1.
Introduction
Resonators are a fundamental requirement for wireless communication systems. Micromechanical silicon resonators are considered as potential substitutes for quartz crystal resonators due to their compact size, high performance, and CMOS-compatible fabrication process[1].
Frequency stability is crucial for a resonator to maintain reliable radio communication without signals drifting into nearby bands[2]. The MEMS resonators exhibit exceptional frequency stability[3–6], for instance, an encapsulated tuning fork resonator with the resonant frequency of 155 kHz has the frequency stability of 3.1 ppm over 330 days[3]. A vacuum-encapsulated length-extensional mode resonator of 13 MHz demonstrated ppm-level frequency stability during a period of 42 days[4]. A 27 MHz aluminum-nitride bulk acoustic wave resonator maintains a frequency fluctuation within 1.2 ppm over 50 days[5]. Additionally, a 12.1 MHz square-extensional mode resonator was measured over a month and the frequency drift is less than 0.1 ppm[6]. However, there are hardly any reports on the frequency stability of very high frequency (VHF) resonators. This work carries out a systematic study on the long-term frequency stability and temperature cycling for the 3D encapsulated disk resonator.
2.
MEMS resonator and package
The disk resonator used in this work was fabricated using the same process as Ref. [7], as shown in Fig. 1 for the SEM photograph. It consists of an 18 μm-radius polysilicon disk with the thickness of 2.5 μm suspended by a stem in the center, two polysilicon electrodes are separated from the disk by a gap of 70 nm[8]. To excite the resonator, a DC-bias voltage (VP) is applied to the disk and an AC driving voltage is applied to the input electrode. The resonator operates at radial-contour mode, which can achieve a high resonant frequency of 150 MHz and high quality factor (Q) close to 10 000 in air. The temperature coefficient of resonant frequency (TCf) for the disk resonators is experimentally acquired as ?24 ppm/°C, which depends on the temperature coefficient of Young’s modulus of polysilicon and the resonator geometry.

class="figure_img" id="Figure1"/>
Download
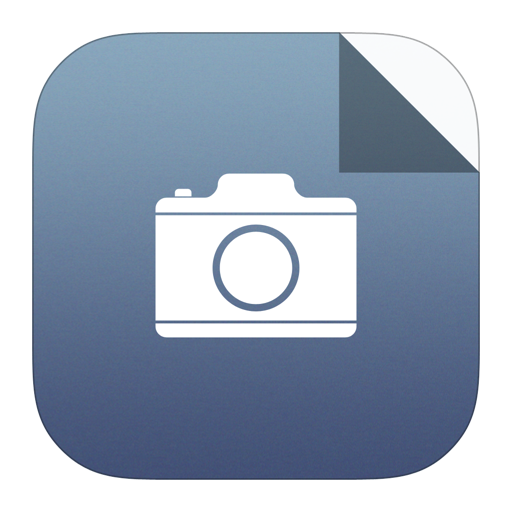
Larger image
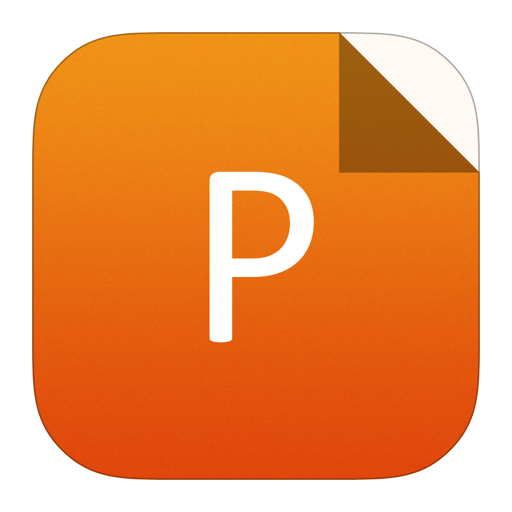
PowerPoint slide
Figure1.
SEM photographs for the radial-contour-mode resonator.
The vacuum packaging of the resonator was implemented using the 3D encapsulation technique[9], as shown in Fig. 2. The encapsulation cap is fabricated with a low resistivity silicon wafer and bonded with Pyrex 7740 to form the glass-in-silicon (GIS) structure, which has low capacitive feed-through and the minimal Q-factor degradation. Au–Sn solder bonding is employed to provide a vacuum encapsulation as well as electrical conductions. He leakage test indicates the good hermeticity of the 3D vacuum packaging technique[10].

class="figure_img" id="Figure2"/>
Download
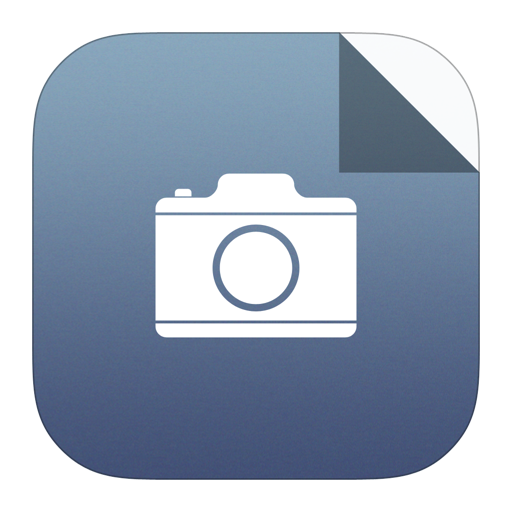
Larger image
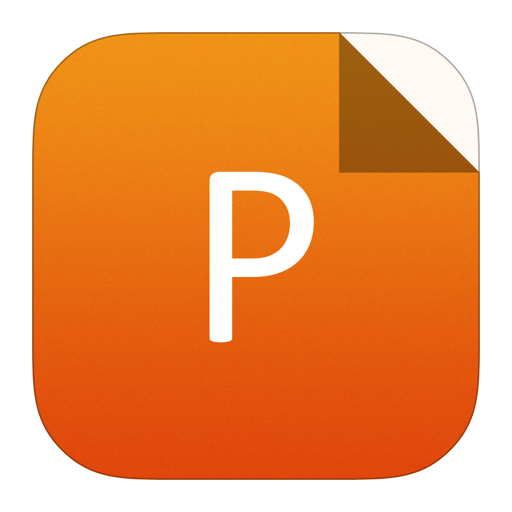
PowerPoint slide
Figure2.
(Color online) (a) Photograph of the packaged resonator using 3D packaging technique. (b) SEM cross-section photograph of the packaged resonator.
3.
Parasitic simulation and elimination
3.1
Parasitic effect and electrical model
The parasitic capacitance between the input and the output could severely deteriorate the performance of the VHF MEMS resonator. For the encapsulated resonator, the parasitic effect has been significantly suppressed by grounding the substrate and optimizing the package structure. However, when the resonator was connected with the electrical circuit, as shown in Fig. 3, an anti-resonance peak is introduced in the frequency spectrum, the signal-to-background ratio (SBR) is reduced from 25 to 17 dB and the Q-factor decreases from 9100 to 7400. The parasitic effect results from the parasitic capacitance in the PCB.

class="figure_img" id="Figure3"/>
Download
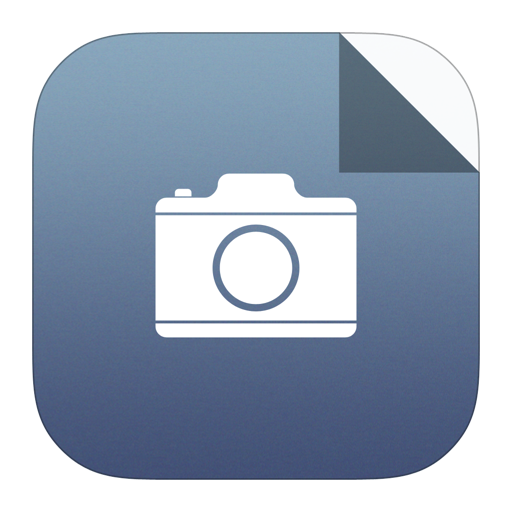
Larger image
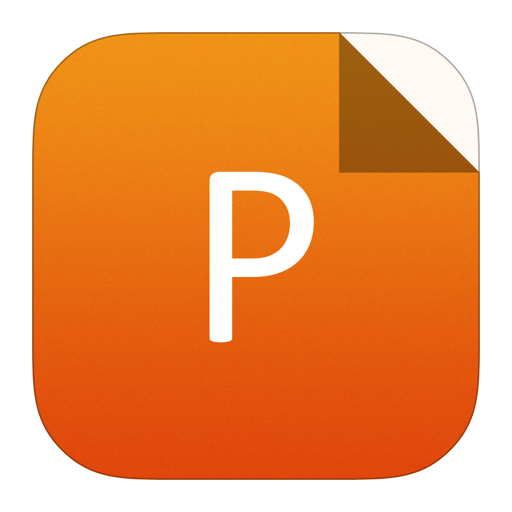
PowerPoint slide
Figure3.
(Color online) Frequency spectrum of the resonator measured with RF probe station and with PCB.
To analyze the parasitic effect, an equivalent circuit model of the packaged resonator connected with PCB is established, as shown in Fig. 4, it consists of a series RLC model, a transformer (?1 : 1), parasitic C1 and R1, feed through C2 and R2, and parasitic capacitance of PCB Cf. Substituting electrical parameters of the disk resonator listed in Table 1 into the equivalent circuit model, the value of Cf = 3 fF can be extracted by fitting the simulated and measured frequency spectra.
R0 (kΩ) | L0 (H) | C0 (fF) | R1 (pF) | C1 (MΩ) | R2 (MΩ) | C2 (fF) |
494.78 | 3.34 | 3.33 × 10?4 | 0.39 | 13.61 | 3.8 | 0.375 |
Table1.
Values of electrical parameters for the disk resonator.
Table options
-->

Download as CSV
R0 (kΩ) | L0 (H) | C0 (fF) | R1 (pF) | C1 (MΩ) | R2 (MΩ) | C2 (fF) |
494.78 | 3.34 | 3.33 × 10?4 | 0.39 | 13.61 | 3.8 | 0.375 |

class="figure_img" id="Figure4"/>
Download
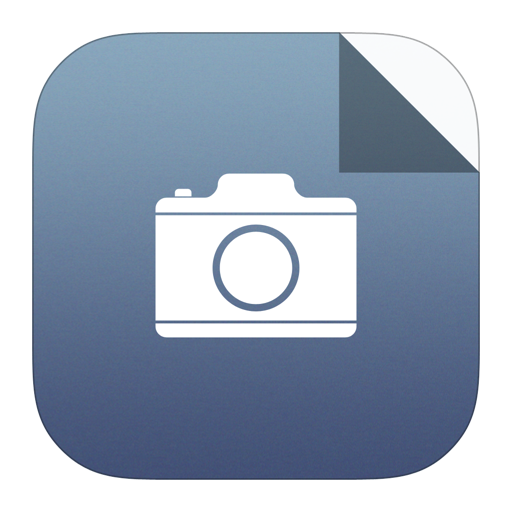
Larger image
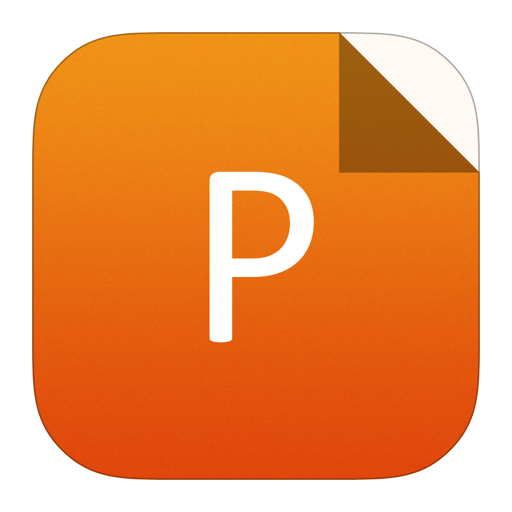
PowerPoint slide
Figure4.
Equivalent circuit model of the disk resonator connected with PCB.
3.2
Parasitic elimination
The parasitic effect was eliminated through a system-level circuit topology, as shown in Fig. 5. One output port of a differential amplifier is connected to the driving electrode of the resonator, and another output signal of the amplifier is in anti-phase with the resonator driving signal and sent to a tunable compensating capacitor Cneg. The large parasitic capacitor Cf is in parallel with the resonator, thus the parasitic effect can be completely eliminated with Cneg = Cf.

class="figure_img" id="Figure5"/>
Download
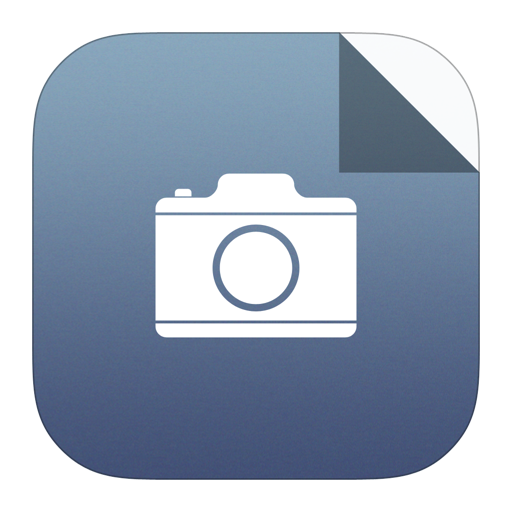
Larger image
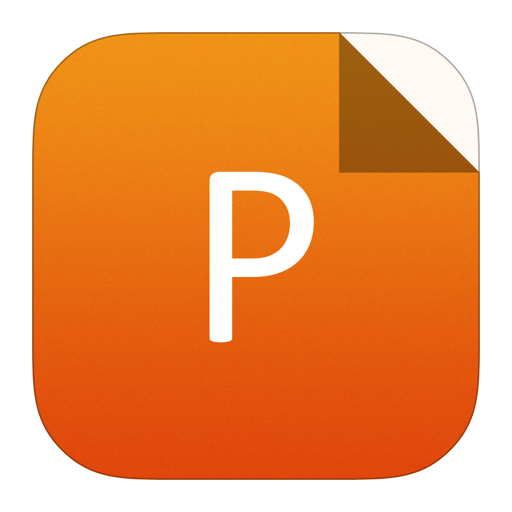
PowerPoint slide
Figure5.
(Color online) (a) Schematic of the negative capacitive compensation for parasitic elimination and (b) the PCB for parasitic elimination.
The measured frequency spectrum with and without the parasitic effect are shown in Fig. 6. There is no anti-resonance in the frequency spectrum with the parasitic signal eliminated, as a result, there are an increase in SBR by 8 dB and an increase in Q-factor by 2500. The negative capacitive compensation is simple and effective for parasitic elimination.

class="figure_img" id="Figure6"/>
Download
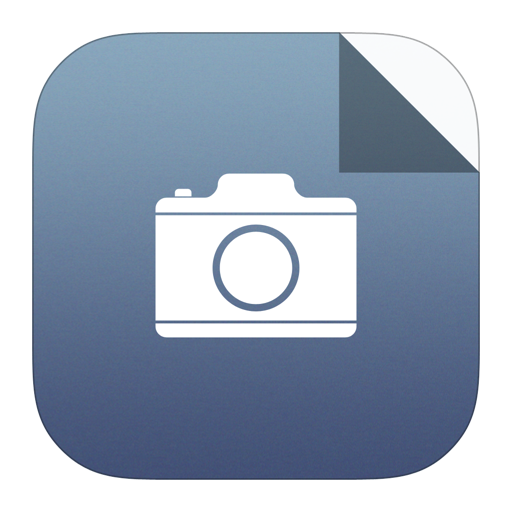
Larger image
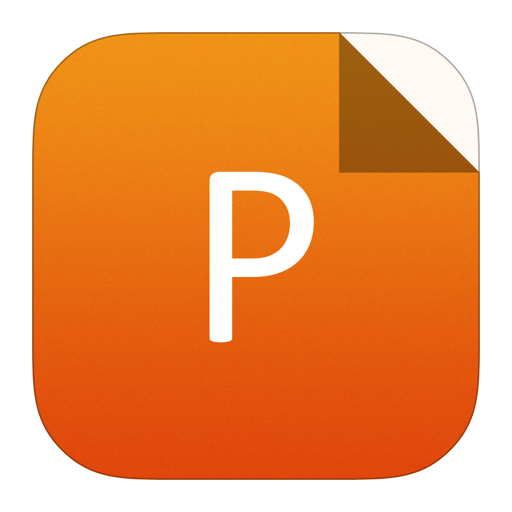
PowerPoint slide
Figure6.
(Color online) Measured frequency spectrum of the resonator with and without the parasitic effect.
4.
Experimental results and discussion
The stability test system based on the parasitic elimination method was established. The schematic of this system is shown in Fig. 7. An Agilent E5071C network analyzer was used to measure the resonant frequency and Q-factor of the device. A Labview program was developed to control the network analyzer for automatic data acquisition. To minimize temperature-related frequency variations, the testing PCB connected with the encapsulated resonator was installed in a temperature-controlled chamber with the temperature drifting within ±0.025 °C. The temperature inside the chamber was measured using a Pt100 sensor, which is in good thermal contact with the encapsulated resonator. The long-term frequency stability and temperature cycling test of the encapsulated resonator was investigated.

class="figure_img" id="Figure7"/>
Download
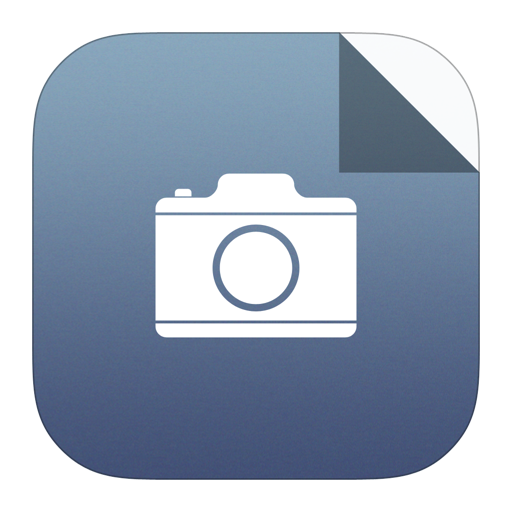
Larger image
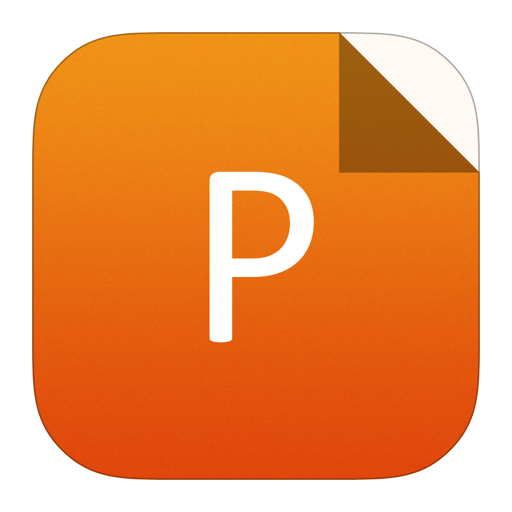
PowerPoint slide
Figure7.
(Color online) Schematic of the stability test system.
4.1
Long-term stability
The resonant frequency and Q-factor of the encapsulated resonator were monitored at 25 ± 0.025 °C for 240 h and measured every 30 s, as presented in Fig. 8. The resonant frequency variation was within ±1 ppm, much better than 10 ppm of the quartz crystal resonators in an initial burn-in period[11]. The initial burn-in is ascribed to the stress relaxation of the resonator. The small frequency drift indicates that the stress in the polysilicon thin film used as the structural layer of the resonator is very small.

class="figure_img" id="Figure8"/>
Download
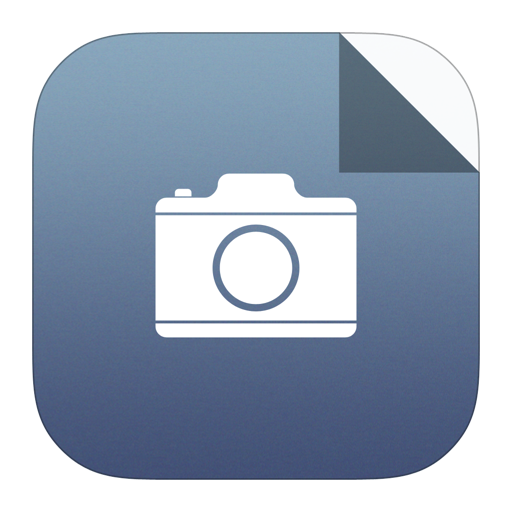
Larger image
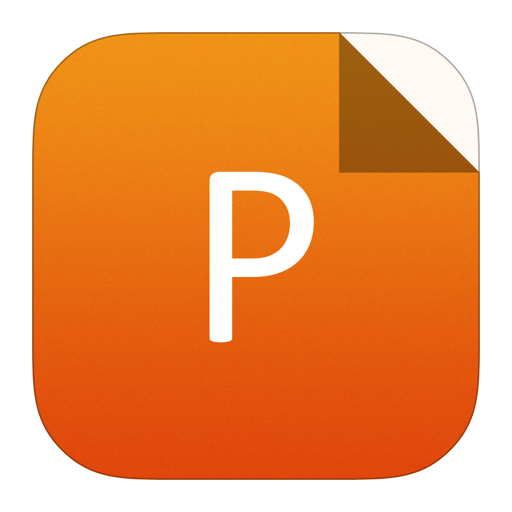
PowerPoint slide
Figure8.
(a) The measured resonant frequencies and (b) Q-factors for the encapsulated resonator during the long-term stability test.
For better understanding the performance of the resonator, Allan deviation was calculated from the long-term testing data by averaging the frequency difference between two adjacent sampling sections. Allan deviation is defined as[12]:
$${sigma ^2}_{{ m{Allan}}}left( tau ight) = sqrt {frac{1}{{2left( {N - 1} ight)}}sumlimits_k^{N - 1} {{{left( {overline {{y_{{{k}} + 1}}} ,,-,, overline {{y_{{k}}}} } ight)}^2}} } .$$ ![]() | (1) |
Fig. 9 presents the Allan deviation versus the averaging time for the resonator. The Allan deviation curve has a “U” shape, with a noise floor of 0.026 ppm, which indicates the maximum frequency stability of the resonator. This noise floor value is competitive with that of the conventional quartz resonators.

class="figure_img" id="Figure9"/>
Download
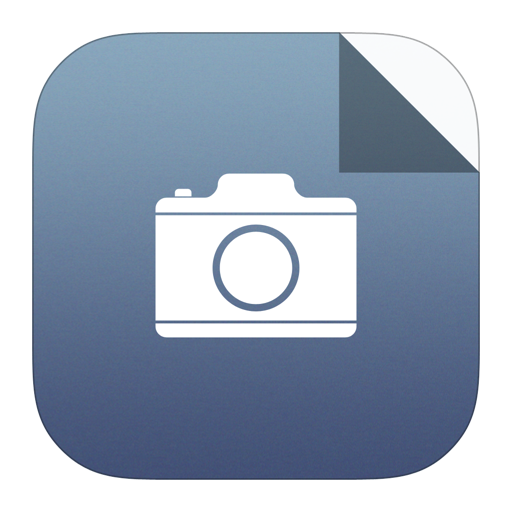
Larger image
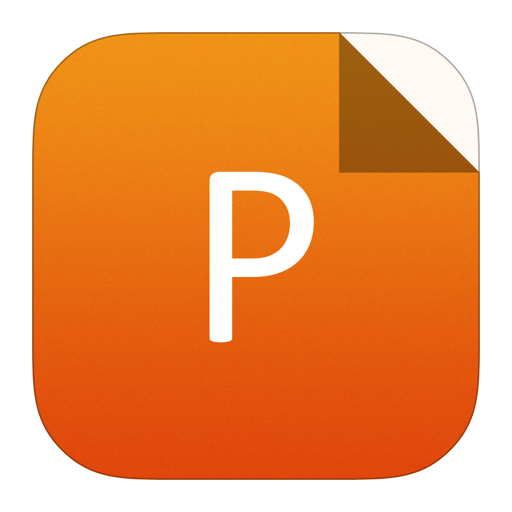
PowerPoint slide
Figure9.
Allan deviation calculated from long-term stability data with different averaging time.
4.2
Temperature cycle
When the encapsulated resonator experiences a rapid temperature change, the stability could be affected due to its residual stress and cracking in package. In this work, a thermal cycle test was done in order to understand the reliability of the 3D encapsulated resonators. Resonators are center anchored and could be immune to the thermal stress. In addition, the Sn-rich Au–Sn bonding employed for the 3D vacuum packaging of the resonator can produce large plastic deformation to release stress.
The resonant frequency and Q-factor of two resonators were measured every two cycles at 25 °C during 200 temperature cycles between ?40 and 85 °C. A 30 min interval was set between the consecutive temperature changes to reach thermal equilibrium. The measurement result is shown in Fig. 10. An overall frequency fluctuation of 1.5 ppm is obtained. Considering the TCf = ?24 ppm/°C, the frequency drift caused by temperature error is 1.2 ppm, indicating that the frequency fluctuation of 1.5 ppm is mainly caused by the temperature detection error, and the stress caused by the thermal expansion is effectively released and the packages maintain good hermeticity during a thermal cycle.

class="figure_img" id="Figure10"/>
Download
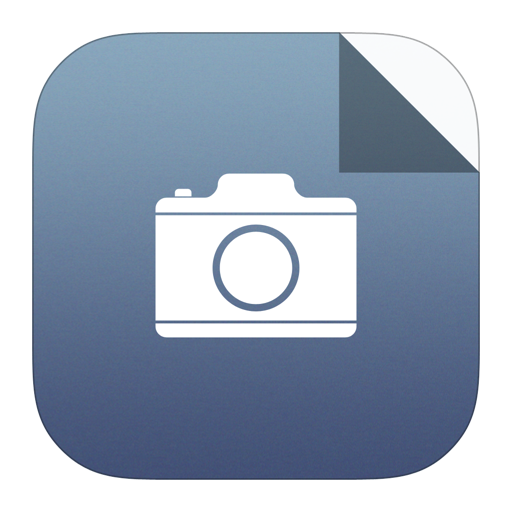
Larger image
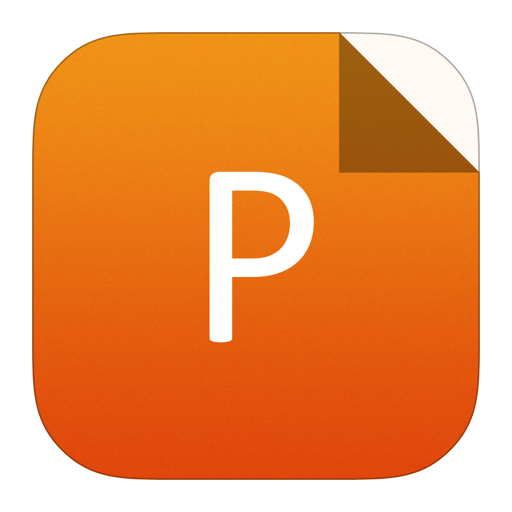
PowerPoint slide
Figure10.
(Color online) (a) The measured resonant frequencies and (b) Q-factors for the encapsulated resonators during the temperature cycling.
5.
Conclusions
The frequency stability of the 3D encapsulated disk resonator has been investigated. The resonant frequency variation during the long-term stability test is ±0.9 ppm and no distinguished initial aging was observed, which indicates that the stress in the structural layer of the resonator is very small. A frequency fluctuation of 1.5 ppm is obtained during the temperature cycling, which is mainly caused by temperature detection error. The 3D encapsulated disk resonators with the good frequency stability have potential applications in wireless communication systems in the future.