贾羽1,丁瑜1,2,王晅1,3,张家生1,3,闫宏业4,张宇1,陈晓斌1,3
(1.中南大学 土木工程学院,长沙 410083;2.江苏海洋大学 土木与港海工程学院,江苏 连云港 222005; 3.中南大学 高速铁路建造技术国家工程研究中心,长沙 410083;4.中国铁道科学研究院集团有限公司,北京 100081)
摘要:
列车荷载作用下路基翻浆冒泥的实质是路基土体细颗粒迁移,细颗粒迁移会改变路基上覆碎石层孔隙结构,影响上覆碎石层力学及水力学性质。为此,采用自主研发的路基翻浆冒泥试验模型进行试验,结合X射线计算机断层(computed tomography,CT)扫描技术,分析循环荷载作用下路基土体细颗粒迁移对上覆碎石层细观孔隙结构的影响。结果表明:循环荷载作用下,碎石层孔隙的压缩作用及路基土体细颗粒迁移至碎石层的堵塞作用,不仅改变碎石层面孔隙率的分布特性,还减小碎石层孔隙率及孔隙连通度;孔隙尺寸分布特性不受加载影响,加载前后,碎石孔隙均呈现小孔隙多、大孔隙少的特点,孔隙分布特征曲线满足对数正态分布,但随着循环荷载的施加,碎石层内孔隙总数量减小;孔隙的形状参数(细长比和扁平度)均呈正态分布,细长比随循环荷载作用次数无明显变化规律,而加载后碎石层孔隙扁平度的分布更加均匀;碎石层孔隙具有明显的分形特性,加载前孔隙三维分形维数为2.40~2.50,随着循环荷载的施加,孔隙三维分形维数逐渐减小。
关键词: CT扫描技术 循环荷载 细颗粒迁移 细观孔隙结构
DOI:10.11918/202212084
分类号:TU41
文献标识码:A
基金项目:国家自然科学基金(51978674);高原高寒铁路典型结构状态检监测与评估关键技术研究(2021YJ341);中国国家铁路集团有限公司科技研究开发计划(L2022G002);湖南省自然科学基金(2022JJ30737)
Evolution analysis of microscopic pore structure in gravel layer under cyclic loading
JIA Yu1,DING Yu1,2,WANG Xuan1,3,ZHANG Jiasheng1,3,YAN Hongye4,ZHANG Yu1,CHEN Xiaobin1,3
(1.School of Civil Engineering, Central South University, Changsha 410083, China; 2.School of Civil and Ocean Engineering, Jiangsu Ocean University, Lianyungang 222005, Jiangsu, China; 3.National Engineering Research Center of High-Speed Railway Construction Technology, Central South University, Changsha 410083, China; 4.China Academy of Railway Sciences Corporation Limited, Beijing 100081, China)
Abstract:
The essence of subgrade mud pumping under train load is the migration of fine particles in subgrade soil. The migration of fine particles will change the pore structure of overlying gravel, thus affect the mechanical and hydraulic properties of gravel layer. To study the influence of fine particle migration from subgrade soil on the microscopic pore structure of gravel layer under cyclic loading, a series of tests are carried out by a self-developed subgrade mud pumping test model, combined with X-ray computed tomography (CT) scanning technology. It has been shown that under cyclic loading, compression of the gravel pores and clogging of migrated fine particles not only change the distribution characteristics of gravel plane porosity, but also decrease the porosity and porosity connectivity. Moreover, the characteristics of the pore size distribution are not affected by loading. The pores of the gravel layer show features of more small pores and fewer large pores, and the characteristic curves of the pore distribution satisfy log-normal distribution before and after loading. However, with the application of cyclic load, the total number of pores decreases. The distribution of pore shape parameters (slenderness ratio and flatness) shows a normal distribution characteristic. The slenderness ratio has not changed significantly with the number of cycles, while the distribution of flatness is more uniform after loading. The pores of the gravel layer have a distinct fractal character. In addition, the three-dimensional fractal dimension of the pores is distributed between 2.40 and 2.50 before loading. With the application of cyclic loading, the three-dimensional fractal dimension gradually decreases.
Key words: CT scanning technology cyclic loading fine particle migration microscopic pore structure
贾羽, 丁瑜, 王晅, 张家生, 闫宏业, 张宇, 陈晓斌. 循环加载下碎石层细观孔隙结构演化分析[J]. 哈尔滨工业大学学报, 2024, 56(6): 116-123. DOI: 10.11918/202212084.

JIA Yu, DING Yu, WANG Xuan, ZHANG Jiasheng, YAN Hongye, ZHANG Yu, CHEN Xiaobin. Evolution analysis of microscopic pore structure in gravel layer under cyclic loading[J]. Journal of Harbin Institute of Technology, 2024, 56(6): 116-123. DOI: 10.11918/202212084.

基金项目 国家自然科学基金(51978674);高原高寒铁路典型结构状态检监测与评估关键技术研究(2021YJ341);中国国家铁路集团有限公司科技研究开发计划(L2022G002);湖南省自然科学基金(2022JJ30737) 作者简介 贾羽(1990—),男,博士研究生;
张家生(1964—),男,博士生导师 通信作者 丁瑜,ding_yu0212@163.com 文章历史 收稿日期: 2022-12-22
Abstract Full text Figures/Tables PDF
循环加载下碎石层细观孔隙结构演化分析
贾羽1, 丁瑜1,2


1. 中南大学 土木工程学院,长沙 410083;
2. 江苏海洋大学 土木与港海工程学院,江苏 连云港 222005;
3. 中南大学 高速铁路建造技术国家工程研究中心,长沙 410083;
4. 中国铁道科学研究院集团有限公司,北京 100081
收稿日期: 2022-12-22; 录用日期: 2023-03-17; 网络首发日期: 2023-05-29
基金项目: 国家自然科学基金(51978674);高原高寒铁路典型结构状态检监测与评估关键技术研究(2021YJ341);中国国家铁路集团有限公司科技研究开发计划(L2022G002);湖南省自然科学基金(2022JJ30737)
作者简介: 贾羽(1990—),男,博士研究生; 张家生(1964—),男,博士生导师
通信作者: 丁瑜,ding_yu0212@163.com
摘要: 列车荷载作用下路基翻浆冒泥的实质是路基土体细颗粒迁移,细颗粒迁移会改变路基上覆碎石层孔隙结构,影响上覆碎石层力学及水力学性质。为此,采用自主研发的路基翻浆冒泥试验模型进行试验,结合X射线计算机断层(computed tomography, CT)扫描技术,分析循环荷载作用下路基土体细颗粒迁移对上覆碎石层细观孔隙结构的影响。结果表明:循环荷载作用下,碎石层孔隙的压缩作用及路基土体细颗粒迁移至碎石层的堵塞作用,不仅改变碎石层面孔隙率的分布特性,还减小碎石层孔隙率及孔隙连通度;孔隙尺寸分布特性不受加载影响,加载前后,碎石孔隙均呈现小孔隙多、大孔隙少的特点,孔隙分布特征曲线满足对数正态分布,但随着循环荷载的施加,碎石层内孔隙总数量减小;孔隙的形状参数(细长比和扁平度)均呈正态分布,细长比随循环荷载作用次数无明显变化规律,而加载后碎石层孔隙扁平度的分布更加均匀;碎石层孔隙具有明显的分形特性,加载前孔隙三维分形维数为2.40~2.50,随着循环荷载的施加,孔隙三维分形维数逐渐减小。
关键词: CT扫描技术 循环荷载 细颗粒迁移 细观孔隙结构
Evolution analysis of microscopic pore structure in gravel layer under cyclic loading
JIA Yu1, DING Yu1,2


1. School of Civil Engineering, Central South University, Changsha 410083, China;
2. School of Civil and Ocean Engineering, Jiangsu Ocean University, Lianyungang 222005, Jiangsu, China;
3. National Engineering Research Center of High-Speed Railway Construction Technology, Central South University, Changsha 410083, China;
4. China Academy of Railway Sciences Corporation Limited, Beijing 100081, China
Abstract: The essence of subgrade mud pumping under train load is the migration of fine particles in subgrade soil. The migration of fine particles will change the pore structure of overlying gravel, thus affect the mechanical and hydraulic properties of gravel layer. To study the influence of fine particle migration from subgrade soil on the microscopic pore structure of gravel layer under cyclic loading, a series of tests are carried out by a self-developed subgrade mud pumping test model, combined with X-ray computed tomography (CT) scanning technology. It has been shown that under cyclic loading, compression of the gravel pores and clogging of migrated fine particles not only change the distribution characteristics of gravel plane porosity, but also decrease the porosity and porosity connectivity. Moreover, the characteristics of the pore size distribution are not affected by loading. The pores of the gravel layer show features of more small pores and fewer large pores, and the characteristic curves of the pore distribution satisfy log-normal distribution before and after loading. However, with the application of cyclic load, the total number of pores decreases. The distribution of pore shape parameters (slenderness ratio and flatness) shows a normal distribution characteristic. The slenderness ratio has not changed significantly with the number of cycles, while the distribution of flatness is more uniform after loading. The pores of the gravel layer have a distinct fractal character. In addition, the three-dimensional fractal dimension of the pores is distributed between 2.40 and 2.50 before loading. With the application of cyclic loading, the three-dimensional fractal dimension gradually decreases.
Keywords: CT scanning technology cyclic loading fine particle migration microscopic pore structure
随着中国经济的快速发展,各地区资源需求量日益增大,铁路运输流量增大、载重提升,各种路基病害尤其是翻浆冒泥病害日益严重。Duong等[1-2]通过试验发现, 列车荷载作用下饱和路基层间产生较大的超孔隙水压力,超孔隙水压力的增长导致有效应力的降低;道砟在外荷载的作用下渗透进入路基层,并将路基土体颗粒挤压进入道砟层孔隙中。Kermani等[3]的研究表明,循环荷载作用下路基内产生较高的超孔隙水压力,超孔隙水压力的消散带动路基土体细颗粒迁移,最终导致了翻浆冒泥现象。列车荷载作用下路基翻浆冒泥的实质是路基土体细颗粒迁移的过程。细颗粒迁移会改变路基上覆碎石的孔隙结构,从而影响上覆碎石层力学及水力学性质[4-5]。研究循环荷载作用下细颗粒迁移对上覆碎石层细观孔隙结构的影响具有重要的理论意义和工程价值。
土体细观孔隙结构常用观测手段有扫描电镜法[6]、压汞仪法[7]、核磁共振[8]以及电子计算机断层(computed tomography, CT)扫描技术[9]等。CT扫描技术具有不损伤土体结构的优点,被广泛应用于土体孔隙结构观测的研究中。Hasan等[10]利用CT扫描技术研究了砂土在剪切过程中剪切带的形成及剪切带内外孔隙比、颗粒接触数量的变化。Jiang等[11]采用中型三轴压缩设备结合CT扫描技术,分析了碎石在三轴压缩过程中的体变行为及颗粒的细观运动特性。Wang等[12]采用CT扫描技术和离散元软件,分析了砂砾土在液化过程中颗粒方位变化、颗粒间力链变化,提出了砂砾土液化的微观机制。熊剑平等[13]对不同加载阶段多孔弹性混合料进行CT扫描,发现混合料试样高度在70 mm以下部分孔隙率随着荷载的施加呈增大的趋势,在70 mm以上部分孔隙结构对荷载的影响不敏感。张家发等[14-15]对不同颗粒形状粗粒土试样进行了CT扫描,从细观角度研究了颗粒形状对粗粒土孔隙特征和渗流特性的影响。
目前,已有很多学者对碎石土孔隙结构特征进行了研究,但是针对循环荷载作用下路基土体细颗粒迁移对上覆碎石层孔隙结构影响的研究几乎是空白的。本文利用自主研发的翻浆冒泥试验模型结合CT扫描技术,研究了循环荷载作用下路基土体细颗粒迁移对上覆碎石层孔隙结构的影响。
1 试验 1.1 试验材料为研究循环荷载作用下路基土体细颗粒迁移对上覆碎石层孔隙结构的影响,选用了易发生翻浆冒泥的粉质黏土作为路基土体[16-18]。该粉质黏土颗粒级配曲线如图 1所示,其基本物理力学性质如表 1所示。
Fig. 1

表 1
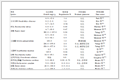
粉质黏土 2.64 33.42 16.64 16.78 1.81 14.7
注:Gs为土粒相对密度, wL为液限,wP为塑限,IP为塑性指数, ρd max为最大干密度;wopt为最优含水率。
表 1 粉质黏土的基本物理力学参数 Tab. 1 Physical and mechanical parameters of silty clay
上覆碎石层采用轧制砂岩,该碎石的颗粒相对密度为2.69,最大干密度为2.08 g/cm3。原状碎石颗粒级配曲线如图 1所示,试验过程中考虑试验设备尺寸的限制,采用类比修正法对原状碎石的颗粒级配进行缩尺处理,缩尺碎石的级配曲线见图 1。
1.2 试验方法 1.2.1 试验模型翻浆冒泥试验采用自主研发的试验模型,主要由轴向加载器、可视化试样筒、试样饱和器以及数据采集仪组成,如图 2(a)所示。该模型可以结合CT扫描设备观测细颗粒迁移过程中道砟孔隙结构的演化,关于试验模型更多介绍见文献[19]。
Fig. 2
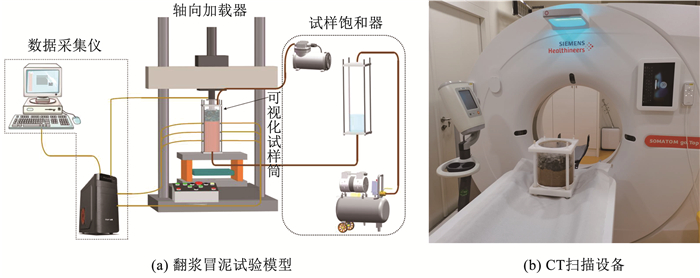
CT扫描试验采用SIEMENS医用CT扫描仪,如图 2(b)所示。该扫描仪可检测试样的最大尺寸为700 mm×400 mm(直径×高度),空间分辨率为700 μm,能够满足试验所需精度。
1.2.2 试验方案在文献[19]的基础上对试样筒进行了改装以适用CT扫描设备的扫描尺寸要求。试样为圆柱形,直径为178 mm,总高度为180 mm。试样下部为粉质黏土,高度为80 mm。粉质黏土制样时采用分层压实法,每一层厚度为40 mm,初始干密度分别为1.40、1.50、1.59 g/cm3,含水率为最优含水率。粉质黏土上部直接铺设碎石层,高度为100 mm,采用分层夯实法制样,每一层厚度为50 mm。
试样制备完成后(试样见图 2(b)),对下部粉质黏土进行饱和。首先,对试样进行抽真空处理,随后打开进水阀门使水从试样下部缓慢流入试样内,待水面高出粉质黏土表面约10 mm时,关闭阀门。将整个试样静置一段时间后,放入CT扫描设备中进行第一次扫描。
对扫描完的试样进行循环加载,采用图 3所示正弦波对列车荷载进行模拟。依据Bian等[20]提出的道砟层最大动应力幅值计算方法,得出了轴重为27 t、运行速度为80 km/h的列车在道砟层产生的最大动应力幅值约为240 kPa,因此,选用的荷载幅值分别为50、100、200 kPa。试样加载前,预先施加40 kPa轴向静应力以模拟轨道结构重力影响。循环荷载频率与列车运行速度和列车轴距等因素有关,列车运行速度在0~100 km/h时对应的荷载频率为0~14 Hz[21],本文选取的加载频率为5 Hz。待试样加载至1 000、15 000、30 000、50 000次时,停止荷载的施加,并将试样缓慢取出进行第2、3、4次及最后一次CT扫描。具体试验方案如表 2所示。
Fig. 3
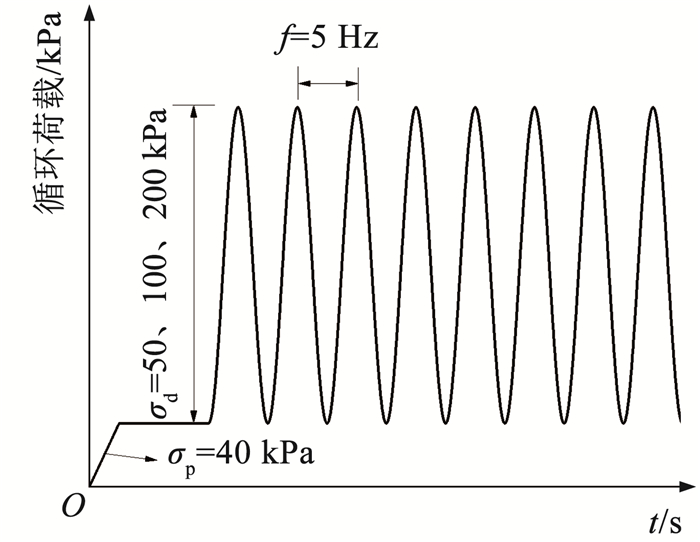
表 2
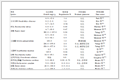
S-1.40-50-5 1.40 50 5 试样饱和后进行一次CT扫描,加载至1 000、15 000、30 000、50 000次时,停止加载,静置后进行CT扫描
S-1.40-100-5 1.40 100 5
S-1.40-200-5 1.40 200 5
S-1.50-100-5 1.50 100 5
S-1.59-100-5 1.59 100 5
表 2 试验方案 Tab. 2 Test program
2 结果分析与讨论 2.1 循环加载过程中试样CT图像图 4为S-1.40-100-5试样在循环加载过程中细颗粒迁移程度,图 5为S-1.40-100-5试样在循环加载过程中的CT扫描图像(纵剖面图)。图 4和图 5表明:试样饱和完成后,道砟层和路基土体的界面清晰;循环荷载施加后,上部道砟层下陷进入软弱的粉质黏土路基内,并将一部分粉质黏土泥浆挤入道砟层的孔隙中,形成夹层;随着循环荷载的继续施加,粉质黏土泥浆也随之继续向上翻冒,碎石层孔隙进一步减小。
Fig. 4
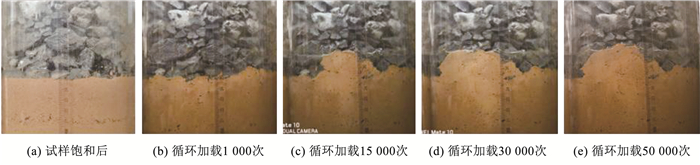
Fig. 5

2.2 碎石层孔隙率的演化图 6为S-1.40-100-5试样碎石层在不同加载状态下面孔隙率沿试样高度方向分布(由于篇幅限制,只绘制了一组试样面孔隙率分布图,其他试样的面孔隙率分布规律类似)。可以看出,试样在饱和之后,高度方向上碎石层面孔隙率分布在35%~55%,呈两端大、中间小的分布特征。随着循环荷载施加,碎石层孔隙被压缩,路基土体细颗粒迁移将碎石层下部孔隙堵塞,两者共同作用使碎石层面孔隙率呈减小的趋势,尤其是碎石层下端面孔隙率几乎接近0,碎石层面孔隙率整体呈现上端大、中间小、下端极其小的分布特征。
Fig. 6
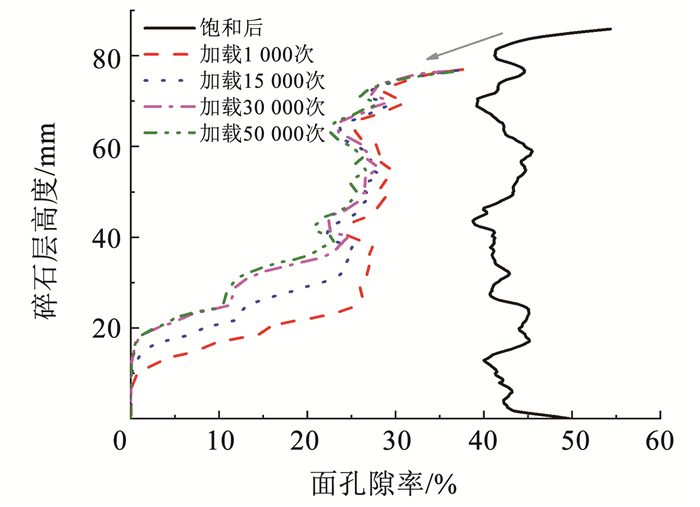
表 3为不同加载阶段碎石层体孔隙率。可以看出,随着循环荷载的施加,碎石层体孔隙率逐渐减小。5组试样在加载前,碎石层体孔隙率在43%左右,加载50 000次后,碎石层的体孔隙率下降为12%~21%;相比加载前,试验结束后碎石层体孔隙率减小了50%~70%。
表 3
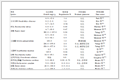
S-1.40-50-5 S-1.40-100-5 S-1.40-200-5 S-1.50-100-5 S-1.59-100-5
饱和后 42.32 42.66 43.45 40.07 43.76
加载1 000次 27.33 22.60 19.28 23.79 23.86
加载15 000次 22.76 19.21 14.04 20.84 21.90
加载30 000次 21.33 17.46 13.11 20.74 21.13
加载50 000次 19.64 16.28 12.26 20.18 21.02
表 3 碎石层在不同加载阶段的体孔隙率 Tab. 3 Volume porosity of gravel at different loading stages
2.3 碎石层孔隙尺寸分布特征的演化图 7为试样S-1.40-100-5在不同加载阶段的碎石层孔隙尺寸分布。可以看出,饱和后,碎石层孔隙等效直径分布在0~17.0 mm,其中,等效直径在>1.0~2.0 mm的孔隙数量占比最多,为32.14%;其次为>2.0~3.0 mm和0~1.0 mm的孔隙数量,分别占孔隙总数量的19.39%和13.78%。随着循环荷载的施加,孔隙被压缩或被迁移细颗粒堵塞,碎石层内大孔隙(如直径在16.0~17.0 mm和9.0~10.0 mm的孔隙)逐渐消失。另外,不同加载阶段试样碎石层孔隙等效尺寸分布服从式(1)所示的对数正态分布:
$f(x)=\frac{\alpha}{\sqrt{2 {\rm{ \mathsf{ π}}}} \sigma x} \exp \left[-\frac{\left(\ln x-\ln x_{\mathrm{c}}\right)^2}{2 \sigma^2}\right]$ (1)
Fig. 7
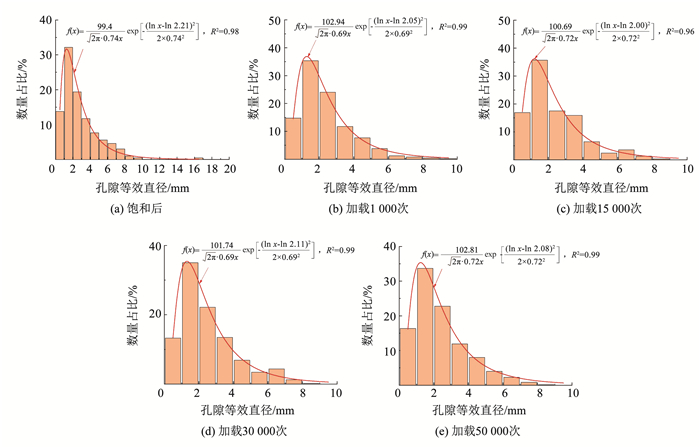
式中:x为孔隙半径,α为分布的相对比例,xc为平均孔隙半径,σ为标准差。不同阶段试样碎石层孔隙尺寸分布函数见图 7。
图 8为试样S-1.40-100-5碎石层内大(孔隙直径>5 mm)、中(孔隙直径3~5 mm)、小(孔隙直径<3 mm)孔隙占比及总孔隙数量随循环加载次数的变化。可以看出,不同加载阶段碎石层内孔隙均呈小孔隙多、大孔隙少的特征。随着循环荷载的施加,碎石层内小孔隙占比增大,大孔隙占比减小,中孔隙占比变化不明显;孔隙总数量呈减小的变化趋势。
Fig. 8

2.4 碎石层孔隙连通度的演化在计算孔隙连通度时,将碎石层划分为几个区域进行计算,取其平均值作为整个碎石层的孔隙连通度。表 4为5组试样在不同加载阶段碎石层孔隙连通度。加载前,碎石层孔隙连通度较高,均在80%以上;随着加载次数的增多,碎石层孔隙连通度逐渐下降。并且应力幅值越大,孔隙连通度下降得越显著,如试样S-1.40-50-5在加载前碎石层孔隙连通度为84.85%,加载50 000次后其孔隙连通度减少为55.89%;试样S-1.40-200-5在加载前碎石层孔隙连通度为80.51%,加载50 000次后其孔隙连通度减少为31.13%。类似地,粉质黏土的初始干密度越大,循环加载后孔隙连通度下降得越不明显,如试样S-1.40-100-5在加载前碎石层孔隙连通度为81.89%,加载50 000次后其孔隙连通度减少为41.58%;试样S-1.59-100-5在加载前碎石层孔隙连通度为85.09%,加载50 000次后其孔隙连通度减少为64.65%。
表 4
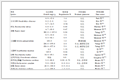
S-1.40-50-5 S-1.40-100-5 S-1.40-200-5 S-1.50-100-5 S-1.59-100-5
饱和后 84.85 81.89 80.51 82.30 85.09
加载1 000次 69.61 57.17 49.63 69.57 74.89
加载15 000次 61.72 51.21 36.61 62.50 67.70
加载30 000次 57.84 45.22 33.02 57.96 65.84
加载50 000次 55.89 41.58 31.13 54.02 64.65
表 4 试样在不同加载阶段的孔隙连通度 Tab. 4 Pore connectivity of samples at different loading stages
2.5 碎石层孔隙形状参数循环荷载作用下碎石层孔隙的压缩及细颗粒迁移对孔隙的堵塞作用使得碎石层孔隙形状发生改变,进而影响其水力学性质。孔隙三维形状可以采用如式(2)和(3)所示的细长比IE和扁平度IF表征[22]:
$I_{\mathrm{E}}=\frac{I}{L}$ (2)
$I_{\mathrm{F}}=\frac{S}{L}$ (3)
式中:L为孔隙最长轴的长度,I为垂直于L方向的次长轴的长度,S为同时垂直于L与I方向的最短轴长度。细长比和扁平度越接近于1.0,表明孔隙形状越接近于正方体或球体。
图 9为S-1.40-100-5试样碎石层孔隙细长比频率分布。可以看出: 碎石层内孔隙细长比主要分布在0.4~0.7,并且细长比分布满足正态分布特征;孔隙细长比随循环加载次数并无明显的变化规律。
Fig. 9
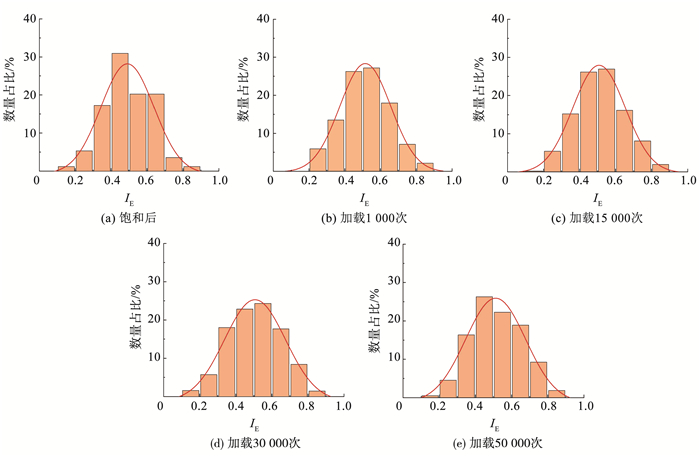
图 10为S-1.40-100-5试样碎石层孔隙扁平度频率分布。可以看出,碎石层内孔隙的扁平度主要分布在0.3~0.6,孔隙扁平度的分布也满足正态分布特征。随着循环荷载的施加,扁平度在>0.4~0.5的孔隙占比明显减少,扁平度在0.3~0.4的孔隙占比增多;其分布形式仍满足正态分布特征。相比未加载的试样,试验结束后,试样碎石层内孔隙扁平度分布更为均匀。
Fig. 10
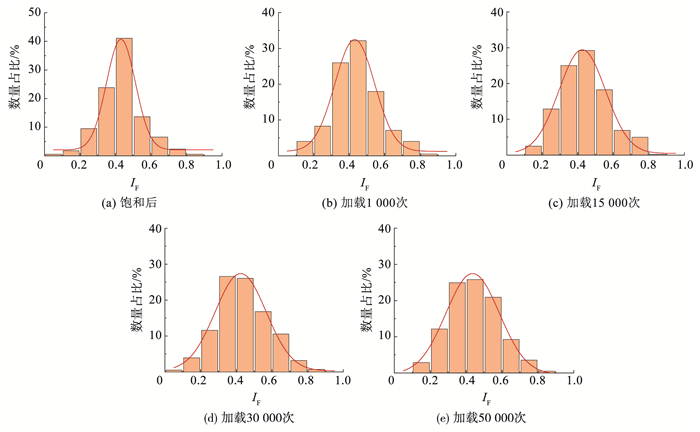
2.6 孔隙的分形特性分形是评价物体自相似程度和复杂程度的概念,可以采用分形维数来定量描述。采用计盒维数法对碎石层孔隙三维分形维数D进行计算,分形维数D定义为
$D=\lim\limits_{\varepsilon \rightarrow 0} \frac{\ln N(\varepsilon)}{\ln \varepsilon^{-1}}$ (4)
式中:ε为测量单元的尺寸,对于三维图形ε等价于球体或正方体; N(ε)为测量单元尺寸下得到的非空单元数量。通常,多孔介质三维分形维数为2~3,数值越大,表明孔隙结构的复杂程度越高。
表 5为5组试样在不同加载状态下的孔隙三维分形维数。试样饱和后,碎石层孔隙三维分形维数为2.40~2.50,随着循环荷载的施加,孔隙三维分形维数呈非线性减小。这说明循环荷载作用下碎石层孔隙结构的复杂程度降低。对比S-1.40-50-5、S-1.40-100-5及S-1.40-200-5 3组试样可知,循环应力幅值对碎石孔隙三维分形维数的变化有显著影响,应力幅值越大,试验结束后孔隙三维分形维数减小的程度越大。对比S-1.40-100-5、S-1.50-100-5及S-1.59-100-5 3组试样可知,粉质黏土初始干密度对上部碎石层孔隙三维分形维数的影响相对较小。
表 5
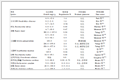
S-1.40-50-5 S-1.40-100-5 S-1.40-200-5 S-1.50-100-5 S-1.59-100-5
饱和后 2.46 2.49 2.44 2.45 2.42
加载1 000次 2.41 2.41 2.33 2.41 2.40
加载15 000次 2.39 2.40 2.28 2.44 2.39
加载30 000次 2.37 2.39 2.26 2.39 2.39
加载50 000次 2.37 2.38 2.23 2.40 2.38
表 5 试样在不同加载阶段的三维分形维数 Tab. 5 Three-dimensional fractal dimension of samples at different loading stages
3 结论1) 循环荷载的作用改变了碎石层面孔隙率沿高度方向的分布特征,使碎石层体孔隙率减小了50%~70%。加载前,碎石层孔隙连通度较高,在80%以上;随着循环荷载的施加,碎石层内孔隙连通度逐渐下降,并且应力幅值越大、粉质黏土初始干密度越小,碎石层内孔隙连通度下降得越明显。
2) 加载前后,碎石层内孔隙均呈小孔隙多、大孔隙少的特征,并且孔隙尺寸分布曲线服从对数正态分布;循环荷载作用使得碎石层内总孔隙的数量减少。另外,碎石层孔隙细长比对加载次数反应不敏感,加载前后孔隙细长比主要分布在0.4~0.7;而随着加载次数的增加,孔隙扁平度的分布更加均匀。
3) 加载前,试样碎石层孔隙三维分形维数为2.40~2.50,随着循环荷载的施加,孔隙三维分形维数逐渐减小,孔隙结构的复杂程度逐渐降低。并且,循环应力幅值对孔隙三维分形维数变化有显著的影响,而粉质黏土初始干密度对分形维数变化的影响不明显。
参考文献
[1] DUONG T V, CUI Yujun, TANG A M, et al. Physical model for studying the migration of fine particles in the railway substructure[J]. Geotechnical Testing Journal, 2014, 37(5): 20130145. DOI:10.1520/gtj20130145
[2] DUONG T V, CUI Yujun, TANG A M, et al. Investigating the mud pumping and interlayer creation phenomena in railway sub-structure[J]. Engineering Geology, 2014, 171: 45. DOI:10.1016/j.enggeo.2013.12.016
[3] KERMANI B, XIAO Ming, STOFFELS S M, et al. Measuring the migration of subgrade fine particles intosubbase using scaled accelerated flexible pavement testing: a laboratory study[J]. Road Materials and Pavement Design, 2017, 20(1): 36. DOI:10.1080/14680629.2017.1374995
[4] TRANI L D O, INDRARATNA B. Assessment of subballast filtration under cyclic loading[J]. Journal of Geotechnical and Geoenvironmental Engineering, 2010, 136(11): 1519. DOI:10.1061/(ASCE)GT.1943-5606.0000384
[5] SINGH M, INDRARATNA B, NGUYEN T T. Experimental insights into the stiffness degradation of subgrade soils prone to mud pumping[J]. Transportation Geotechnics, 2021, 27: 100490. DOI:10.1016/j.trgeo.2020.100490
[6] NI Hongyang, LIU Jiangfeng, PU Hai, et al. Microscopic pore structure and improved permeability characterization of COx argillite based on SEM images[J]. International Journal of Geomechanics, 2021, 21(8): 04021144. DOI:10.1061/(ASCE)GM.1943-5622.0002069
[7] WANG Jiaqi, WANG Qing, KONG Yuanyuan, et al. Analysis of the pore structure characteristics of freeze-thawed saline soil with different salinities based on mercury intrusion porosimetry[J]. Environmental Earth Sciences, 2020, 79(7): 161. DOI:10.1007/s12665-020-08903-w
[8] TANG Liyun, LI Gang, LI Zhen, et al. Shear properties and pore structure characteristics of soil-rock mixture under freeze-thaw cycles[J]. Bulletin of Engineering Geology and the Environment, 2021, 80: 3233. DOI:10.1007/s10064-021-02118-4
[9] NI Hongyang, LIU Jiangfeng, HUANG Bingxiang, et al. Quantitative analysis of pore structure and permeability characteristics of sandstone using SEM and CT images[J]. Journal of Natural Gas Science and Engineering, 2021, 88(1): 103861. DOI:10.1016/j.jngse.2021.103861
[10] HASAN A, ALSHIBLI K A. Experimental assessment of 3D particle-to-particle interaction within sheared sand using synchrotron microtomography[J]. Géotechnique, 2010, 60(5): 369. DOI:10.1680/geot.2010.60.5.369
[11] JIANG Jingshan, LIN Cheng, CHENG Zhanlin, et al. Investigation of macroscopic and microscopic behavior of gravels using triaxial compression test with CT scan[J]. Journal of Testing and Evaluation, 2019, 47(6): 20180547. DOI:10.1520/JTE20180547
[12] WANG Yong, WANG Yanli. Liquefaction characteristics of gravelly soil under cyclic loading with constant strain amplitude by experimental and numerical investigations[J]. Soil Dynamics and Earthquake Engineering, 2017, 92: 388. DOI:10.1016/j.soildyn.2016.10.029
[13] 熊剑平, 廖公云, 祁孔庆, 等. 单轴循环加载下多孔弹性混合料孔隙结构演变[J]. 中南大学学报(自然科学版), 2021, 52(7): 2299.
XIONG Jianping, LIAO Gongyun, QI Kongqing, et al. Evolution of pore structure within porous elastic mixture under unilateral cyclic loading[J]. Journal of Central South University (Science and Technology), 2021, 52(7): 2299. DOI:10.11817/j.issn.1672-7207.2021.07.017
[14] 彭家奕, 张家发, 沈振中, 等. 颗粒形状对粗粒土孔隙特征和渗透性的影响[J]. 岩土力学, 2020, 312(2): 236.
PENG Jiayi, ZHANG Jiafa, SHEN Zhenzhong, et al. Effect of grain shape on pore characteristics and permeability of coarse-grained soil[J]. Rock and Soil Mechanics, 2020, 312(2): 236. DOI:10.16285/j.rsm.2019.0066
[15] 叶加兵, 张家发, 邹维列. 颗粒形状对碎石料孔隙特性影响研究[J]. 岩土力学, 2018, 39(12): 169.
YE Jiabing, ZHANG Jiafa, ZOU Weilie. Influences of grain shape on pore characteristics of filled breakstone aggregate[J]. Rock and Soil Mechanics, 2018, 39(12): 169. DOI:10.16285/j.rsm.2017.0689
[16] 冷伍明, 粟雨, 滕继东, 等. 易发生翻浆冒泥的细粒土物理状态指标分析与评判[J]. 铁道学报, 2018, 40(1): 116.
LENG Wuming, SU Yu, TENG Jidong, et al. Analysis and evaluation on physical characteristics of fine-grained soils prone tomud pumping[J]. Journal of the China Railway Society, 2018, 40(1): 116. DOI:10.3969/j.issn.1001-8360.2018.01.018
[17] 聂如松, 冷伍明, 粟雨, 等. 基床翻浆冒泥土的物理力学性质[J]. 西南交通大学学报, 2018, 53(2): 286.
NIE Rusong, LENG Wuming, SU Yu, et al. Physical and mechanical properties of mud pumping soils in railway subgrade bed[J]. Journal of Southwest Jiaotong University, 2018, 53(2): 286. DOI:10.3969/j.issn.0258-2724.2018.02.010
[18] SINGH M, INDRARATNA B, RUJIKIATKAMJORN C. Use of geosynthetics in mitigating the effects of mud pumping: a railway perspective[J]. Geotechnics for Transportation Infrastructure, 2019, 623. DOI:10.1007/978-981-13-6713-7_48
[19] 丁瑜, 贾羽, 王晅, 等. 颗粒级配及初始干密度对路基翻浆冒泥特性的影响[J]. 岩土力学, 2022, 43(9): 2539.
DING Yu, JIA Yu, WANG Xuan, et al. Influence of particle size distribution and initial dry density on the characteristics of subgrade mud pumping[J]. Rock and Soil Mechanics, 2022, 43(9): 2539. DOI:10.16285/j.rsm.2021.1971
[20] BIAN Xuecheng, JIANG Jianqun, JIN Wanfeng, et al. Cyclic and postcyclic triaxial testing of ballast and subballast[J]. Journal of Materials in Civil Engineering, 2016, 28(7): 04016032. DOI:10.1061/(ASCE)MT.1943-5533.0001523
[21] SUN Qideng, INDRARATNA B, NIMBALKAR S. Deformation and degradation mechanisms of railway ballast under high frequency cyclic loading[J]. Journal of Geotechnical and Geoenvironmental Engineering, 2016, 142(1): 4015056. DOI:10.1061/(ASCE)GT.1943-5606.0001375
[22] KRUMBEIN W C. Measurement and geological significance of shape and roundness of sedimentary particles[J]. Sepm Journal of Sedimentary Research, 1941, 11(2): 64.