waals heterostructure (vdWH) engineering is accompanied by the in-depth study of 2D materials[23]. Just like building blocks[24], people can stack different materials to form heterostructures at will under the premise of lattice matching. The heterostructures usually show novel properties which are different from those of compositional materials and we can learn it from studying the charge transfer[17], exciton state[25–27], band alignment[17, 28, 29] and so on. With the emergence of 2D FM materials, we have more choices to construct heterostructures. We can’t help thinking that what novel properties would exhibit when a traditional non-magnetic material, such as TMDC, graphene, came into contact with a magnetic material. In fact, this topic is not fresh, and the magnetic and non-magnetic interface engineering, such as MoTe2/EuO[30], MoS2/EuS[31], WS2/MnO[32], has been studied before. However, due to the uncertainty of 2D magnet before, the problem was most studied in the mixed dimensional heterostructures (2D/3D) composed of 2D nonmagnetic materials and magnetic bulk materials.
In this review, we summarize recent researches on 2D FM materials and 2D FM vdWHs, including the intrinsic physical properties of 2D FM monolayers and 2D FM vdWHs, as well as the regulation of physical properties by some external conditions such as defect, doping, strain, and electric field. We also discuss the developing trend of 2D FM monolayers and vdWHs. We believe that it can help readers to further understand 2D FM materials.
2.
Research about 2D FM materials
2.1
Intrinsic physical properties of 2D FM materials
We first show the crystal model of CrI3 and Cr2Ge2Te6 in Fig. 1(a). It can be seen that the atomic structure of CrI3 is simpler, which means that it has higher symmetry. And Figs. 1(b) and 1(c) display the band structures of monolayer CrI3 and Cr2Ge2Te6, respectively, from which we can see that these two monolayers are both ferromagnetic semiconductors. Also, their Curie temperature (Tc) and magnetic anisotropy have been systematically studied[8, 9]. In addition, Zhong et al. find that the monolayer CrI3 possesses a giant magneto band-structure effect, i.e. the change of spin orientation can significantly modify the band structure of this material. Here, rotating the magnetic moment of CrI3 from out-of-plane to in-plane will cause a direct-to-indirect bandgap transition (Fig. 1(f)), which can result in a magnetic field controlled photoluminescence. They also find a significant change of Fermi surface with different magnetization directions, giving rise to giant anisotropic magnetoresistance. Moreover, the spin reorientation is found to modify the topological states[33]. Their work opens a new paradigm for spintronics applications. Xu et al. have studied phonon edge states and phonon Berry Phase with the monolayer CrI3 as a plateform through first-principles calculations. They find that the phonon Berry phase is quantized to ± π at two inequivalent valleys and the phonon edge states terminated at the projection of phonon Dirac cones (Figs. 1(d) and 1(e)). This work extend the knowledge of valley physics, providing wide applications of topological phonons[34].

class="figure_img" id="Figure1"/>
Download
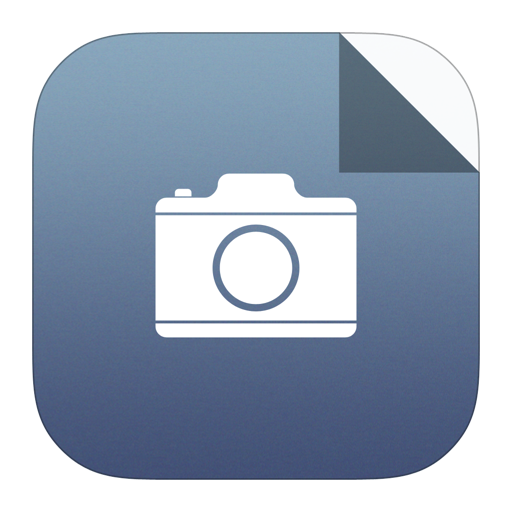
Larger image
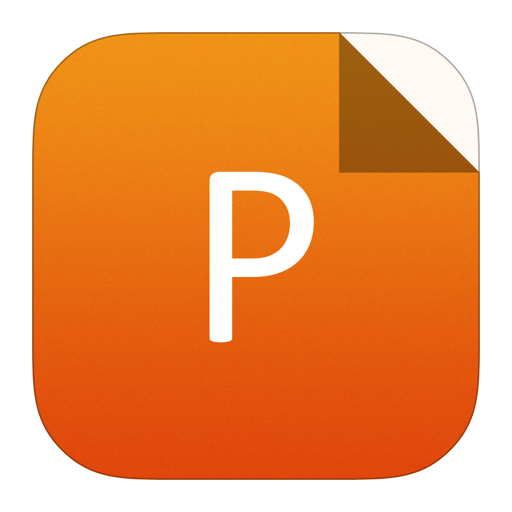
PowerPoint slide
Figure1.
(Color online) (a) The structure diagram of monolayer CrI3 (left) and monolayer Cr2Ge2Te6 (right). Reprinted with permission from Refs. [8, 9]. Copyright 2017, Springer Nature. The band structures and density of state (DOS) of (b) CrI3 and (c) Cr2Ge2Te6. Reprinted with permission from Ref. [17]. Copyright 2019, AIP Publishing. (d) The distributions of phonon Berry curvature near two inequivalent valleys K and K’, respectively, indicating that a phonon Dirac point is a singularity in momentum space. (e) The LDOS projected on edges of semi-infinite nanoribbons of (top) CrI3 and (down) YGaI along the zigzag direction. The nontrivial edge states terminated at the projection of phonon Dirac cones are clearly visible. Reprinted with permission from Ref. [34]. Copyright 2018, American Chemical Society (f) The band structure with magnetic moment along the out-of-plane c axis (left) and in-plane a axis (right). The red arrows depict the band gap that is direct with M//c and indirect with M//a. The insets show the side view and top view of the crystal structure with spin orientation along the c axis and a axis, respectively. Reprinted with permission from Ref. [33]. Copyright 2018, American Chemical Society.
2.2
Regulation of physical properties of 2D FM materials
In addition to study the intrinsic physical properties of two-dimensional ferromagnet, it is also very important to study the effects of external conditions such as the defect[35], charge doping[14, 36], electric field[13, 17, 37] and strain[17, 38] on its physical properties, such as electronic structure, magnetic anisotropy, Tc and so on. Wang et al. find that the I vacancies on the surface of monolayer CrI3 can not only can enhance the intrinsic ferromagnetism of monolayer CrI3 but also induce switchable out-of-plane electric polarization (Fig. 2(a)), and the I vacancies do not break the semiconducting nature of CrI3. In addition, they also find that the polarization direction can be reversed by switching the position of I vacancices and this method is also applicable to many other metal trihalides[35]. This work provides a new way for people to realize and regulate the electric polarization in low-dimensional systems, which is meaningful for engineering the multifunctional nanodevices. According to the study of Wu et al., now we have already known that electron or hole doping can transform CrI3 from semiconductor to metal, and improve the stability of its FM ground state[36], see Figs. 2(b) and 2(c). In addition, the electronic structure and the magnetism regulation of the 2D ferromagnet can also be realized by the strain engineering and the external electric field[17], see Figs. 2(d) and 2(e). For example, Gong et al. found that by applying a vertical electric field, the bilayer VS2 can convert from A type antiferromagnet to half metal. Fig. 2(f) shows the side view of bilayer VS2. The regulation of the electric field on the energy levels shows the opposite trends in different spin channels, leading to the gradual decrease of the gap of spin-α states and the opening of the gap of the spin-β states[37]. When the electric field reaches a certain value, the band gap of one spin state is zero, while the other spin state is still an insulated state. Thus, the 100% spin polarization current will be generated in bilayer VS2, see Fig. 2(g). Their research is very meaningful for designing the spin field effect transistor. What’s more, Wu et al. also point out that the energy difference between FM state and AFM state directly corresponds to the Tc[36]. From this we can conclude that the above methods can certainly change the Tc of the material. And in experiment, Zhang et al. have proved that the Tc of Fe3GeTe2 can be increased to room temperature by electric field[18]. In addition, the regulation of 2D ferromagnetism by external electric field[13] and electrostatic doping[14] has been realized experimentally.

class="figure_img" id="Figure2"/>
Download
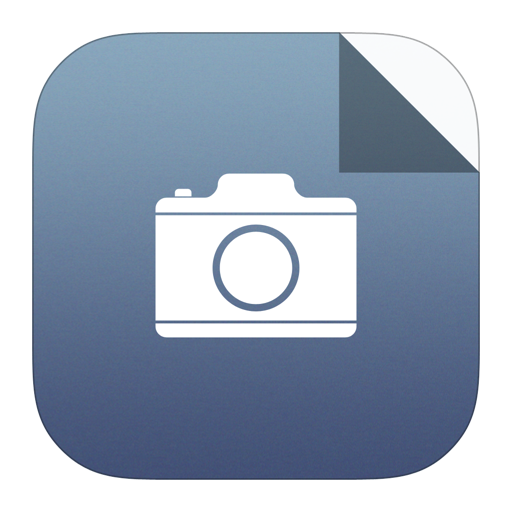
Larger image
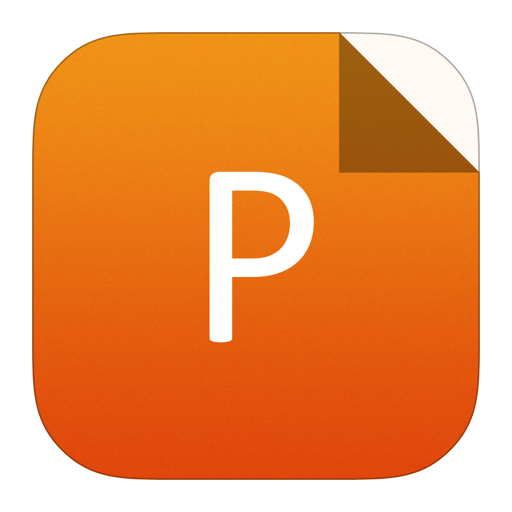
PowerPoint slide
Figure2.
(Color online) (a) The I-vacancies models of monolayer CrI3. Reprinted with permission from Ref. [35]. Copyright 2018, American Chemical Society. (b) The DOS of CrI3 monolayer doped with 0.5 hole or 0.5 electron, and (c) Relationship between ferromagnetic stability and carrier doping concentration. The dashed vertical lines in (b) refer to the shifting Fermi level[36]. (d) The strain engineering of monolayer Cr2Ge2Te6 shows that the bandgaps vary with the increase of biaxial strain for the FM state (the red line), and the black line represents the variety of total energy difference between the AFM and FM configurations with the strain in the monolayer Cr2Ge2Te6. (e) The dependence of PBE and HSE06 bandgaps under a perpendicular electric field with different field strengths. Reprinted with permission from Ref. [17]. Copyright 2019, AIP Publishing. (f) The side view of the bilayer 2H-VSe2, with the electric field applied perpendicularly from layer 2 to layer 1. (g) The schematic spin-polarized current versus the gate voltage, with Vc indicating the critical voltage. The switching of the spin-ɑ current Iɑ and spin-β current Iβ can be manipulated by the gate voltage. Reprinted with permission from Ref. [37]. Copyright 2017, National Academy of Sciences.
Through these studies, we can see that FM materials under 2D limit possess unusual properties in many aspects. I believe this will attract more and more attention, and there will be more groundbreaking and meaningful results in the future.
2.3
More 2D FM materials with higher Tc
Compared with the past, people simply obtained a kind of new material or a class of new materials by atomic substitution, but now people have begun to search for new 2D materials quickly and systematically using high-throughput calculation methods[39–41] such as structure search, machine learning and the like. Moreover, we can further screen 2D FM materials with excellent performance from the database through formula screening, geometry screening, manual screening and DFT calculations (Fig. 3(a)).

class="figure_img" id="Figure3"/>
Download
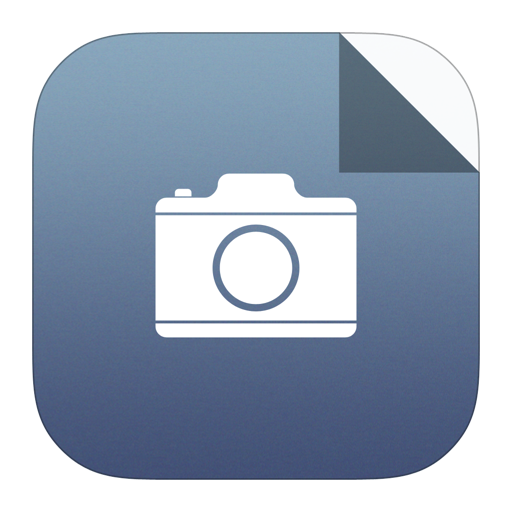
Larger image
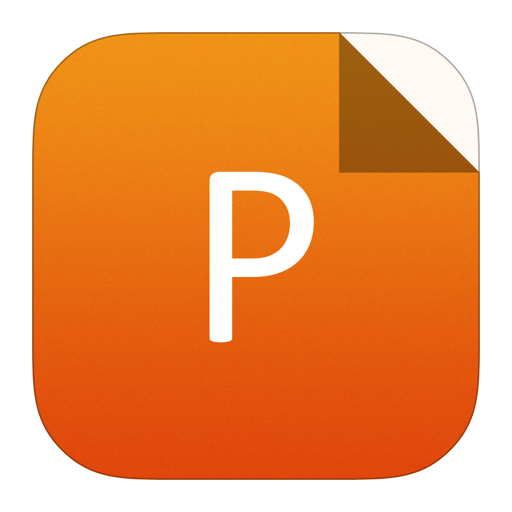
PowerPoint slide
Figure3.
(Color online) (a) A schematic diagram to illustrate the search procedure for 2D FM materials. Reprinted with permission from Ref. [41]. Copyright 2018, American Physical Society. (b) A schematic diagram to obtain the 2D intrinsic ferromagnet from the van der waals antiferromagnetic bulk. Reprinted with permission from Ref. [42]. Copyright 2018, American Chemical Society. (c) the monolayer VI3 be exfoliated from the bulk VI3. Reprinted with permission from Ref. [22]. Copyright 2019, American Chemical Society.
At present, people have gradually established a 2D material database[39], and are still trying to discover new 2D ferromagnets from theory and experiment. As shown in Fig. 3(b), Sun et al. theoretically find a class of new 2D ferromagnets, CrOCl and CrOBr monolayers, which can be obtained by exfoliating from the layered AFM bulk materials[42]. Also, Lei et al. successfully synthesize vdW magnetic compound VI3 in experiment, and theoretically predict that the monolayer VI3 possesses the ferromagnetic-insulator property and are feasible to be exfoliated from the bulk[22] (Fig. 3(c)). It can be seen that the combination of theoretical prediction and experimental verification will greatly accelerate the research process of 2D FM materials, and there will be more and more 2D FM materials be made in the future.
Although there are many 2D FM materials predicted by theory, very few have been successfully prepared in experiments, and most of the Tc are much lower than room temperature, such as monolayer CrI3 (Tc = 45 K)[8], Cr2Ge2Te6 (Tc = 28 K)[9], Fe3GeTe2 (Tc ≈ 19 K)[18], which will limit the application of these materials in pragmatic spintronic devices. Therefore, it is urgent to find 2D FM materials with the higher Tc and the method of raising the Tc. The Journal of Science has published 125 of the most important issues, one of which is whether it is possible to produce a room-temperature FM semiconductor[43]. Recently, some research progress has been made in these areas.
In theory, Zhao et al. systematically screen out five high temperature 2D FM materials (LaCl, YCl, ScCl, LaBr2, and CrSBr with Tc > 200 K) using the first-principle calculation methods[44], see Fig. 4(a). Kan et al. propose a method to raise the Tc. As shown in Fig. 4(b), they found that in double-orbital model, the ferromagnetism of the material is closely related to the virtual exchange gap. Thus, we can reduce the exchange gap by equivalent alloying the material, which can greatly enhance the FM coupling. They also validated the proposed theory with CrI3, CrGeTe3 and so on, and the results show that their Tc is increased by 3–5 times by alloying[45]. In addition, Yang et al. also raise a method to achieve high Tc. They design a special organometallic frameworks connected by antiaromatic rings, which can enhance the Tc of 2D FM semiconductors much higher than room temperature[46], see Figs. 4(c)–4(e).

class="figure_img" id="Figure4"/>
Download
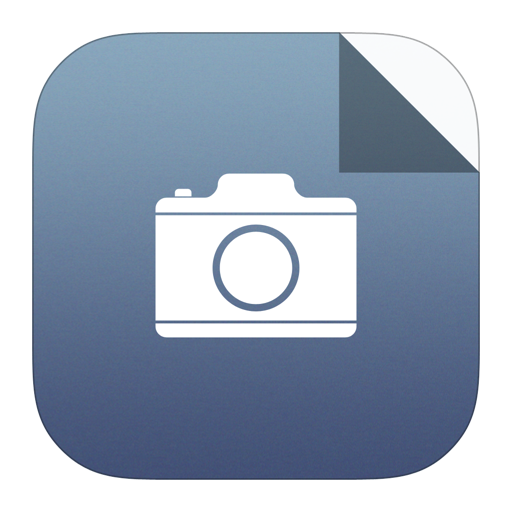
Larger image
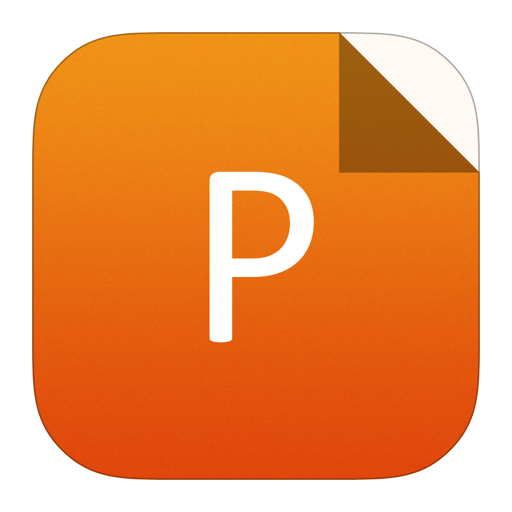
PowerPoint slide
Figure4.
(Color online) (a) The diagram of Tc and structural models of several high temperature FM materials. Reprinted with permission from Ref. [44]. Copyright 2018, American Chemical Society. (b) the mechanism of superexchange in two semiconducting alloy compounds CrWI6 and CrWGe2Te6 monolayers. The insert is the enhanced Tc of CrWI6 and CrWGe2Te6 compared to 2D CrI3 and CrGeTe3. Reprinted with permission from Ref. [45]. Copyright 2018, American Chemical Society. (c) The structural model of metal organic framework. (d) Spin density of ferrimagnetic (top) and FM (bottom) coupling for 2D Cr-pentalene with an isovalue of 0.05 e/?3. Red and blue indicate spin up and spin down, respectively. (e) Variation of magnetic moment (M) per unit cell (black) and specific heat (CV) (red) with respect to temperature from classic Heisenberg model Monte Carlo simulation. Reprinted with permission from Ref. [46]. Copyright 2018, American Chemical Society.
In experiments, it has been mentioned that different research groups have tried to increase TC of materials through some external conditions. At the same time, people are trying to explore new 2D intrinsic ferromagnetic materials with room temperature. For example, VSe2 and MnSe2 monolayers have been successfully obtained in experiments, and the results show that they have high Tc with 330 K for VSe2 and 300 K for MnSe2[19, 20].
3.
2D FM vdWHs
Not only 2D ferromagnets themselves have many novel physical properties, but also many novel physical phenomena will appear when they are stacked with other nonmagnetic 2D materials, such as proximity induced spin polarization[47, 48], valley polarization[49, 50], and topological properties[51, 52]. Now, more and more people have started to study 2D FM vdWHs. Very recently, Zhang et al. have summarized several factors related to the interface engineering of magnetic heterostructures in a review[53]. So we will not describe it here. In this section, we focus on the theoretical works on 2D FM vdWHs, hoping to help readers further understand this direction.
3.1
Spin-polarized band structures
When the ferromagnetic materials and non-magnetic materials are stacked forming the vdWHs, due to the spin-polarized electronic structure of magnetic materials, the heterostructures will also possess different electronic states in different spin channels. As shown in Fig. 5(a), the Mg(OH)2/VS2 vdWH possess the spin-polarized band structure with the conduction-band maximum (CBM) are located at K and M point in the spin-up channel and spin-down channel, respectively. Obviously, the band gaps of different spin channels are different with 0.14 eV for spin up and 0.57 eV for spin down. Moreover, it can also be seen from the band alignment that there are different band offsets in different spin channels, see Fig. 5(b), which means that there are different separation possibilities for electrons and holes in spin-up and spin-down channels. In addition, Xiong et al. also found that the external electric field can induce the different band-alignment transition in different spin channels[47] (Fig. 5(c)). The characteristic of multiple-band alignments induced by electric field potentially prompts a new type of multifunctional spintronic device.

class="figure_img" id="Figure5"/>
Download
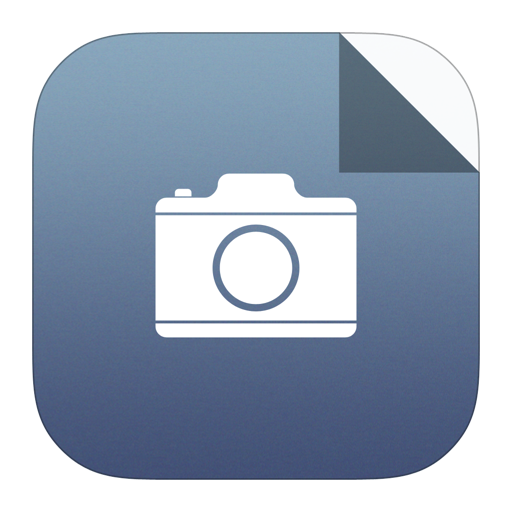
Larger image
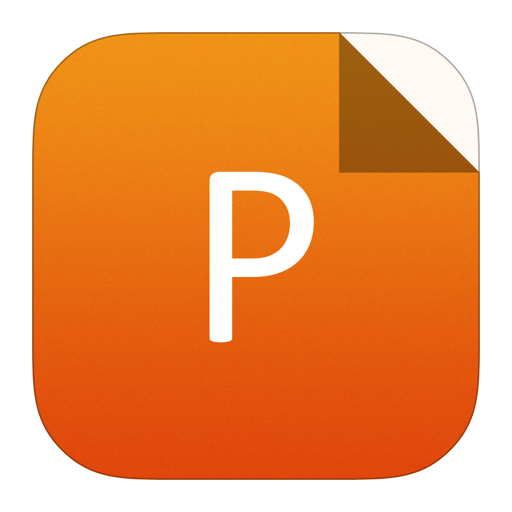
PowerPoint slide
Figure5.
(Color online) (a) The spin-polarized band structure of Mg(OH)2/VS2 heterostructure. (b) The band alignment and work function of the heterostructure, referring to the vacuum level (Evacuum). (c) Band alignments of Mg(OH)2/VS2 heterostructure at various electric field values: –0.3, 0, 0.6, and 0.7 V/?, respectively, referring to the Evacuum. Reprinted with permission from Ref. [47]. Copyright 2017, American Physical Society.
3.2
Valley polarization and quantum anomalous Hall effect
More and more attention has been paid for the valley-physics properties of graphene and monolayer transition metal dichalcogenides (TMDC) MX2 (M = Mo, W; X = S, Se, Te). Compared with graphene, the monolayer MX2 have stronger spin orbit coupling (SOC) effect, which leads to obvious energy level splitting at K and K’ valleys, and these two valleys are energetically degenerate because of the time-reversal symmetry. Also, the broken inversion symmetry features two inequivalent valleys with different angular momenta, as shown in Fig. 6(a). Based on the above factors, we can implement the valley hall effect in monolayer MX2 where carriers in different valleys flow to opposite transverse edges when applying an in-plane electric field[54]. In addition, when we somehow produce a non-zero net magnetic moment in this material, the valley degeneracy can be lifted (Fig. 6(b)), which we call the valley polarization. At present, several methods are often used to realize valley polarization, such as optical pumping[55], external magnetic field[56], doping magnetic atoms[57], stacking on magnetic materials[30–32]. Among them, constructing FM vdWHs is a very promising method because it is more stable and easier to regulate.

class="figure_img" id="Figure6"/>
Download
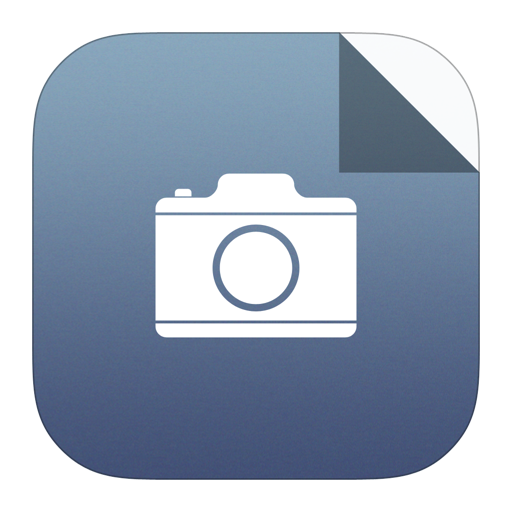
Larger image
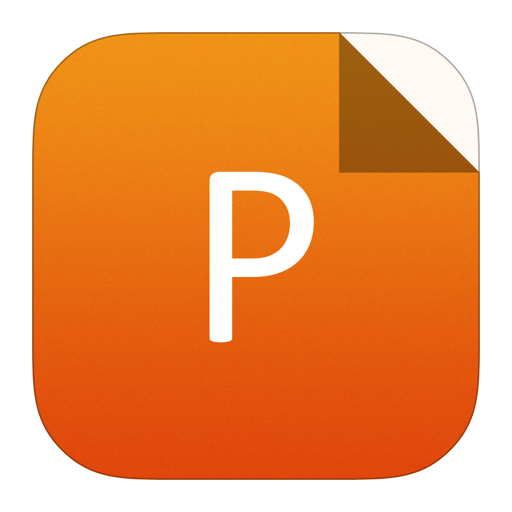
PowerPoint slide
Figure6.
(Color online) (a) The energy diagram of the monolayer WSe2 at the K, K’ valleys. E(σ+) and E(σ–) represent the interband optical transition energies of right-hand (σ+) and left-hand (σ–) circularly polarized photons, respectively. The spin-up and spin-down valley-spin states are denoted with orange up- and green down-arrows, respectively. (b) Energy diagram depicting the K and K’ valley degeneracy lifting. The VB and CB stand for the valence and conduction band valley splittings, respectively. The black-up arrow denotes the Cr spin is aligned vertically upward, i.e., the magnetization axis of the CrI3. Reprinted with permission from Ref. [49]. Copyright 2019, American Physical Society. (c) projection bands of graphene around ±K with SOC under the electric field. (d) schematics illustration of change of valley splitting at ±K under the electric field. Reprinted with permission from Ref. [51]. Copyright 2019, Springer Nature. (e) The calculated edge density of states of the semi-infinite armchair-edged graphene system. (f) A schematic diagram depicting the observation of the QAH effect in graphene/CrI3 heterobilayer. The vertical red arrow denotes the external compression. The small horizontal yellow arrows indicate the two dissipationless edge current channels owned in the heterostructure. Reprinted with permission from Ref. [51]. Copyright 2018, American Physical Society.
With the emergence of 2D FM materials, people began to study the valley polarization in 2D/2D systems. Recently, Liu et al. have studied the effect of stacking mode on valley polarization in WSe2/CrI3 vdWH[49], and Farooq et al. found that the valley polarization also exists in graphene/CrI3 and bilayer graphene/CrI3 vdWHs, and the valley-polarization characteristics can be regulated by electric field[50], see Figs. 6(c) and 6(d). In addition, it is also in graphene/CrI3 vdWH, a Chern insulating state can be acquired if the interlayer distance is compressed to a distance between about 3.3 and 2.4 ?, and the quantum anomalous Hall effect (QAHE) is promising to be observed[51] (Figs. 6(e) and 6(f)). Very recently, the high-temperature QAHE also has been predicted existing in 2D germanene/Cr2Ge2Te6 and germanene/CrI3 vdWHs[52]. These results show that there are still many physics waiting for us to discover in 2D FM vdWH systems. In addition, they also point out the direction for the experimental study of 2D FM vdWHs.
We also note that a new type of multiferroic vdWHs has recently been proposed[58, 59]. Multiferroic means that one material has two or more properties of ferroelectricity, ferromagnetism and ferroelasticity at the same time. However, very few intrinsic multiferroic materials have been found, which limits the research of this direction and the development of multifunctional nano-electronic devices. Now, we can simply stack a 2D FM material with a 2D ferroelectric material to form a multiferroic heterostructure, which may provide a new way for people to achieve multiferroic in experiments.
4.
Conclusion
In conclusion, we summarize the recent theoretical advances on intrinsic 2D FM materials and vdWHs, including the intrinsic electronic structure, magnetism, Tc, valley polarization, band alignment and so on. These studies show that 2D FM materials and vdWHs possess many novel physical properties, and they are promising materials to be applied in spintronic and valleytronic nanodevices. Moreover, many methods, such as vacancy, doping, strain and external electrical field, can be used to regulate the electronic structure, magnetism, Tc, valley polarization, and band alignment of 2D FM materials and vdWHs, indicating the properties of 2D FM materials and vdWHs are highly tunable. However, although many 2D FM materials have been predicted in theory, very few of them have been successfully prepared in experiments. In addition, most Tc of 2D FM materials are much lower than room temperature, which limit the applications of these materials in practice. Therefore, it is necessary to find the 2D FM materials with the higher Tc or find the method of raising the Tc. In short, although we have made many breakthroughs in this field recently, there are still many problems waiting for us to find and solve.