HTML
--> --> -->Early studies of WSHR focused on South China, with the definition developed according to the situation in that region. In recent years, WSHR events in other regions, such as southern China (25°?35°N, east of 110°E) and North China have been studied. A heavy rainfall event occurred in North China on 21 July 2012, which included warm-sector and frontal precipitation (Chen et al., 2012a; Zhong et al., 2015). As WSHR ahead of the cold front had not been predicted in numerical models, the total precipitation of this extreme torrential rainfall was much weaker than the actual rainfall (Chen et al., 2012a; Sun et al., 2012).
WSHR events often cause severe flooding, huge economic losses, and many casualties, but the operational prediction of these events is difficult and often inaccurate. It is therefore of great operational significance to further study WSHR events. To encourage more scientists to study this problem, the existing research and the challenges presented by WSHR are summarized in this paper. The definition, synoptic weather patterns, and trigger mechanisms of WSHR in South China are summarized in section 2. WSHR events in other regions of China (southern China and North China) are discussed in section 3. In section 4, a discussion of WSHR events and conclusions are provided.
2
2.1. Definition and objective identification of WSHR
Atkinson and Smithson (1972) found that precipitation was mainly located near warm fronts and in the warm sector within a certain distance of a warm front in England and Wales, but no specific definition of warm-sector rainfall was proposed. The high frequency of WSHR events in South China has the following features (Lin et al., 2006): (1) severe rainfall occurs under the background environmental conditions of high temperature, high humidity, and strong instability; (2) the rainfall is concentrated over several hours; (3) the rainfall has a smaller coverage than frontal heavy rainfall, with horizontal scales from dozens to hundreds of kilometers. Some previous studies have defined WSHR (Tao, 1980; Huang et al., 1986; Lin et al., 2006), but there are no objective criteria for identifying WSHR events.Liu et al. (2019) studied a 34-year dataset of heavy rainfall in South China and quantitatively defined the criteria for WSHR events: (1) No frontal systems appear in vertical cross sections, the rainfall areas are influenced by a southerly wind in the middle and lower troposphere, and the distance to the surface northerly wind is more than 200 km. (2) When frontal systems occur in the vertical cross sections, the distance between the precipitation and front is more than 200 km. According to these criteria, 177 WSHR events were identified objectively, which occurred from April to September during 1982 to 2015 (Fig. 1), which accounted for 16.86% of the selected severe heavy rainfall events. Most WSHR events occurred in the pre-rainy season (April to June), and the peak appeared in June, which was consistent with the results of previous studies (Huang et al., 1986; Ding et al., 2011; Chen et al., 2012a). It was also found that most of the WSHR events in April to June occurred in Guangdong Province, while events in July to September mainly occurred in Guangxi Province (Liu et al., 2019). There were fewer WSHR events in inland locations than in the eastern coastal areas (Fig. 2a). Four main WSHR precipitation areas were identified (Fig. 2a): the coastal area in Guangxi (centered on Qinzhou); the mountainous area in northern Guangdong (centered on Longmen); the coastal region in southern Guangdong (centered on Yangjiang and Enping); and the coastal area in eastern Guangdong (centered on Shanwei). In Guangxi Province, the frequency of WSHR events was lower and the precipitation was weaker in inland areas compared to the coast regions (Fig. 2b), with precipitation being stronger in individual coastal stations than in inland stations. In the inland regions, the precipitation in Guangdong was stronger than that in Guangxi, and the rainfall in coastal regions of Guangdong was the heaviest among the four main WSHR areas.
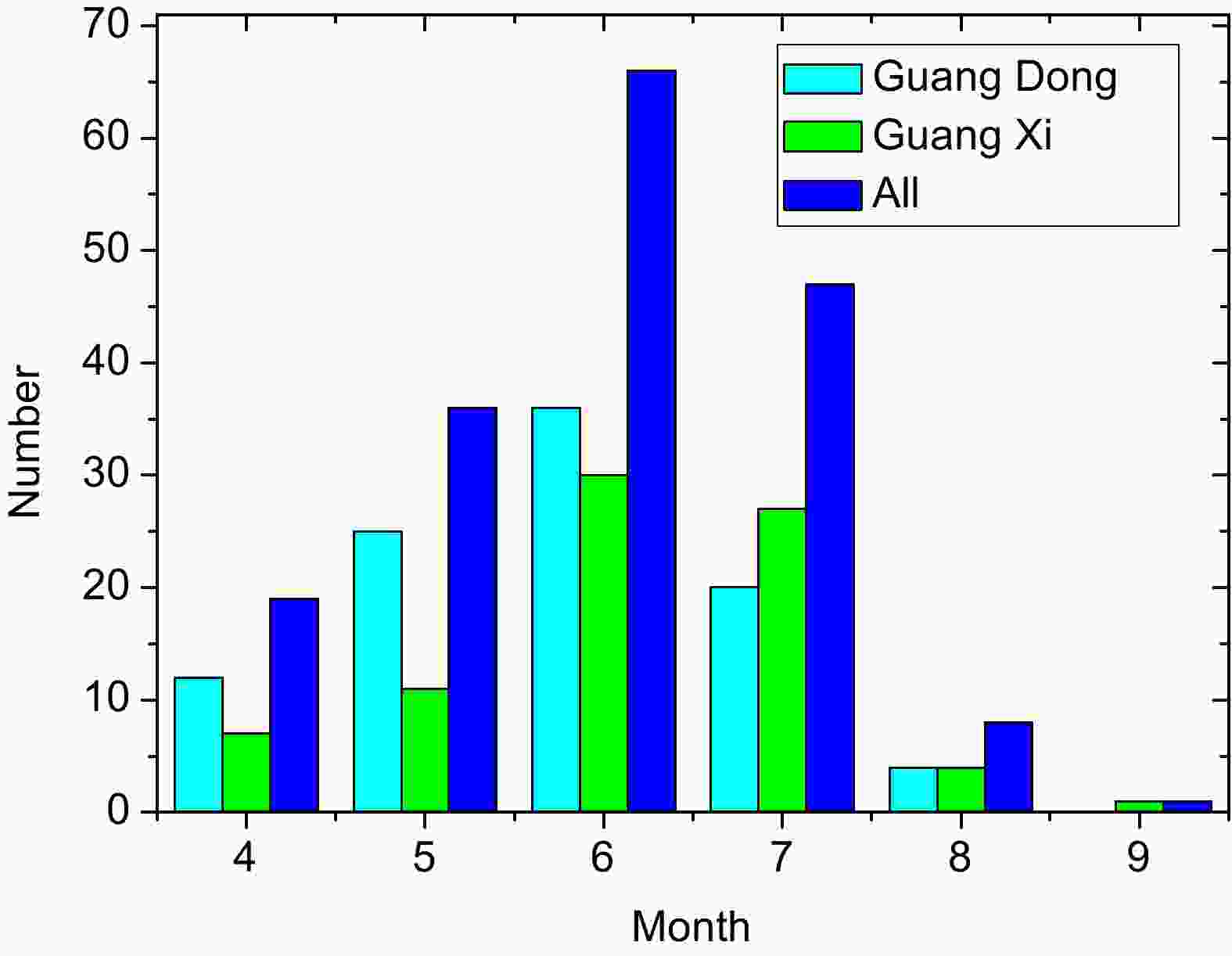
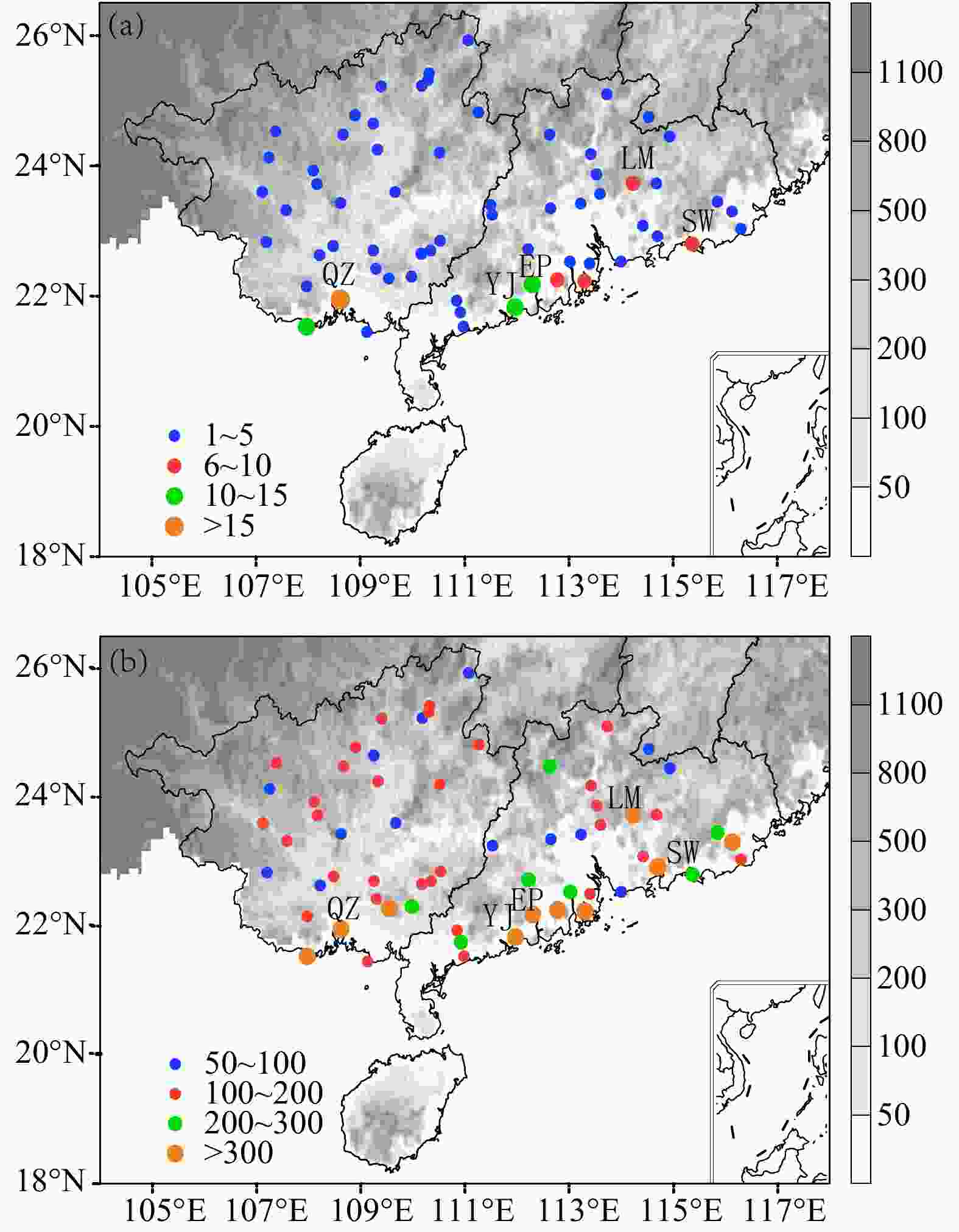
2
2.2. Synoptic weather patterns
For operational forecasting in China, the forecaster often determines an occurrence of WSHR according to the synoptic weather situation, as most WSHR cannot be predicted successfully by numerical models. Therefore, many studies have focused on the weather systems and environmental conditions that produce WSHR in South China (Huang et al., 1986; Lin et al, 2006; Miao et al., 2018; Zheng et al., 2018). Huang et al. (1986) summarized heavy rainfall in four types of weather systems: warm wind shear line, the LLJ along coastlines, the prefrontal LLJ, and cold fronts or quasi-stationary fronts. Forecasters in South China have categorized the synoptic weather systems influencing WSHR events into three types (Lin et al., 2006): backflow heavy rainfall caused by converging flows or warm and wet wind shear behind a transformed cold high pressure system; heavy rainfall caused by a strong southwestern monsoon surge or southwestern LLJ, and heavy rainfall produced by the upper-level trough and subtropical LLJ. The backflow WSHR was not included in the categories proposed by He et al. (2016). Based on previous research and numerous case studies, Liu et al. (2019) divided the synoptic patterns of heavy rainfall into four types: wind shear, low vortex, southern wind, and backflow. The wind shear and low vortex types of heavy rainfall were mainly concentrated in June and July (Fig. 3), while the frequency of southern wind type heavy rainfall was similar from April to July and the backflow type occurred in April to the middle of May (Fig. 3). It was also found that different types of WSHR could influence the weather in different regions of South China. The low vortex type of heavy rainfall mainly occurred along the coastline of Guangxi Province, while the backflow heavy rainfall often occurred in the coastal regions of the Pearl River Delta in Guangdong Province during the spring.
Under the control of the above-mentioned weather systems, synoptic circulations are favorable for the occurrence of convective systems, such as large amounts of convective available potential energy (CAPE, greater than 800 J kg?1), abundant water vapor, and thick convective unstable layers. Among the key environmental parameters of the four types of WSHR, CAPE in the low vortex type is the strongest, but is the weakest in the backflow type (Liu, 2018). It is possible that all the backflow type events occurred in spring, with strong baroclinity, and the dynamic forcing played a more important role than thermal forcing. However, for the 0?3 km vertical wind shear, the warm wind shear line type was the strongest.
2
2.3. MCSs and trigger mechanisms for WSHR
Generally, WSHR is produced by MCSs related to certain weather systems and environmental conditions. The triggering of WSHR events in South China shows different features in different situations (Zhao et al., 2007; Luo et al., 2017; Du and Chen, 2018). Since the relevant triggering mechanism locally is far from the obvious synoptic forcing and/or cold air, rainfall forecasting has been a challenge and has attracted wide attention (Chen et al., 2014, 2015; Wang et al., 2014; Wu and Luo, 2016; Du and Chen, 2018). The triggering mechanisms are the key scientific uncertainty associated with MCSs. Generally, there are two kinds of triggering mechanism: (1) the inhomogeneous underlying surface layer, such as the wave or lifting effect triggered by topography and/or land?sea contrast (Xu et al., 2012; Chen et al., 2014, 2015, 2016a), and (2) the atmospheric perturbation instability (Wilson and Schreiber, 1986; Weckwerth and Wakimoto, 1992; Ding and Shen, 1998; Moncrieff and Liu, 1999), density current (Houston, 2017), and the gravity wave (Liu et al., 2012; Xu et al., 2013).The terrain in South China is high in the north and low in the south, and features various mountain ranges, hills, and a convoluted coastline. The terrain blocking, lifting, and abundance of water vapor near the oceans complicates the triggering mechanism of WSHR events in South China. The specific mechanisms are still unclear, and it is difficult for NWP models to forecast these heavy rainfall events. Huang et al. (1986) proved that WSHR generally occurs under a southerly wind in the low troposphere. When the mountain direction is perpendicular to the low-level southerly wind, the lifted warm and wet air can cause severe convective storms or strengthen the precipitation. According to the statistical results, the WSHR in South China occurred more frequently in coastal areas than in inland areas (Liu et al., 2019). Some studies have indicated that the topography and land?sea contrast affected the triggering mechanism associated with WSHR near the coastal region (Chen et al., 2014, 2015; Du and Chen, 2018). An observational analysis of surface station, satellite, and radar datasets demonstrated that the diurnal variation of the rainfall in the eastern coastal areas is very significant (Yu et al., 2007; Chen et al., 2009, 2013a, 2014, 2015; Jiang et al., 2017; Zheng et al., 2019), and the diurnal variation of deep convection and rainfall is related to the circulation of land?sea breezes (Zheng and Chen, 2013; Chen et al., 2015, 2016a). The interaction between convergence of land?sea breezes and topography near coastal areas enhances the intensity of precipitation (Chen et al., 2016a). Additionally, a relatively high topography and the trumpet-shaped topography in inland South China could also induce WSHR and enhance the total precipitation (Sun and Zhao, 2002a, b; Xia et al., 2006, Xia and Zhao, 2009; Tu et al., 2017; Liu et al., 2019). These previous studies suggest that topography and land?sea contrast are important factors impacting the intensity and distribution of WHSR events in South China.
Besides the influences of topography and land?sea contrast, the MCSs that produce WSHR events are mostly associated with the mesoscale convergence line and the LLJ, and other disturbances in the boundary layer (Lin et al., 2006). It has been suggested that warm-sector convection could be triggered by the low-level convergence associated with boundary layer processes and thin layers of cold air intruding into the boundary layer in South China (Sun and Zhao, 2002a, b; Xia et al., 2006). Heavy rainfall in South China is often accompanied by an LLJ or boundary layer jet (BLJ) (Chen and Yu, 1988; Chen et al., 2005, 2006; Tu et al., 2014). An LLJ or BLJ was also found to be significant in the generation of heavy rainfall (Tao and Chen, 1987; Zhang et al., 2000; Du and Chen, 2018, 2019; Du and Rotunno, 2018; Xue et al., 2018). Du and Chen (2018, 2019) found that the WSHR is associated with the southerly BLJ occurring over the northern region of the South China Sea. Moreover, the synoptic system-related LLJ and a BLJ, and their coupling, have key effects on triggering mechanisms, while the BLJ colliding with terrain may enhance coastal convergence for amplifying triggering mechanisms (Du and Chen, 2018, 2019). Moreover, the cold pool, the mesoscale outflow boundaries or cold dome, due to the precipitation, has been shown to have a strong effect on triggering convection (Wang et al., 2014; Wu and Luo, 2016; Tu et al., 2017; Liu et al., 2018). Especially, the mesoscale outflow boundaries over South China present weak surface out?ows with little movement, which is crucial to the generation of long-lasting extreme rainfall events (Liu et al., 2018). Some observational analyses and numerical simulations have been conducted on these MCSs, and the three-dimensional structure of convective systems and their evolution has been revealed (Zhang et al., 2000; Sun and Zhao, 2002a, b; Xia et al., 2006; Zhao et al., 2007). As WSHR events are usually not directly affected by synoptic forcings and/or cold air, accurate prediction in numeral models is a big challenge (Chen and Xu, 2011; Zhang et al., 2016; Huang and Luo, 2017; Wu et al., 2018a). The predictability of MCSs is also an important scientific topic within the field of mesoscale meteorology, with NWP models used to investigate the predictability of MCSs (Anthes et al., 1985; Cintineo and Stensrud, 2013). Chen and Xu (2011) found that the forecasting error and dispersion of WSHR events in South China was larger than that for heavy rainfall in the Yangtze River?Huaihe Valley, which led to large uncertainties in simulation results. In terms of error growth and ensemble forecasts, the numerical predictability of heavy rainfall in South China is lower than this in the Yangtze River?Huaihe Valley. The errors associated with water vapor transport and the humidity of the initial field have large impacts on the predictability of convective systems and its rainfall. Liu et al. (2005) indicated that the heating and water vapor transport from the convective systems in the South China Sea could influence synoptic circulation and the evolution of convection in South China. Many studies have proved that the humidity error is the largest uncertainty in the initial conditions and has a large impact on convection over South China (Wu et al., 2013, 2018a; Zhang et al., 2016; Lu et al., 2018). Lu et al. (2018) indicated that convective systems in the South China Sea play an important role in the convection and precipitation over the coastal areas of South China. Zhang et al. (2016) found that the assimilation of wind profile data significantly improved the short-range prediction of WSHR in southern China in terms of both intensity and location because of the improved representation of wind and moisture at lower levels in the analyses. Bao et al. (2017) demonstrated that assimilating the radial velocity of operational weather radar near the South China coast in both deterministic and probabilistic experiments generally improves the prediction of heavy-rain-producing MCSs.
Some studies have focused on WSHR events over southern areas of China in addition to the South China region (Chen et al., 2016b; Jiang et al., 2017; Wu et al., 2018; Wang et al., 2018). Some heavy rainfall south of the Yangtze River has been observed in the warm sector, about 100?300 km from the surface cold front or warm wind shear line at 850 hPa, and at the edge of the western Pacific subtropical high (WPSH) (Chen et al., 2016b; Jiang et al., 2017). The WSHR events in the regions south of the Yangtze River and the middle and lower reaches of the Yangtze River have the synoptic patterns of the warm wind shear line, pre-cold front, and edge of the WPSH. It has also been reported that the severe precipitation maxima in these WSHR events are dispersed and occur on the mountains, borders of mountains and plains, and borders between lakes (Jiang et al., 2017). Torrential rainfall occurs frequently in the mountainous areas in western Hunan Province, and can cause extreme flooding events. The WSHR maxima over the middle and lower regions of the Yangtze River Valley occur in the Dabie Mountains and the hilly areas in southern Anhui Province. These rainfall events occur in an environment of low-level high humidity, inhomogeneous local heating differences on the underlying surfaces, and the accumulation of large unstable energy in the upper dry and lower wet air (Jiang et al., 2017). Although it is difficult to forecast WSHR events, according to the mesoscale analysis technology from the National Meteorological Center of the China Meteorological Administration (Zhang et al., 2012), some events could be successfully predicted.
WSHR events can also occur in North China, such as the extreme heavy rainfall on 21 July 2012 that caused many casualties and considerable economic losses. This extreme heavy rainfall included warm-sector and frontal precipitation. Due to the lack of understanding of WSHR, forecasts of their occurrence are inaccurate and the forecast intensity is typically less than the actual precipitation record (Chen et al., 2012b, 2013b). After the rainfall event on 21 July 2012, multi-scale weather systems, convections, and predictability were studied (Sun et al., 2013; Zhang et al., 2013; Meng et al., 2019), but the triggering mechanisms of the convection required for WSHR ahead of cold fronts in North China are still not fully understood. Some WSHR events in North China have been studied, and it has been suggested that the conditional symmetric instability might be responsible for the formation of parallel-type warm sector rain bands (Xu and Wang, 1989; Wang et al., 1990). However, there is still uncertainty regarding the temporal and spatial characteristics, triggering, and maintenance mechanisms of WSHR events in North China.
(1) The environmental conditions, topography, land?sea contrast, BLJ and SLJ could impact the triggering and development of WSHR events. A WSHR can be affected by more than two of these factors. The interaction of these factors is very complicated. For example, the land?sea breezes that occur due to the heating differences in the coastal areas of South China, together with the trumpet-shaped and hilly topography of the Pearl River Delta, play important roles in the intensity of heavy rainfall events. Additionally, large urban agglomerations could influence the local thermal circulations. Because of the complex mechanisms of the WSHR in South China, the specific effects of the above-mentioned factors and their impacts under different synoptic background circulations need to be investigated and explored. Thus, the mechanisms of triggering, maintenance, and organization of the convections in WSHR can be better understood and used to improve forecasts.
(2) Most studies of WSHR in China have focused on South China. To better understand the concentrated summer precipitation in the Yangtze River?Huaihe Valley and North China, with its complicated terrain, a high spatial and temporal resolution dataset should be collected and used to analyze the distribution and environmental conditions of WSHR events. This would enable the differences and similarities of the triggering and maintenance mechanisms of WSHR events to be evaluated in different regions.
(3) Current NWP models have a limited ability to predict WSHR events. To improve the prediction of WSHR events, there is a need to better understand their mechanisms based on high spatial and temporal resolution observations. There is also a need to improve the initial conditions and physical processes of numerical models, especially the description of the triggering process and the microphysical parameterizations.
Acknowledgments. This research was supported by the National Natural Science Foundation of China (Grant No. 41675045), National Key R&D Program of China (Grant No. 2018YFC1507200), and the Jiangxi Key Basic Research and Development Project of China (Grant No. 20171BBG70005).